FIG. 27.1 Normal oxyhemoglobin dissociation curve is indicated with the solid line. This curve may shift (indicated with broken lines) whenever pH, temperature, PCO2, or 2,3-DPG values are increased or decreased. 2,3-DPG, 2,3-Diphosphoglycerate; Pco2, partial pressure of carbon dioxide; Po2, partial pressure of oxygen; SO2, oxygen saturation.
Adequate oxygenation is a factor of not only adequate oxygen saturation and hemoglobin values but also of adequate oxygen delivery, which necessitates appropriate cardiac output (CO) and the ability of the tissues to effectively use oxygen. Tissue hypoxia results when oxygen demand exceeds oxygen supply. Pulse oximetry readings therefore should be assessed in conjunction with all other indices of oxygenation.
Dysfunctional hemoglobin, a variant of the hemoglobin molecule unable to transport oxygen, presents a similar problem. Despite the high SpO2 level, hemoglobin may be insufficient to carry oxygen. Carboxyhemoglobin is hemoglobin that is bound with carbon monoxide and therefore is unavailable for carrying oxygen. Its effect must be considered in patients with burns or in tobacco smokers with carbon monoxide poisoning. In methemoglobinemia, the iron molecule on the hemoglobin is oxidized from the ferrous to the ferric state. This form of iron is unable to transport oxygen. Methemoglobinemia, although rare, can occur in patients who receive nitrate-based and other drugs and in those who are exposed to a variety of toxins. When dysfunctional hemoglobins are suspected, assessment of oxygenation with pulse oximetry must be supplemented with arterial blood gas saturations measured with a laboratory cooximeter to determine whether dyshemoglobins are present and oxygenation is adequate.
Perfusion at the sensor application site must be sufficient for the pulse oximeter to detect pulsatile flow, which is an important consideration for some patients in the PACU such as those treated with vasoconstrictors, those with marked hypothermia, and those with significantly reduced CO. A well-perfused site should be selected for application of the sensor. If in doubt, the pulse and adjacent capillary refill can be checked.
If the monitor cannot track the pulse, the patient first is evaluated for adverse physiologic changes. Next, the perianesthesia nurse should ensure that blood flow is not restricted such as by a flexed extremity, a blood pressure cuff, an arterial line, any restraints, or a sensor applied too tightly. Local perfusion to the sensor site can be improved by covering the site with a warm towel or with the use of a forced air warming device. Certain sensors, such as nasal sensors, are designed for application to areas where perfusion is preserved even when peripheral perfusion is relatively poor. Finally, some pulse oximeters use an electrocardiographic signal as an aid in identification of the pulse, thus enhancing the instrument’s ability to detect a weak pulse.
Patient movement can produce false signals that interfere with the ability of the pulse oximeters to identify the true pulse, thus leading to unreliable SpO2 and pulse rate readings. The sensor should be properly and securely applied; a sensor that is loosely attached or incorrectly positioned can magnify the effect of motion. If the problem persists, consideration should be given to moving the sensor to a less active site. Pulse oximeters that use the electrocardiographic signal as an aid in identification of the pulse can have an enhanced ability to distinguish between the true pulse and artifacts produced by motion. The result is more reliable SpO2 readings.
Normally, venous blood is nonpulsatile and is not detected with a pulse oximeter. In the presence of venous pulsations, the SpO2 value provided by the pulse oximeter may be a composite of both arterial and venous saturations. Venous pulsations can occur in patients with severe right-sided heart failure or other pathophysiologic states that create venous congestion and in patients receiving high levels of positive end-expiratory pressure. They can also occur when the sensor is placed distal to a blood pressure cuff or occlusive dressing and when additional tape is wrapped tightly around the sensor. When venous pulsations are present, the perianesthesia nurse should take care in interpreting the SpO2 readings and, if possible, attempt to eliminate the cause.
Because pulse oximeters are optical measuring devices, the perianesthesia nurse must be aware of additional factors that can influence the reliability of SpO2 readings. To ensure good light reception, the sensor’s light sources and detector must always be positioned according to the manufacturer’s specifications. In the presence of bright lights, such as infrared warming devices, fluorescent lights, direct sunlight, and surgical lights, the sensor must be covered with an opaque material to prevent incorrect SpO2 readings. In addition, agents that significantly change the optical-absorbing properties of blood, such as recently administered intravascular dyes, can interfere with reliable SpO2 measurements. The use of pulse oximetry with certain nail polishes, especially those that are black, blue, green, and reddish-brown in color, can result in inaccurate readings. If nail polish in these shades cannot be removed, the sensor should be applied to an alternate unpolished site.
Monitoring of Ventilation With Capnography
Monitoring of end-tidal carbon dioxide (ETCO2) in respiratory gases provides an early warning of physiologic and mechanical events that interfere with normal ventilation. Capnography, which measures ETCO2 at the patient’s airway, is increasingly used in the PACU. It allows continuous assessment of the adequacy of alveolar ventilation, cardiopulmonary function, ventilator function, and the integrity of the airway and the breathing circuit. Consequently, it enables early detection of many potentially catastrophic events including the onset of malignant hyperthermia, esophageal intubation, hypoventilation, partial or complete airway obstruction, breathing circuit leaks or disconnections, a large pulmonary embolus, and cardiac arrest.
Two variants of the instrument are available. A capnometer provides numeric measurement of exhaled CO2 levels. A capnograph provides the same numeric information, and it also displays a CO2 waveform. Both types of instruments usually incorporate an adjustable alarm system and often have trending and printing capabilities. The following discussion focuses on the use of capnographs because they allow more complete and effective patient assessment than do capnometers. As discussed subsequently, changes in the shape of the CO2 waveform can provide crucial diagnostic information about ventilation, similar to the way in which the waveform provided with an electrocardiogram (ECG) can provide crucial diagnostic information about the heart.
Technology Overview
For measurement of exhaled CO2, the most common type of capnograph passes infrared light at a wavelength that is absorbed by CO2 through a sample of the patient’s respiratory gas. The amount of light absorbed by the patient’s gas reflects the amount of CO2 in the sample.
Capnographs differ in the manner in which they obtain respiratory gas samples for analysis. Sidestream (or diverting) capnographs transport the sample through narrow-gauge tubing to a measuring chamber. Mainstream (or nondiverting) capnographs position a flow-through measurement chamber directly on the patient’s airway. Special adapters are available to allow sidestream capnographs to be used on patients who are not intubated. The sample adapter should be placed as close to the patient’s airway as possible.
