“Anaesthesia-paralysis” is the title of a review article published in 1897 by Dr. Henry J. Garrigues, an obstetrician at the New York Maternity Hospital. In this review, he lauds the introduction of surgical anesthesia as “the fairest leaf in the rich wreath of laurels won by American surgery;” however, even early in the history of general anesthesia use in this country, it was recognized that this blessing was not without its own untoward consequences. He writes, “This blessing to suffering humanity, by which alone the gigantic strides of modern surgery have become possible, is however, not free from danger.” He proceeds to describe a complication of anesthesia: multiple limb paralysis after surgical procedures mostly seen with obstetrical surgery. More than 110 years later, an editorial characterizing perioperative nerve injury as anesthesia’s “silent scream” was published in a prominent anesthesiology journal. It addressed the incidence of and damage resulting from intraoperative peripheral nerve injury secondary to a combination of positioning and the anesthesia. These were severe neurologic complications, sometimes resulting in lifelong disability. Modern anesthesia techniques have dramatically improved outcomes after surgery, even for the most dangerous and complex procedures. However, for many reasons, the occurrence of intraoperative nerve injury has not abated.
The modern demands for extreme patient positioning in order to improve surgical access continue to test and tax our ability to position and secure our patients safely on the operating table. These extreme positions can increase the stretch of vulnerable peripheral nerves while efforts to secure the patient safely can lead to increased pressure on nerves. In addition, the advancements in patient monitoring and safety of the anesthetic drugs coupled with the increased technical complexity of the surgical procedures have resulted in prolonged anesthetic times for the patient, increasing the chances of nerve injury. Increasingly, the comorbidities of the surgical patient population have increased and will continue to increase. Lastly, increases in patient size have also made nerve injury more likely for it is a challenge to position patients correctly who have high body mass indices without the proper equipment.
As anesthesiologists, we are all aware of these risks. However, it is often the case that these risks are not explained in detail to the patient. While the risk of dental damage, blood transfusion, or cardiac complications is carefully discussed with the patient, it is rare that a discussion regarding the risk of a severe motor and sensory deficit would take place. Many factors contribute to this lapse. (1) Nerve injuries are perceived as rare complications. (2) It is difficult to predict where the damage might occur. (3) Nerve injuries are considered to pale in terms of gravity when compared to the primary reason for the operation and other complications such as heart attack, stroke, and death. But are intraoperative nerve injuries really too insignificant to discuss preoperatively? Paralysis, blindness, or sympathetic dystrophy (Chronic Regional Pain Syndrome) is a lifelong burden. The ramifications of unexpected postoperative blindness obviously are unfathomable. Even a more “minor” injury such as a persistent ulnar nerve palsy is career ending for anyone who has a job that requires fine motor dexterity such as an artist, musician, or anesthesiologist.
Currently, the estimated incidence of peripheral nerve injury (PNI) associated with an anesthetic is 15% of American Society of Anesthesiologists (ASA) closed claims. The incidence of postoperative visual loss, another type of nerve injury, is approximately 0% to 4.5% worldwide. A proper informed consent process should include the discussion of these risks. Who should be discussing the risk of nerve injury with the patient? Ideally, the responsibility of explaining the risks of unusual positioning should be shared among surgeons, nurses, and anesthesiologists since all have an important role in the positioning of the patient and ultimately the safety of the patient in the operating room. A laissez-faire approach to positioning where the anesthesiology team watches the positioning of the patient by the surgical and nursing teams is not appropriate. When these cases come to court, in the muddy waters of jurisprudence it is often the anesthesiologist’s responsibility to help prevent a PNI that is put on trial.
I. POSTOPERATIVE VISION LOSS
A. Introduction
1. Definition
Postoperative vision loss (POVL) was first described in the literature in 1948 and as a complication following neurosurgery in 1954. It is a rare and severely debilitating complication of surgery, primarily documented in patients who have undergone either prolonged spine procedures in the prone position or cardiac operations. The vision loss may occur in one or both eyes.
