Anticonvulsant Poisoning
Steven B. Bird
Anticonvulsants can be divided into four groups based on their primary mechanism of action: those that primarily act on neuronal membranes (membrane-active agents), those that act on neurotransmitters or their receptor sites (synaptic agents), those with multiple sites of action, and those that are not yet understood. Membrane-active agents alter ion fluxes and include carbamazepine (CBZ), oxcarbazepine, ethosuximide, zonisamide, phenytoin, and lamotrigine (LTG). Synaptic agents primarily affect the activity of gamma-aminobutyric acid (GABA) and include barbiturates, benzodiazepines, gabapentin (GBP), tiagabine, and vigabatrin. Agents that have multiple sites of action include valproate, GBP, felbamate, and topiramate, and those for which mechanisms of action still are not understood are levetiracetam, stiripentol, and remacemide [1,2,3].
(Barbiturates and benzodiazepines are discussed in Chapter 143.) The precise action mechanisms of many of the newer anticonvulsants also remain unknown. Even within groups, the site or mechanism of action may differ. Pharmacologic differences are important from a therapeutic standpoint. In the treatment of seizures, combining agents from different groups may be effective whenever a single agent is ineffective or requires a toxic dose for efficacy. Therapeutic synergism may also occur when different agents of the same group are combined (e.g., benzodiazepines and barbiturates).
(Barbiturates and benzodiazepines are discussed in Chapter 143.) The precise action mechanisms of many of the newer anticonvulsants also remain unknown. Even within groups, the site or mechanism of action may differ. Pharmacologic differences are important from a therapeutic standpoint. In the treatment of seizures, combining agents from different groups may be effective whenever a single agent is ineffective or requires a toxic dose for efficacy. Therapeutic synergism may also occur when different agents of the same group are combined (e.g., benzodiazepines and barbiturates).
Phenytoin
Phenytoin (diphenylhydantoin) is the most commonly used anticonvulsant medication [4]. It is also used in the treatment of trigeminal neuralgia. Phenytoin was the antidysrhythmic of choice for digitalis toxicity before the advent of digitalis Fab fragments [5]. Iatrogenic intoxications can occur with drug interactions because distribution, protein binding, and clearance of phenytoin are affected by other medications and disease states. Toxicity may occur when the daily-administered dose exceeds endogenous metabolism and elimination [6,7,8]. Toxicity may also result when switching dosage forms or between generic and proprietary forms of the drug because of different release and absorption characteristics. There are idiosyncratic and hypersensitivity reactions associated with therapeutic use that are unrelated to dose, most commonly seen in patients with underlying neurologic disorders [9].
Pharmacology
Phenytoin is the prototypic membrane-active anticonvulsant. It acts on sodium pumps and channels in excitable cell membranes and is classified as a type 1B antidysrhythmic agent. By blocking the accumulation of intracellular sodium during tetanic stimulation, it limits the posttetanic potentiation of synaptic transmission and prevents seizure foci from detonating adjacent areas.
Phenytoin is a weak acid, with a pKa of 8.5. The intravenous (IV) form has a pH of 10 to 12, contains 50 mg per mL, and is dissolved in a 40% propylene glycol and 10% ethanol vehicle. The phenytoin prodrug fosphenytoin (Cerebyx) has a pH between 8.6 and 9.0 and greater solubility. It is compatible with common IV preparations, lacks the cardiotoxic diluent propylene glycol, and may be administered intramuscularly as well as intravenously. It has a conversion half-life of 8.4 to 32.7 minutes to active phenytoin and is dosed in phenytoin equivalent (PE) units (75 mg per mL of fosphenytoin equals 50 mg per mL of phenytoin) [10]. In many institutions, fosphenytoin has replaced phenytoin.
Absorption occurs in the duodenum but depends on dosage form, gastric emptying, and bowel motility. Peak levels occur between 2.6 and 8.9 hours after oral dosing of an extended-release capsule. In overdosage, absorption may continue for up to 7 days, possibly due to decreased gastric motility and pharmacobezoar formation. The volume of distribution (Vd) of phenytoin is 0.6 L per kg, and it distributes preferentially into the brainstem and cerebellum [11]. Phenytoin is highly protein bound; decreased protein binding increases the free, pharmacologically active form of the drug and the Vd. Because usually only total phenytoin levels are measured, toxicity from increased free phenytoin may occur at lower total phenytoin levels [8].
Hepatic metabolism of phenytoin follows first-order elimination kinetics, with an average half-life of 22 hours (range: 7 to 55 hours). When plasma levels exceed 10 μg per mL, metabolism follows zero-order elimination kinetics, yielding a much longer half-life. The enzyme system may be induced or inhibited by other drugs, inherited genetic disturbances, or liver disease [12,13].