Sidestream capnographs incorporate moisture-control features that are designed to minimize clogging of the sample tube, protect the measurement chamber from moisture-induced damage, and minimize the risk of cross contamination. The design of these moisture-control systems significantly affects a monitor’s ease of use. Most systems rely on water traps, which must be emptied routinely. A new technology uses a special system of filters and tubing to dehumidify the sample and thus eliminate the need for water traps.
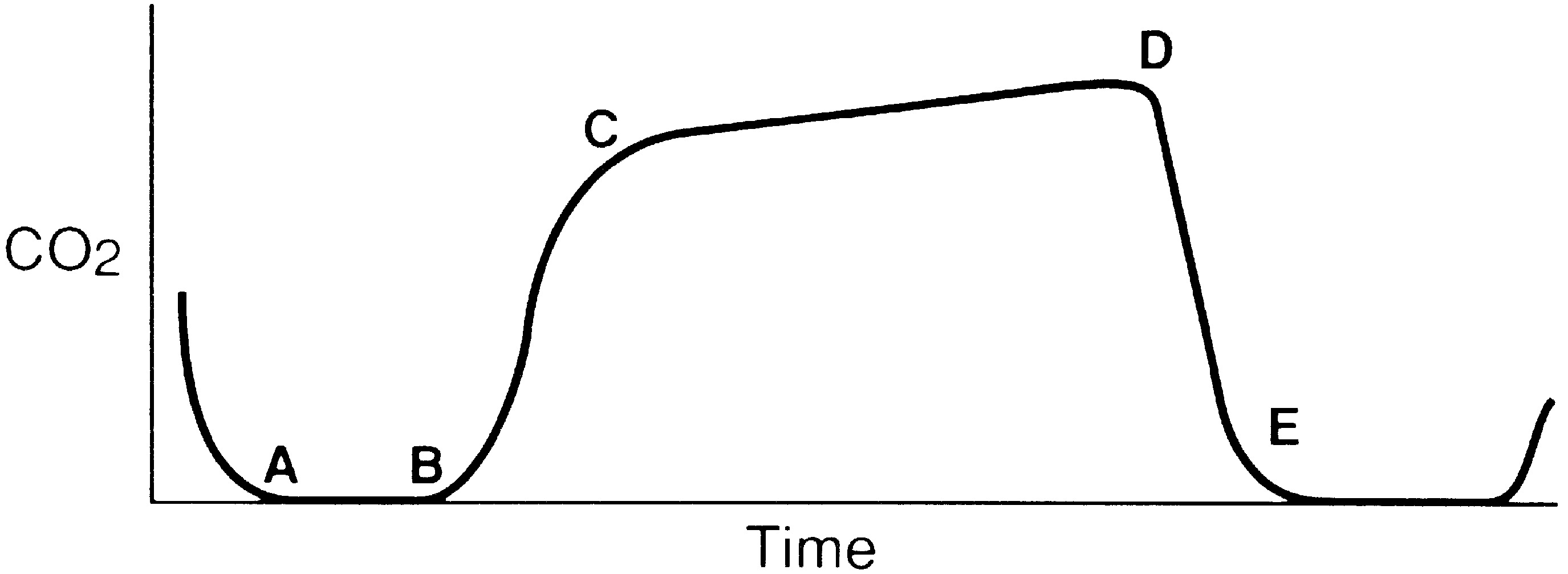
FIG. 27.2 Normal capnogram. (A, B) Beginning exhalation, dead space; (B, C) initial alveolar emptying; (C, D) end-alveolar emptying; (D) end-tidal CO2; (E) inspiration (CO2-free gas).
Capnographs also differ in calibration requirements. Many require removal of the patient from the respiratory circuit and adjustment of the instrument with special mixtures of calibration gases. Advanced capnographic technology includes automatic calibration and does not require any user calibration skills or time.
The Normal Capnogram
For effective use of capnography, it is important to understand the components of the normal CO2 waveform (capnogram)—a square-wave pattern with a plateau (Fig. 27.2). Early in exhalation, air from the anatomic dead space, which is virtually CO2 free, is measured with the instrument. As exhalation continues, alveolar gas reaches the sampling site, and the CO2 level increases rapidly. The CO2 concentration continues to increase throughout exhalation and reaches the alveolar plateau because alveolar gas dominates the sample. At the end of exhalation, the ETCO2 occurs, which in the normal lung is the best approximation of alveolar CO2 levels. The CO2 concentration then drops rapidly as the next inhalation of CO2-free gas begins.
End-Tidal Versus Arterial Carbon Dioxide
In normal conditions, when ventilation and perfusion are well matched, ETCO2 closely approximates arterial CO2 (PaCO2). The difference between the PaCO2 and the ETCO2 level is the alveolar-arterial CO2 difference (a-ADCO2). ETCO2 is usually as much as 5 mm Hg lower than PaCO2. When the two measurements differ significantly, an anomaly in the patient’s physiology, the breathing circuit, or the capnograph is usually present. Significant divergence between ETCO2 and PaCO2 is often attributable to increased alveolar dead space. CO2-free gas from nonperfused alveoli mixes with gas from perfused regions, thus decreasing the ETCO2 measurement. Clinical conditions that cause increased dead space, such as pulmonary hypoperfusion, cardiac arrest, and embolic conditions (e.g., air, fat thrombus, amniotic fluid), can increase the a-ADCO2. Changes in the a-ADCO2 can be used in assessing the efficacy of the treatment; as the patient’s dead space improves, the partial pressure of the alveolar carbon dioxide less the partial pressure of arterial carbon dioxide (PACO2 – PaCO2) narrows. Increases in dead space ventilation lower ETCO2 and therefore increase the PaCO2 – ETCO2 gradient. Widened PaCO2 – ETCO2 examples include embolic phenomena, hypoperfusion, and chronic obstructive pulmonary disease. Alternatively, a significant PACO2 – PaCO2 value can indicate incomplete alveolar emptying (e.g., with reactive airway disease), a leak in the gas-sampling system that allows loss of respiratory gas, and contamination of respiratory gas with fresh gas.4
Interpretation of Changes in the Capnogram
An abnormal capnogram provides an initial warning of many events that warrant immediate intervention. Abnormalities may be seen on a breath-by-breath basis or when the CO2 trend is examined. For this reason, visualization is preferable of both the real-time waveform and the CO2 trend on the monitor display. Examples follow that examine changes produced by significant events that commonly occur in the PACU.