The exact etiology of POVL has yet to be determined, but it has several known manifestations. The most common form is non-arteritic ischemic optic neuropathy (ION). Non-arteritic ION is further subdivided into posterior (PION) and anterior (AION) forms. PION accounts for the majority of POVL cases. Central retinal artery occlusion (CRAO), cortical blindness (CB), and POVL of unknown origin make up the remainder of the cases.
Of note, outside the operating room (OR) spontaneous non-arteritic ION is well known to ophthalmologists as the most frequent form of acute optic neuropathy resulting in blindness in patients > 50 years of age.
2. Incidence
There are conflicting reports about the rising or declining incidence of POVL. Taken as a group, the reports imply a rise in occurrence. Whether this trend is due to the increased awareness of POVL and better reporting or is secondary to the rise in annual spinal operations utilizing complex instrumentation is unclear. Exact numbers are very difficult to determine and, in the current literature, estimates range from 0% to 4.5%. Data primarily come from case reports, case control studies, and retrospective analysis of data sets. Prospective studies have been performed in cardiac surgery where POVL occurs most commonly. These studies are difficult to accomplish in spine and other surgeries because of the rarity of POVL. Adding to the confusion, inclusion criteria and definitions vary vastly among the studies.
The incidence of persistent POVL in all anesthetics ranges from 1:61,000 to 1:118,783 patients. The highest incidence occurs in cardiac surgery, followed by spine surgery, especially spinal fusions. The most recently published 10-year study examined the incidence of POVL related to eight categories of major surgery and confirmed that the highest rate occurred in patients who had cardiac surgery (8.64 in 10,000), followed by spinal fusion (3.09/10,000), and then laminectomy without fusion (0.86/10,000). The majority of cardiac cases exhibited CRAO (77%), whereas in spine surgery, the incidence of ION is higher at 28% with CRAO at 23% and CB at 49%. Surprisingly, in this retrospective study, data showed that spine surgery patients < 18 years of age appeared to have the highest prevalence of POVL (4.37/10,000). These results need to be interpreted with extreme caution as the mode of data acquisition used in this study by “coders” is controversial.
3. ASA POVL registry
In order to learn more about POVL, the American Society of Anesthesiologists (ASA) established a task force and registry, the American Society of Anesthesiologists Postoperative Visual Loss Registry, composed of voluntarily submitted cases. Patients who had POVL after prone spine surgery constitute the single largest group (67%). Bilateral disease was present in 66% of the cases with complete visual loss in the affected eye(s) in 58%.
Data in the registry show that among the reported cases of POVL that involved spine surgery the predominant mechanism of blindness was ION (89% of the cases). Of these, 60% involved the posterior optic nerve. Other shared features that appear to be correlated to POVL are blood loss ≥1,000 mL (82%) and operative duration over 6 hours (94%) (www.asaclosedclaims.org).
B. The optic canal
1. Anatomy
a. The optic nerve traverses the optic canal in its prechiasma portion. This narrow canal is limited anteriorly by the ocular globe and laterally by bony structures of the frontal and maxillary sinuses. Toward its posterior part, the canal opens within the surrounding cerebral structures. Cerebrospinal fluid (CSF) surrounds the neurovascular bundle up to the globe; the thickness of the CSF layer varies. The space between nerve and bone is 2 to 3 mm wide.
FIGURE 28.1 Diagram (based on camera lucida drawings) showing one of the intraneural branches of the central retinal artery that runs backward in the axial part of the optic nerve posterior to the central retinal artery. CZ = circle of Zinn and Haller; PCA = posterior ciliary artery; CRA = central retinal artery.
b. Cup-to-disc ratio
The optic disc is the anatomical location of the eye’s “blind spot,” the area where the optic nerve and blood vessels enter the retina. The cup is an apparent space centrally. The usual cup-to-disc ratio is about 0.3. The larger the cup, the larger is the scleral opening at the back of the globe. Of relevance, in some patients, there appears to be the innate existence of a smaller canal from which the optic nerve exits the sclera. This causes a small optic cup-to-disc ratio that may lead to ischemic damage even without the presence of other preconditions. The diagnosis of POVL depends on which part of the nerve is affected since the anterior and posterior sections have different blood supplies.