The anticonvulsant effects of phenytoin occur with plasma levels between 10 and 20 μg per mL. This can be achieved within 45 to 60 minutes by an IV-loading dose of 15 to 20 mg per kg of phenytoin or PE units of fosphenytoin. The rate of IV phenytoin administration should not exceed 50 mg per minute because of propylene glycol toxicity [14]. To avoid hypotension, fosphenytoin administration should not exceed 150 PE units per minute. Phenytoin has been successfully administered by the interosseous route in children with poor venous access. Maintenance dosing is usually 4 to 6 mg per kg per day in single or divided doses, although neonates may require higher doses (5 to 8 mg per kg per day) [15]. Death from isolated phenytoin ingestions is unusual but has been reported in young children with ingestions of 100 to 220 mg per kg [16,17]. Death results from central nervous system (CNS) depression with respiratory insufficiency and hypoxia-related complications.
Clinical Manifestations
Toxicity resulting from acute and chronic intoxication has a similar presentation. Patients with serum phenytoin concentrations between 20 and 40 μg per mL typically have nausea, vomiting, normal to dilated pupils, nystagmus in all directions, blurred vision, diplopia, slurred speech, dizziness, ataxia, tremor, and lethargy [18]. They may also be excited and agitated. As phenytoin serum concentration increases, confusion, hallucinations, and apparent psychosis may develop. Progressive CNS depression occurs, and nystagmus may improve. Pupillary response becomes sluggish, and deep tendon reflexes diminish [7]. Severe toxicity with coma and respiratory depression occurs with serum concentration exceeding 90 μg per mL [19]. Slowing of alpha wave activity is seen on electroencephalograms. As toxicity increases, brainstem evoked potentials are suppressed and may be absent. Paradoxical hyperactivity has been reported in patients with underlying neurologic deficits, with findings of dystonia, dyskinesia, choreoathetoid movements, decerebrate rigidity, and increased seizure activity [7,20]. Patients with baseline focal neurologic deficits may show contralateral abnormalities, including hemianopia, hemianesthesia, and hemiparesis. Patients recover completely if no anoxic or hypoxic complications develop during acute toxicity. Cerebellar atrophy after acute intoxication with phenytoin that was not known to be attributed to hypoxia has been reported, however [21]. Recovery may take 1 week or longer.
In rare instances, chronic toxicity has been associated with a syndrome of inappropriate antidiuretic hormone [22], encephalopathy, and cerebellar degeneration [11]. Chronic use of phenytoin causes hyperglycemia, vitamin D deficiency and osteomalacia, folate depletion, megaloblastic anemia, and peripheral neuropathy. Other adverse drug events include altered collagen metabolism that causes hirsutism, gingival hyperplasia, keratoconus, and hypertrichosis [23].
Non–dose-dependent phenytoin adverse drug events include hypersensitivity reactions such as fever, rash eosinophilia, hepatitis, lymphadenopathy, myositis, a lupuslike syndrome, rhabdomyolysis, nephritis, vasculitis, and hemolytic anemia [9]. Phenytoin administration during pregnancy has resulted in fetal hydantoin syndrome [24].
Phenytoin-induced dysrhythmias, hypotension, congestive failure, respiratory arrest, and asystole result predominately from propylene glycol toxicity during rapid IV phenytoin administration (e.g., > 50 mg per minute). If the rate of infusion is slowed or temporarily halted, these effects usually resolve spontaneously but may persist for 1 to 2 hours [17,25]. Cardiovascular toxicity from phenytoin intoxication itself is rare,
represents significant toxicity, and primarily occurs in patients with underlying cardiac disorders [26,27].
represents significant toxicity, and primarily occurs in patients with underlying cardiac disorders [26,27].
Diagnostic Evaluation
Essential laboratory studies should include sequential serum phenytoin levels (free and total, if available) and levels of other anticonvulsant medications, particularly when enteric-coated dosage form is involved. The interval between drug levels should be based on factors such as severity of intoxication, rate of rise of levels, and time since exposure. Intervals should be more frequent during the initial evaluation phase, while absorption is still occurring, than later, during the postabsorptive phase. In stable patients whose drug levels have peaked or started to decline, it may be appropriate to obtain levels every 12 to 24 hours until they return to the therapeutic range. Recommended laboratory studies include serum complete blood cell count, electrolytes, blood urea nitrogen, creatinine, glucose, albumin, and liver function tests. In hypoalbuminemic patients, the corrected phenytoin concentration is equal to the measured phenytoin concentration multiplied by 4.4 and divided by the serum albumin level. In all deliberate overdoses, an electrocardiogram (ECG) and acetaminophen and salicylate levels should be obtained. Arterial blood gas, chest radiograph, head computed tomography, and lumbar puncture should be obtained as clinically indicated.