A sudden decrease in ETCO2 to a near-zero level indicates that the monitor is no longer detecting CO2 in exhaled gases (Fig. 27.3). Immediate action is crucial for detection and correction of the cause of this loss of ventilation. Possible causes include a completely blocked endotracheal tube, esophageal intubation, a disconnection in the breathing circuit, and inadvertent extubation. The latter three possibilities are particularly likely if the decrease in ETCO2 coincides with movement of the patient’s head. First, after elimination of possible clinical causes for this decrease in ETCO2, a clogged sampling tube or instrument malfunction is investigated as the cause of the problem.
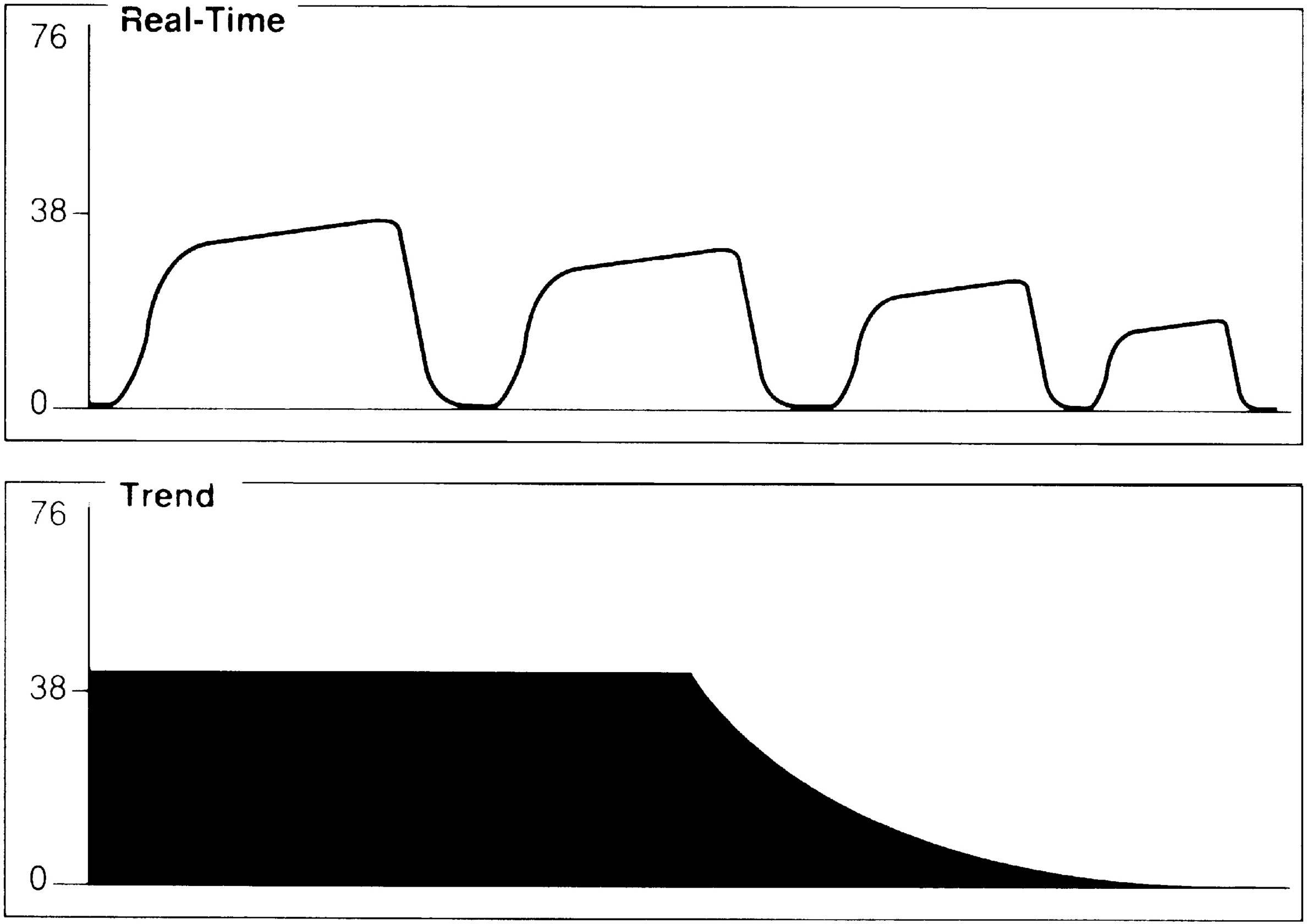
FIG. 27.4 Exponential decrease in ETCO2: Possible causes include hyperventilation, pulmonary embolus, hypotension, and circulatory arrest.