2. Blood supply and blood flow of the optic nerve
a. The optic nerve can be divided into an anterior (optic nerve head) part and a posterior part as determined by the blood supply (Fig. 28.1, Fig. 28.2). The interactions of blood supply and blood flow with systemic and local factors contribute to individual susceptibility to optic nerve ischemia. There are individual variations between patients as well as in each optic nerve in the same patient.
1. The anterior part of the optic nerve is supplied mainly by the posterior ciliary artery (PCA) circulation via the peripapillary choroid and short PCA collateral branches. This network is also called the circle of Zinn and Haller. The sectored distribution of these arterial branches is thought to explain segmental vision loss.
2. The posterior part of the optic nerve is supplied by multiple arteries but not by the PCA. The intracanalicular part is supplied anteriorly by the pial vascular plexus constructed of multiple arterial sources and intraneural branches of the CRA and posteriorly mostly by small collateral arteries primarily from the ophthalmic artery (the first branch of the internal carotid artery [ICA]). The intracanalicular part derives its blood supply from fine collateral branches of the ophthalmic artery as well. The intracranial part has only a pial vascular plexus composed of arterioles from the surrounding arteries, mostly the anterior cerebral artery, middle meningeal artery, ophthalmic, and hypophyseal arteries.
FIGURE 28.2 Diagrammatic representation of blood supply of the various parts of the optic nerve, and location of the circle of Haller and Zinn (CZ), as seen from above. Blood supply of: (A) the optic nerve head and (B) the optic nerve Abbreviations: A, arachnoid; Ant. Sup. Hyp. Art., anterior superior hypophyseal artery; C, choroid; CAR and CRA, central retinal artery; Col. Br., collateral branches; CRV, central retinal vein; CZ, circle of Zinn and Haller; D, dura; ICA, internal carotid artery; LC, lamina cribrosa; LPCA, lateral posterior ciliary artery; Med. Mus., medial muscular artery; MPCA, medial posterior ciliary artery; NFL, surface nerve fiber layer of the disc; OA, ophthalmic artery; OD, optic disc; ON, optic nerve; P, pia; PCA, posterior ciliary artery; PR and PLR, pre-laminar region; R, retina; RA, retinal arteriole; Rec. Br. CZ, recurrent pial branches from peripapillary choroid/CZ; S, sclera; SAS, subarachnoid space.
Although highly variable, the venous drainage occurs primarily by way of the peripapillary choroidal veins into the central retinal vein and, to a lesser extent, the orbital veins and ultimately into the cavernous sinus.
b. There is substantial anatomical interindividual variability in the ocular blood supply between patients and even between the two eyes of the same patient. The pattern of PCA circulation can differ with regard to the number of PCA branches, the segmental distribution of the supplied area, and the location of watershed zones between the PCAs. The location of the watershed zones determines the vulnerability of the optic nerve head (ONH) to ischemia as in the case of a fall in perfusion pressure. Furthermore, anatomical differences within the PCAs render some PCAs more prone to ischemia during episodes of arterial hypotension.
c. Blood flow to the optic nerve depends on perfusion pressure, resistance to flow, presence of autoregulation, and rheologic properties of the blood. The resistance to blood flow depends on the autoregulation of ONH blood flow, vascular blood supply, and rheologic properties of the blood.
1. The perfusion pressure of the orbit is the mean arterial pressure (MAP) minus intraocular pressure (IOP). MAP represents the major determinant of perfusion pressure of the eye. Animal studies have shown that arterial hypotension can decrease the blood flow to the optic nerve significantly. Oxygen delivery to the optic nerve can be similarly decreased during systemic hypotension alone and even more so when hypotension is associated with anemia and venous congestion.
2. Autoregulation of orbital perfusion breaks down at a MAP < 30 mm Hg in patients who have normal vasculature and at higher pressures in patients who have concomitant vascular disease. The posterior portion of the optic nerve derives its main vascular supply from pial vessels that come from branches of the ophthalmic artery. These vessels are incapable of autoregulatory control so that the posterior optic nerve is particularly vulnerable to a fall in perfusion pressure. This part of the nerve is also readily injured by a drop in oxygen availability as from anemia. Clinically, increasing the IOP in the setting of low systemic BP may reduce ocular perfusion pressure (OPP) beyond the point of the autoregulatory mechanism’s ability to compensate.
d. An IOP < 5 mm Hg is referred to as ocular hypotony. An IOP > 21 mm Hg is considered abnormally high and is often referred to as ocular hypertension. IOP increases significantly during surgery in the prone position and rises further as the duration of the operation increases. It may occasionally reach up to three times the patient’s baseline pressure, exceeding 21 mm Hg and thus putting susceptible patients at risk for a reduction in perfusion pressure and inadequate ocular perfusion. An increase in arterial carbon dioxide tension PaCO2 seems to increase the IOP. The clinical significance of this for ocular nerve perfusion during anesthesia is unknown.