The differential diagnosis of phenytoin intoxication includes sedative–hypnotic agents, other anticonvulsants, phencyclidine, neuroleptic agents, and other CNS depressant drugs. Other conditions such as diabetic ketoacidosis; hyperosmolar nonketotic coma; sepsis; CNS infection, tumor, and trauma; seizure disorders; extrapyramidal syndromes; postictal states; and cerebellar abnormalities may also mimic phenytoin intoxication.
Management
Patients should have a rapid evaluation of respiratory status followed by intubation if hypoxia or risk of aspiration is present. Vascular access should be established and the patient placed on continuous cardiac monitoring. If the mental status is abnormal, a fingerstick blood sugar should be obtained. Patients who are hyperglycemic from phenytoin intoxication can be treated with discontinuation of the drug; insulin therapy is rarely required. Flumazenil, the benzodiazepine antagonist, has no role in managing phenytoin intoxication, even if benzodiazepines are part of the polypharmacy overdose, as its use may increase the risk of status epilepticus, particularly in patients with a preexisting seizure disorder.
Hypotension occurring during phenytoin infusion is treated with discontinuation of the infusion and administration of crystalloids. Pressors are rarely necessary. Treatment of cardiac dysrhythmias is supportive, with use of the appropriate antidysrhythmics when indicated. Type IB antidysrhythmic agents should be avoided [28].
Patients with a seizure disorder should be placed on seizure precautions due to the possibility of paradoxical seizures during the acute intoxication phase or breakthrough seizures during the recovery phase when phenytoin levels may be in the subtherapeutic range. Seizures should be treated with benzodiazepines or a different anticonvulsant.
Because phenytoin has a long elimination half-life, measures to increase the rate of elimination should be considered. Gastrointestinal (GI) tract decontamination uses oral-activated charcoal administration. Phenytoin undergoes enterohepatic recirculation with active gut secretion; multiple-dose oral activated charcoal (MDAC) can increase the rate of elimination and may decrease hospital stay [29,30] (see Chapter 117). MDAC is indicated in patients with a phenytoin concentration greater than 40 μg per mL, moderate neurologic toxicity, or rising levels after GI tract decontamination. As drug levels may continue to decline for many hours after stopping MDAC, such therapy should be discontinued before drug levels reach the therapeutic range in patients who require phenytoin for therapeutic purposes. Serum levels of concurrent anticonvulsant medications may also decline when MDAC is administered, increasing the risk of breakthrough seizures. An observation period is necessary to ensure establishment of a therapeutic anticonvulsant regimen and documentation of stable therapeutic serum levels even after passage of charcoal stools. Because phenytoin has a high degree of protein binding and hepatic elimination, forced diuresis, hemodialysis, and hemoperfusion are not useful [31]. It is anticipated that hemofiltration would not be useful for similar reasons.
Disposition
Because the majority of patients with phenytoin poisoning do well with supportive therapy alone, determining the degree of toxicity is important. After adequate GI decontamination, the patient should be assessed for progression of toxicity. Patients who are not suicidal or ataxic, have no underlying cardiac dysrhythmia, can feed themselves, and are not at risk of hurting themselves can be discharged, providing serum phenytoin levels are not rising and a reliable caretaker is available. Patients who do not meet these criteria should be admitted. Severely toxic patients, those with underlying cardiac or CNS disorder, intubated patients, or patients with rapidly progressive signs of toxicity require intensive care monitoring.
Valproic Acid
Valproic acid (VA) 2-propylpentanoic or 2-propyl valeric acid is structurally unique among the anticonvulsants. VA is a branched-chain carboxylic acid with a pKa of 4.8. In addition to being an anticonvulsant medication, VA is commonly used for the treatment of acute manic episodes, mood stabilization, and prophylaxis of migraine and affective disorders.
VA is marketed as a sodium salt (Depakene); in a syrup solution; in a prodrug form, divalproex sodium (Depakote); and as a sustained-release form of divalproex sodium (Depakote ER). The latter is a molecular complex that dissociates in the GI tract into two molecules of VA. There is also a parenteral form for VA.
Pharmacology
VA is thought to mediate its anticonvulsant effect by increasing cerebral and cerebellar levels of GABA [32] by blocking its metabolism through inhibition of GABA transferase and succinic aldehyde dehydrogenase. It may also prolong the recovery of inactivated sodium channels and have effects on potassium channels in neuronal cell membranes.