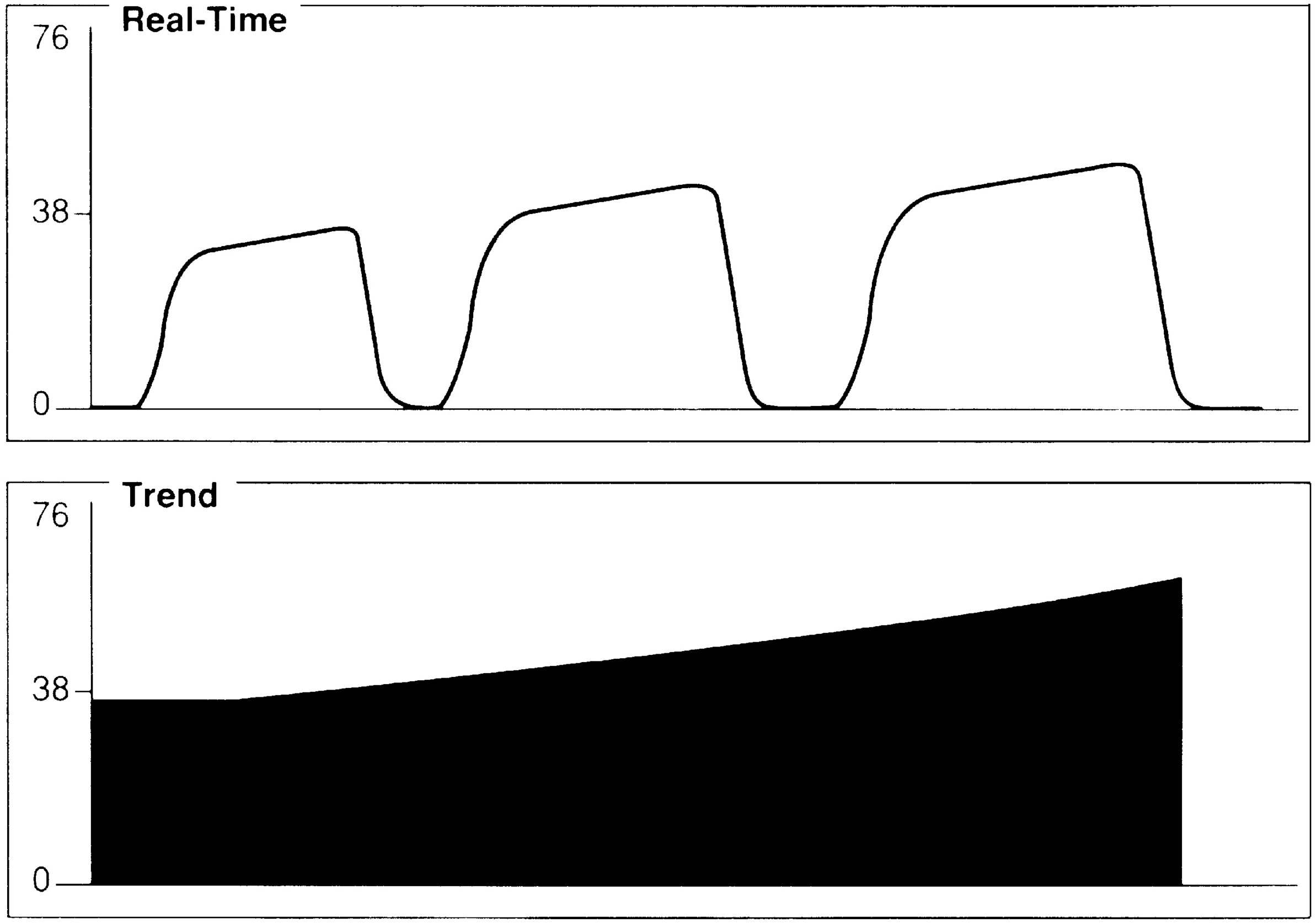
FIG. 27.5 Gradual increase in ETCO2. Possible causes include ventilator leak, partial airway obstruction, hyperthermia, shivering, and malignant hyperthermia.
An exponential decrease in ETCO2 over a small number of breaths usually signals a life-threatening cardiopulmonary event that has dramatically increased dead space ventilation (Fig. 27.4). Sudden hypotension, pulmonary embolism, and circulatory arrest with continued ventilation must be considered.
A gradual increase in the ETCO2 level while the capnogram retains its normal shape usually indicates that ventilation is inadequate to eliminate the CO2 that is produced (Fig. 27.5). This situation can be the result of a small ventilator leak or a partial airway obstruction that reduces minute ventilation. It can also reflect increased CO2 production associated with increased body temperature, the onset of sepsis, or shivering. Of particular importance, a large increase in ETCO2 can be one of the earliest signs of malignant hyperthermia, which may not begin until after emergence from anesthesia.
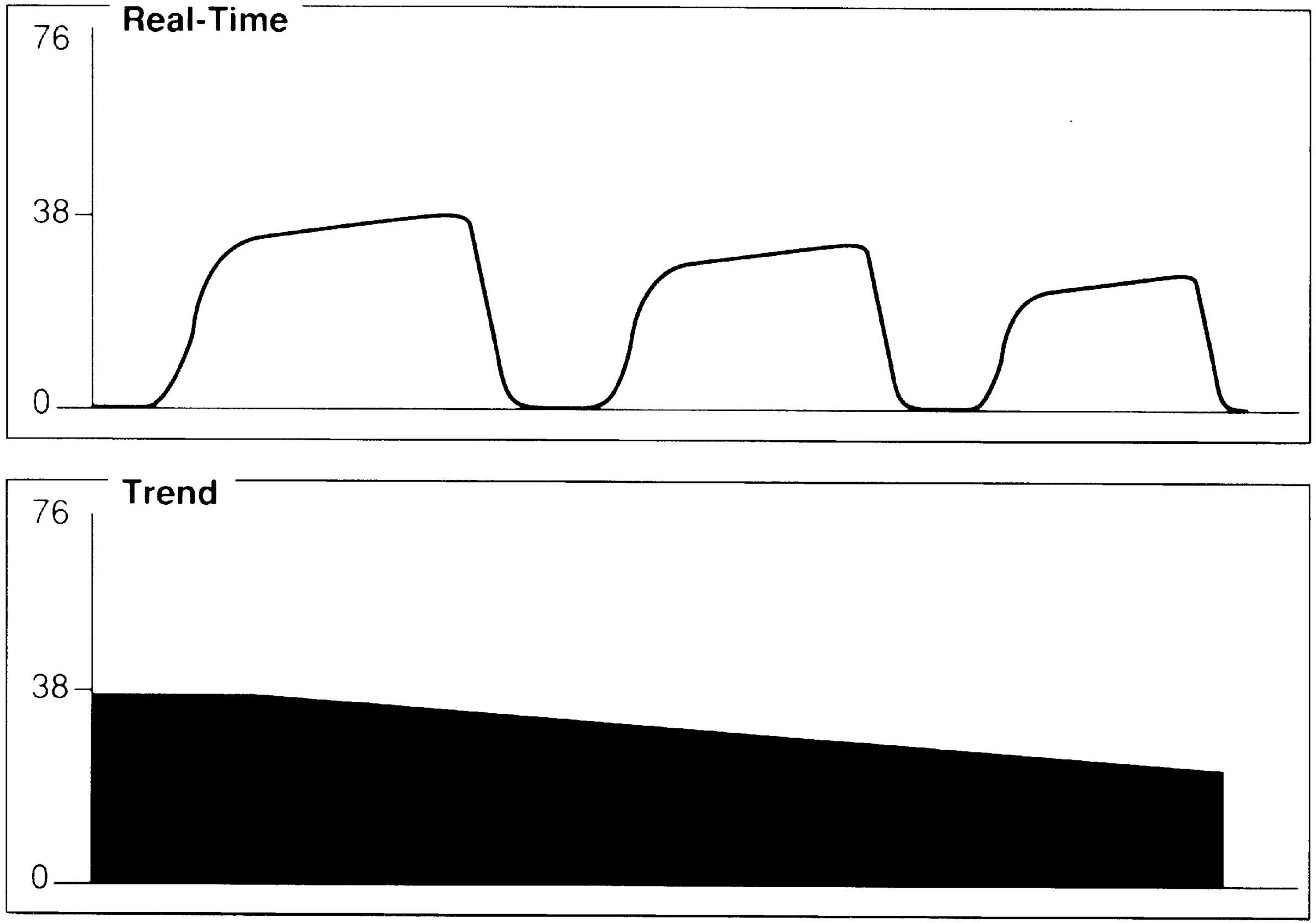
FIG. 27.6 Gradual decrease in ETCO2. Possible causes include hyperventilation, hypothermia, anesthetized, and patient narcotized.