C. What do we know about POVL?
1. Signs and symptoms of POVL
POVL will often appear within the first 24 hours after operation, or sometimes later, as a painless loss of some or all sight. When it is a partial loss, the visual field defects often obey the horizontal midline. These patients may have sluggish pupils or an afferent pupillary defect. All other causes of acute loss of vision must be ruled out. Unfortunately, POVL is frequently bilateral and irreversible at this point in time.
a. Ischemic optic neuropathy is an acute ischemic disorder of the optic nerve.
1. In anterior ischemic optic neuropathy (AION), the fundoscopic examination reveals edema of the optic disc and, occasionally, hemorrhage. An afferent pupillary defect may also be found. Binocular involvement (60%) is more common than monocular involvement (40%). Sixty percent of the patients are female. The 40% of patients who are male are usually middle aged, almost always have undergone lumbar surgery, and have a higher incidence of vascular risk factors, especially coronary artery disease (CAD), diabetes mellitus (DM), and obesity. The onset of AION occurs within 48 hours in 40% of the cases. In about 20%, the onset can be delayed to the ninth postoperative day. In such cases, it is difficult to differentiate spontaneous AION from postoperative AION.
2. In posterior ischemic optic neuropathy (PION), the optic disc at the time of onset of the visual loss appears normal without edema of the disc. Disc edema may appear several days later as the swelling progresses into the anterior region. Occasionally, there is an afferent pupillary defect and a non-reactive pupil. There are no signs of retinal ischemia (cherry-red spot). As with AION, binocular involvement (53%) is generally more common than monocular involvement (47%). Both eyes are equally affected in approximately 20% to 24% of cases. PION is diagnosed in the majority of cases (59%) upon awakening; an additional 29% are diagnosed within 24 hours. Only 12% occur on postoperative days 2 to 5, but not later.
PION affects more men (70%) and at a younger age with the youngest report at 12 years of age. While PION is more common after lumbar surgery, it can occur after cervical and thoracic operations as well. The operative duration is generally slightly shorter than with AION. Half of the patients have no vascular risk factors; when risk factors are present, the most common is arterial hypertension.
b. Central retinal artery occlusion (CRAO) is caused by embolization of the central retinal artery, which results in ischemic necrosis from a loss of blood supply to the inner layer of the retina. The patient has acute and persistent painless visual loss, an afferent pupillary defect, and a pale, swollen optic nerve with splinter hemorrhages. Occasionally emboli can be seen. Fundoscopic examination within 15 minutes to several hours after the event reveals a pale opacified retina that is yellow white in appearance and a characteristic “cherry-red spot.” In the acute situation, CRAO causes edema of the inner layer of the retina and pyknosis of the ganglion cell nuclei. The arterial occlusion usually resolves within 4 to 6 weeks; however, irreversible cell injury can occur in < 90 to100 minutes of total CRAO. Certainly after 24 hours of retinal ischemia, the damage is permanent. Antiplatelet therapy and heparin have been tried with poor results.