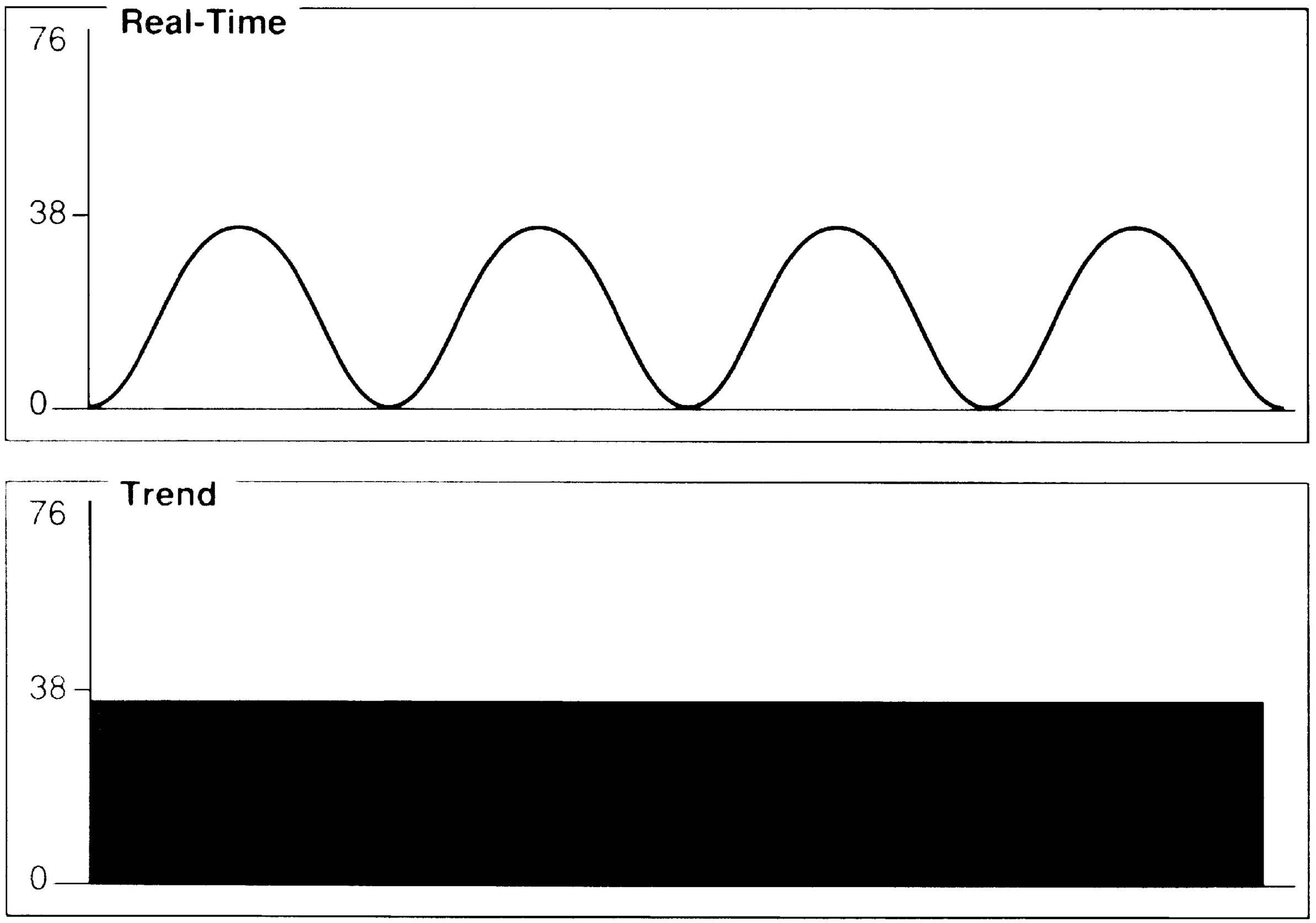
FIG. 27.7 Incomplete alveolar emptying. Possible causes include bronchospasm, obstruction in airway, or breathing circuit.
A gradual decrease in the ETCO2 level commonly occurs in the patient who is anesthetized, narcotized, hyperventilated, or hypothermic (Fig. 27.6).
Assessment of the capnogram can reveal information about the quality of alveolar emptying. For example, the patient with bronchospasm is unable to completely empty the alveoli, and the resulting capnogram does not have an alveolar plateau (Fig. 27.7). The ETCO2 reported by the capnograph in this instance is not a good estimate of alveolar CO2. Effective administration of bronchodilator therapy commonly improves alveolar emptying and results in a more normal capnogram.
Clinical Issues
In addition to the diagnostic usefulness of changes in the capnogram, some specific applications of capnography are particularly valuable in the PACU. Of primary importance is its ability to provide early warning of hypoventilation that, in the PACU, may be the result of anesthesia, sedation, analgesia, or pain. A falling ETCO2 value may indicate pulmonary hypoperfusion from blood loss or hypotension. During rewarming, ETCO2 values are likely to increase as metabolic activity increases.
Capnography can signal when shivering produces an unacceptable increase in oxygen consumption and metabolic rate. During ventilator weaning, capnography is valuable in assessing adequacy of ventilation. Recent studies have shown the value of capnography in monitoring the course and efficacy of cardiopulmonary resuscitation (CPR). ETCO2 measurements, which decrease during cardiac arrest, typically reach approximately 50% of normal levels during effective CPR. When spontaneous circulation is restored, ETCO2 values increase dramatically. The presence and persistence of normal ETCO2 values are also useful determinants in confirming tracheal intubation, because CO2 is not normally found in the esophagus. However, capnography cannot be substituted for chest auscultation and radiography in elimination of the possibility of bronchial intubation.
Importantly in the PACU, capnography can provide critical information about the patient’s ventilatory status, including an early warning of apnea that results in overall patient safety.2,5 Deterioration of a patient is discernable 2 to 3 minutes earlier in a patient with an ETCO2 reading than with oxygen saturation. Therefore, capnography is a helpful assessment for patients who are extremely sedated, who require high doses of opioids, or who have OSA.2,5,6
Practice Recommendation 10 in the 2015–2017 ASPAN Standards addresses the topic of OSA in the adult patient. The recommendations include monitoring of ETCO2 in the phase I PACU to promote perianesthesia patient safety for the OSA patient. Each institution should have a multidisciplinary guideline in place to meet the needs of its patient population. The seven recommendations identified in the ASPAN Practice Recommendation 10 are3:
1. Assess and screen patients for risk factors/comorbidities associated with OSA.
2. Assess and screen undiagnosed patients for signs and symptoms of OSA.
3. Incorporate the use of standardized screening tool to identify patients at risk for OSA.
4. Initiate postanesthesia management of the patient with diagnosed or suspected OSA with management suggestions for phase I and phase II levels of care.
5. Plan for patient discharge with diagnosed or suspected OSA: phase I.
6. Plan for patient discharge with diagnosed or suspected OSA: phase II.
7. Provide discharge education to patients with suspected/diagnosed OSA.
Cardiovascular Function and Perfusion
The three basic components of the circulatory system that must be evaluated are (1) the heart as a pump, (2) blood, and (3) the arteriovenous system. The maintenance of good tissue perfusion depends on a satisfactory CO; therefore, most assessment is aimed at evaluating CO.
Clinical Assessment
Observe the overall condition of the patient, especially skin color and turgor. Peripheral cyanosis, edema, dilatation of the neck veins, shortness of breath, and many other findings may be indicative of cardiovascular problems. In addition to checking all operative sites for blood loss, note the amount of blood lost during surgery and the patient’s most recent hemoglobin level.
Blood Pressure Monitoring
Arterial blood pressure must be assessed in the preoperative physical assessment, on admission to and discharge from the PACU, and at frequent regular intervals during the PACU stay. Arterial blood pressure is the pressure of blood in the arteries and arterioles. It is a pulsatile pressure because of the cardiac cycle, and systolic (peak) and diastolic (trough) numbers are reported in millimeters of mercury. Arterial blood pressure is regulated by the vasomotor tone of the arteries and arterioles, the amount of blood entering the arteries per systole, and blood volume. The pressure is currently measured either noninvasively (indirectly) or invasively (directly). Noninvasive methods include manual cuff measurement with either an aneroid sphygmomanometer or automatic measurement with an electronic blood pressure monitor. Invasive measurement can be accomplished via an arterial line connected to a transducer. A clear understanding of proper technique is essential to ensure accurate and reliable readings with all the blood pressure measurement methods.
Noninvasive Measurement
Manual Method
An aneroid sphygmomanometer with inflatable cuff and stethoscope is needed for the standard auscultatory blood pressure measurement technique. The cuff is placed on the extremity around the arm or thigh. The pressure may be auscultated over the brachial artery of an arm cuff or popliteal artery at the ankle over the posterior tibial artery (just posterior to the medial malleolus). Systolic pressure in the legs is usually 20 to 30 mm Hg higher than in the brachial artery. Use of the correct cuff size is essential. The width of the inflatable bladder encased inside the cuff should be 40% to 50% of the upper arm or thigh circumference. A bladder that is too wide underestimates blood pressure, whereas a bladder that is too narrow overestimates blood pressure. The length of the cuff bladder should be at least 80% of the arm circumference.
The cuff is inflated, and when cuff pressure exceeds the arterial pressure, arterial blood flow ceases and the pulse is no longer palpated. As pressure is released with turning the valve of the inflation bulb, blood flow resumes and audible (Korotkoff) sounds are noted with the stethoscope. These sounds change in quality and intensity throughout further cuff deflation and generally disappear. Systolic pressure is noted as the first audible sound in the cuff-deflating process.7 The diastolic pressure is marked by the disappearance of sounds in the adult patient and the muffling of sounds in the pediatric patient.
A common cause of error in blood pressure measurement is an auscultatory gap that may be present especially in patients with hypertension. This gap is a silent interval between the systolic and diastolic pressures. During this gap, the pulse is palpable. Therefore, to avoid mistakenly low systolic readings, the cuff should be inflated until the pulse is obliterated. Blood pressure readings should be recorded completely including the systolic pressure, the points at which the sounds become muffled and cease, and the range of the auscultatory gap, if present.
Auscultatory blood pressure measurements can be completed quickly and easily in many circumstances. The accuracy and reliability of the readings can be affected by low-flow states (including decreased CO and vasoconstriction) or decreased sound transmission caused by factors related to the patient (edema and obesity) or the environment (noise). Cuff size and placement, user error, and improperly calibrated manometers can also contribute to unreliable readings. Because measurements are intermittent and must be initiated by the user, blood pressure changes may go unnoticed in the postoperative patient with labile hemodynamics or sudden blood loss. The use of automatic blood pressure monitors that can be set to measure blood pressure at regular frequent intervals can minimize some of this risk. Single measurements do not adequately reflect a patient’s blood pressure. Trending blood pressure readings is essential.
Automatic Method
Automatic blood pressure monitoring with electronic devices has become increasingly prevalent in the PACU. The devices are commonly used for frequent blood pressure measurements over relatively brief periods, when the need for arterial sampling is minimal to absent, and when the risks of arterial lines cannot be justified.
One of the most commonly used automatic noninvasive blood pressure methods is based on oscillometric technology. The cuff is chosen and applied according to conventional technique. Oscillations of the arterial wall are occluded as the cuff is inflated and are detected during cuff deflation. Systolic pressure is indicated at the onset of oscillations. As cuff pressure decreases, oscillations increase in amplitude and peak at the mean arterial pressure. The point at which oscillations disappear is the diastolic pressure (Fig. 27.8). All three pressures are normally reported on oscillometric monitoring devices.
Automatic noninvasive blood pressure monitors can be set to cycle at various measurement periods. The instruments alarm when systolic and diastolic pressures register outside of a preset range. Equipment should be calibrated on a regular basis, and preventive maintenance should include assessment for leaks. The use of automatic devices may be limited in patients with low-flow states or high peripheral vascular resistance and in those who are severely obese or edematous. These devices provide only intermittent measurements and are less desirable for assessment of the patient who is labile.