c. Cortical blindness
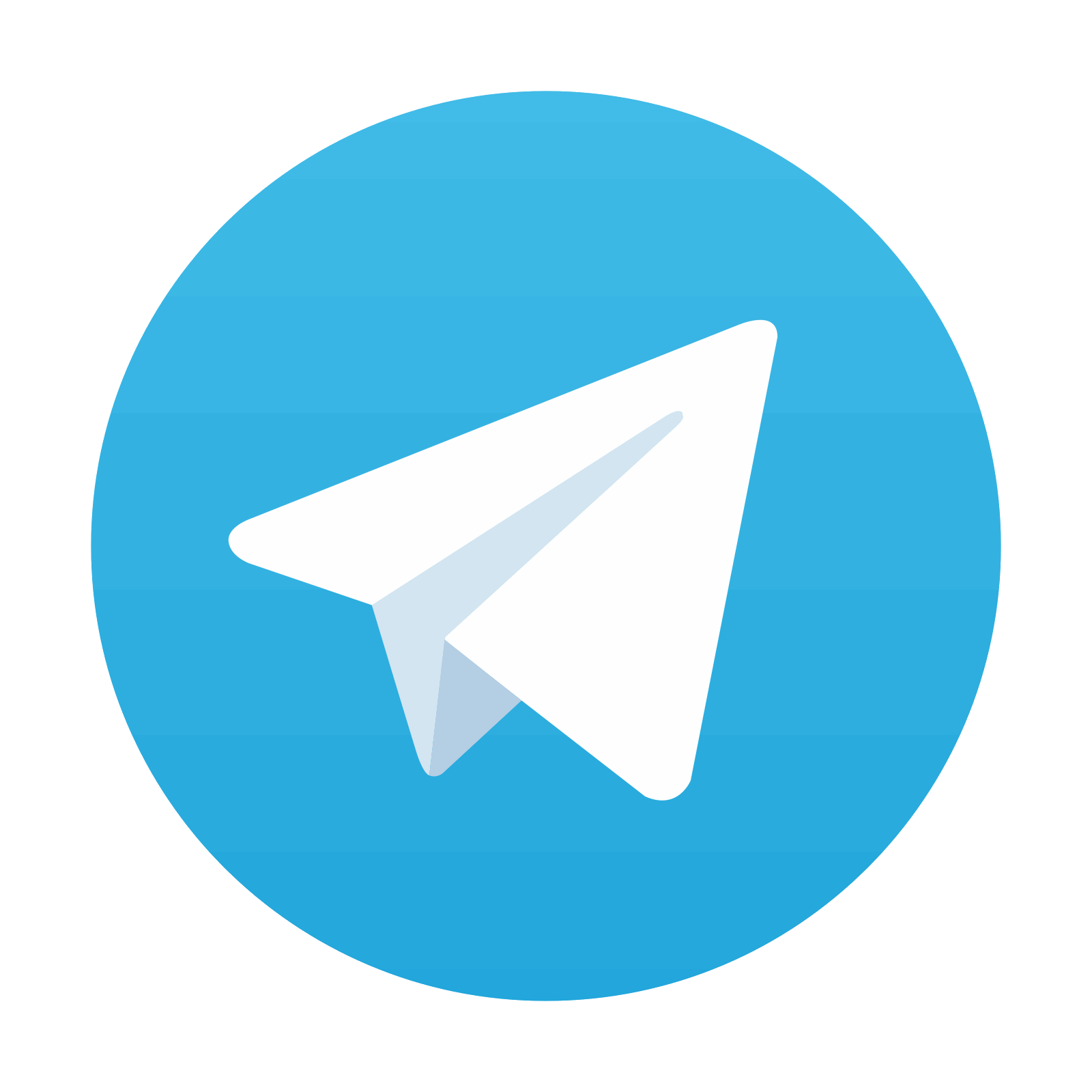
Stay updated, free articles. Join our Telegram channel

Full access? Get Clinical Tree
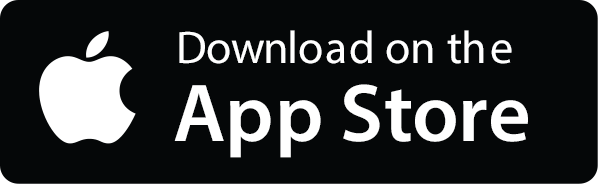
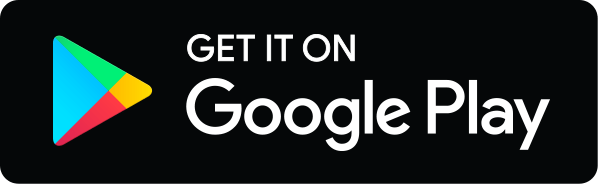