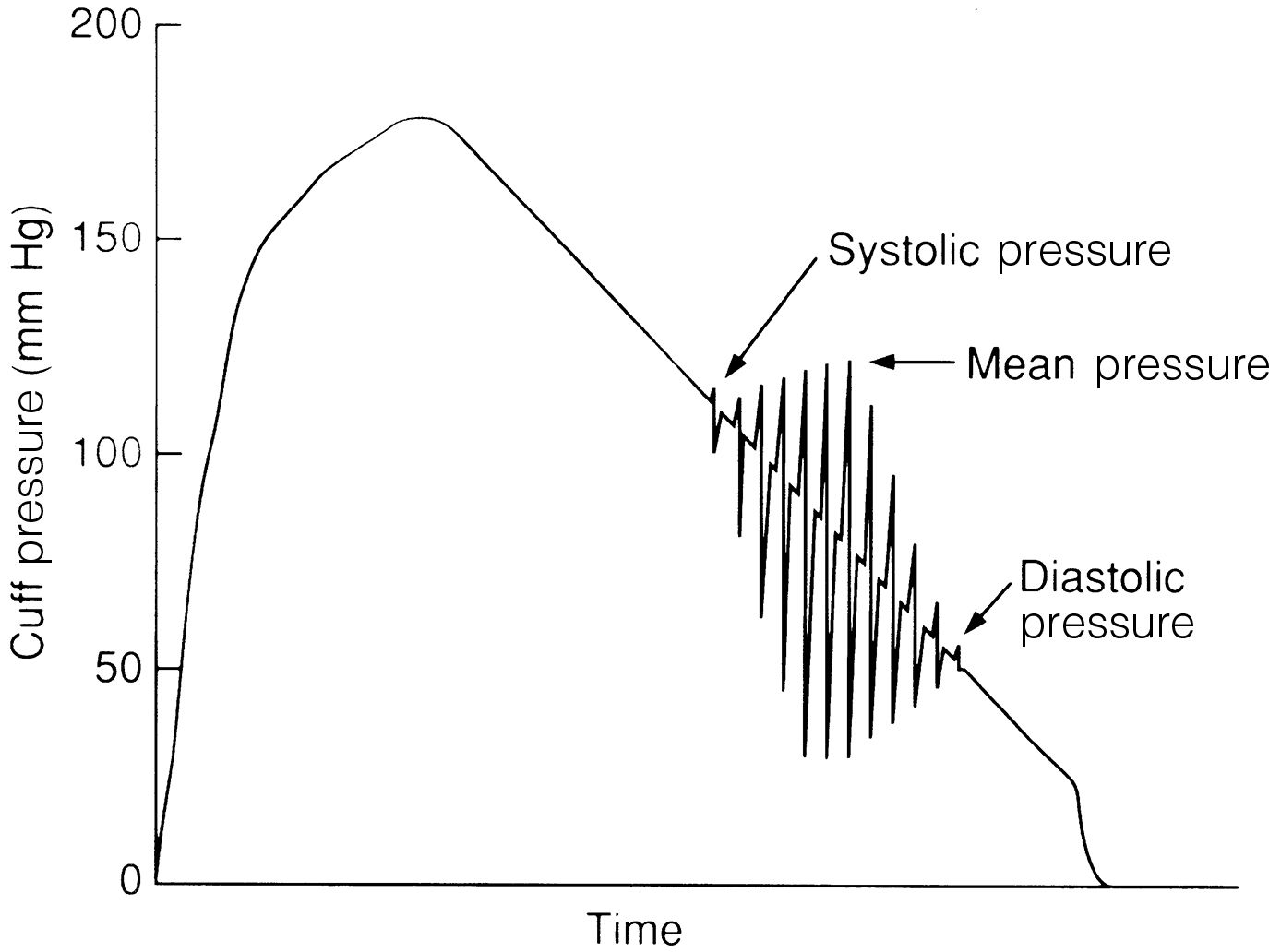
FIG. 27.8 Oscillometric blood pressure measurement. Systolic pressure is indicated at onset of oscillations. Mean arterial pressure occurs when oscillations peak in amplitude. Point at which oscillations disappear is diastolic pressure.
Newer advances in noninvasive blood pressure technology currently available for intraoperative monitoring of the patient who is anesthetized include continuous monitoring capabilities that ensure detection in sudden blood pressure variations. The only other reliable method of continuous blood pressure monitoring currently available is invasive arterial blood pressure technology.
Invasive Measurement
Invasive arterial pressure measurements are most commonly obtained via cannulation of the radial artery but can also be obtained at brachial or femoral sites. A continuous flush solution is connected to the intraarterial catheter and is slowly infused into the arterial vessel under pressure. The pressure within the artery is transmitted through the column of fluid via noncompliant pressure tubing to the transducer. The transducer then converts this pressure to an electric signal that can be converted to millimeters of mercury and displayed on the monitor. A corresponding arterial waveform or pressure pulse is also displayed on the monitor.
Arterial blood pressure measurements are continuous and are indicated for patients at high risk hemodynamically. Changes in patient pressures can be observed on an ongoing basis. This technology may also be chosen for patients in whom indirect measurements fail because of diminished or absent Korotkoff sounds (i.e., patients who are obese or edematous) and for those with high peripheral vascular resistance. The direct arterial access is also beneficial if the patient needs frequent blood samples for laboratory analysis.
To ensure more reliable arterial blood pressure readings, the clinician should level and zero the system according to the manufacturer’s specifications. Level the transducer by positioning the open air reference stopcock at the fourth intercostal level at the midaxillary line known as the phlebostatic axis. The air reference stopcock is when the stopcock is turned off to the patient and opened to air. Depressing the 0 button on the monitor balances the transducer to atmospheric pressure so that it reads 0 mm Hg. Once the monitor is zeroed, turn the stopcock so that it is open to the patient, and pressure readings will appear. An aseptic technique must always be used during placement and maintenance of the arterial line and transducer.
Damping of the arterial waveform with subsequent unreliable readings may occur for a variety of reasons including clotting and kinking of the arterial catheter, positioning of the catheter against the arterial wall, and the presence of air bubbles within the arterial line system. Loose connections, calibration error, and equipment failure can also contribute to unreliable readings. A square-wave test evaluates the dynamic response of the pressure monitoring system. To perform a square-wave test, activate the fast flush device for 1 to 2 seconds, and note the monitor configuration. The patient waveform will be replaced with a square wave (Fig. 27.9).
An Allen test may be performed before radial artery cannulation for minimization of the risk of hand ischemia. However, the Allen test is not used as often in clinical practice as in the past. Bedside ultrasound instead can be used to evaluate blood flow to the radial artery (see Chapter 11). If arterial lines are discontinued in the PACU, constant pressure should be applied to the site for 5 to 10 minutes or until bleeding has ceased. A pressure dressing should be applied, and the site should be checked frequently for any bleeding and radial pulse palpation.
Complications and risks of invasive arterial blood pressure monitoring include infection, thrombosis, emboli, tissue ischemia, hemorrhage, and vessel perforation. Arterial blood pressure monitoring is generally contraindicated in patients with septicemia, coagulopathies, irradiated arterial sites, anatomic anomalies, inadequate collateral blood flow, or thrombosis. No intravenous solution or medications should be administered through the arterial pressure monitoring system at any time. If the catheter patency is in question, blood and fluid are aspirated from the blood drawing port or stopcock, and the system is flushed with the fast flush device, not a syringe.2,8
Clinical Issues
For assessment of significance, blood pressure readings in the postoperative period must be compared with preoperative baseline measurements. A low postoperative blood pressure may be the result of a number of factors including the effects of muscle relaxants, spinal anesthesia, preoperative medication, changes in the patient’s position, blood loss, poor lung ventilation, and peripheral pooling of blood. The administration of oxygen to help eliminate anesthetic gases and to assist the patient in awakening causes an increase in blood pressure. Deep breathing, leg exercises, verbal stimulation, and conversation can be instituted to raise the blood pressure. A low fluid volume may be augmented by increasing the rate of intravenous fluids, which helps to maintain the arterial pressure. Any method designed to raise the pressure must be instituted with consideration for the patient’s overall condition.
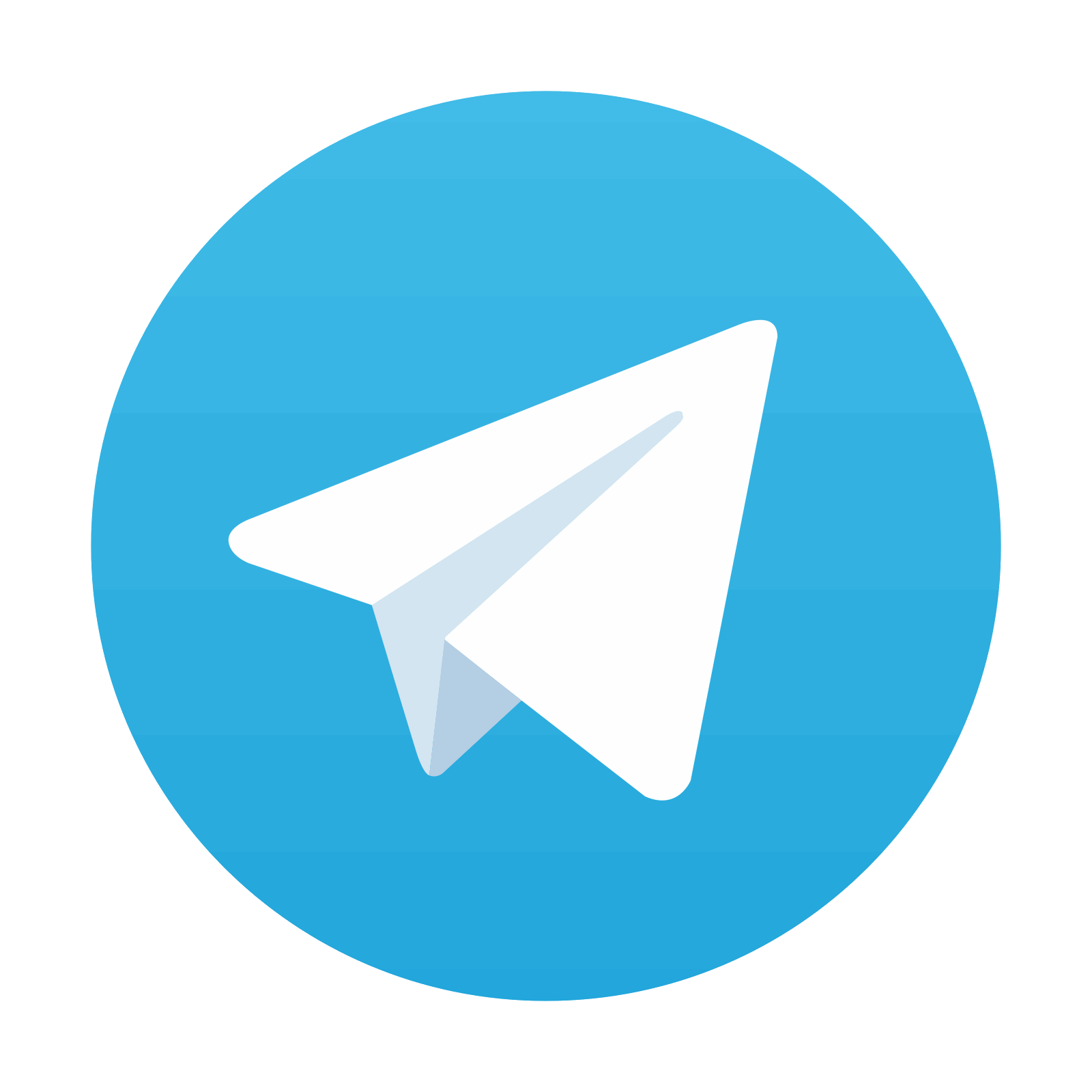
Stay updated, free articles. Join our Telegram channel

Full access? Get Clinical Tree
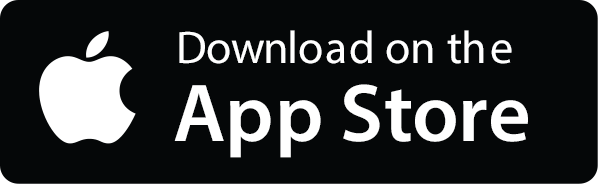
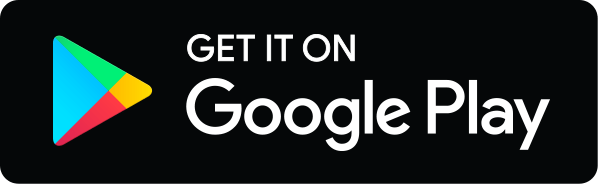