VA’s pharmacokinetic profile is significantly altered in an overdose setting. Within its therapeutic range (50 to 100 mg per mL), VA is 80% to 95% serum protein bound [33,34]. The degree of protein binding decreases and the Vd (0.13 to 0.22 L per kg) increases as VA levels exceed 90 μg per mL [33,34]. The resultant increase in free VA levels is evident by enhanced distribution into target organ systems and better than predicted extracorporeal drug removal. This has been demonstrated by
a higher cerebrospinal fluid-to-serum level and hemodialysis extraction ratio in the VA-poisoned patient [35,36]. Protein binding of VA may also be decreased in uremic patients or in the presence of other highly protein-bound agents (e.g., acetylsalicylic acid), which displace VA from its binding sites [37].
a higher cerebrospinal fluid-to-serum level and hemodialysis extraction ratio in the VA-poisoned patient [35,36]. Protein binding of VA may also be decreased in uremic patients or in the presence of other highly protein-bound agents (e.g., acetylsalicylic acid), which displace VA from its binding sites [37].
VA is highly bioavailable, with the time to peak serum levels after ingestion dependent on the dosage form and VA species. In capsule form, VA itself achieves peak serum levels after 1 to 4 hours in therapeutic dosing, whereas peak serum levels may be delayed 4 to 5 hours after ingestion of the enteric-coated divalproex sodium tablets. Peak serum levels may be delayed out to 17 hours in overdose [38]. This may be explained by the enteric-coating dissolution time and the sequential process of intestinal conversion of divalproex to the sodium salt. This is followed by the final conversion to the free acid, the only form absorbed from the GI tract. There is no evidence suggesting formation of pharmacobezoars from large numbers of VA tablets.
VA is metabolized predominantly by the liver, with 1% to 4% excreted unchanged in the urine [33]. It undergoes beta and omega oxidation to several metabolites: hydroxyvalproate, 2-propylglutarate, 2-propylpent-4-enoate, 5-hydroxyvalproate, and 4-hydroxyvalproate. At high doses of VA, the omega oxidation pathway may become saturated, leading to a decrease in total VA body clearance [35]. The metabolites undergo glucuronidation and biliary excretion, with a possible enterohepatic recirculation [35,39]. At therapeutic levels, VA elimination half-life averages 10.6 hours (range: 5 to 20 hours), but in an overdose it may extend to 30 hours.
VA disrupts amino acid and fatty acid metabolism, sequesters acetyl coenzyme A by forming valproyl coenzyme A, and interrupts the ornithine–citrulline shuttle and carnitine transport [40,41,42]. This may result in encephalopathy associated with hyperammonemia at therapeutic levels of VA [43,44], acutely contribute indirectly to the CNS-depressant effects, and chronically contribute to other target organ toxicity. VA metabolites have been implicated in the metabolic perturbations associated with VA poisoning [44,45], interfere with urine ketone determinations, and may be the hepatotoxic mediators of VA. There may be a link between VA- and opiate-induced CNS toxicity because of their similar influence on the GABAnergic systems [19,46]. Because VA and its metabolites are low molecular weight, branched chain carboxylic acids, they may be used as substrates for several enzymatic processes. This leads to inhibition of critical biochemical pathways, such as the urea cycle, and subsequent fatalities in some sensitive patient populations. Death has occurred after therapeutic doses of VA in patients with a congenital deficiency of ornithine carbamoyltransferase [47]. In addition, a frequently fatal Reye-like hepatitis has been observed in patients receiving therapeutic doses. Those at greatest risk appear to be very young patients (younger than 2 years of age), those being treated with multiple anticonvulsants, and those with other long-term neurologic complications. The fatality rate is 1 per 500 in this patient population [48]. This hepatotoxic reaction occurs in chronic exposure and may be mediated by metabolites formed via the cytochrome P450 pathway. These metabolites in turn depress fatty acid oxidation in the hepatocyte mitochondria [49]. This effect may parallel that seen after ingestion of ackee fruit containing hypoglycin, causing Jamaican vomiting sickness [49]. VA can produce a hyperammonemia and encephalopathy exclusive of the hepatotoxic reaction [39]. This may be associated with VA-induced carnitine deficiency [44].
Valproate as the sodium salt provides a significant sodium load (13.8 mg sodium per 100 mg VA) in overdose. VA and its metabolites are low-molecular-weight, osmotically active, free-acid, or anionic species. They may produce a slightly elevated osmolar gap and an elevated anion gap metabolic acidosis with a reduction in circulating endogenous cations, particularly calcium [35,40,49,50,51,52,53]. Valproate may have a dose-related toxic effect on bone marrow and platelet function, with resultant hematologic consequences such as thrombocytopenia, anemia, and leukopenia [54,55,56].
The morbidity and mortality from dose-related acute or acute-on-chronic VA poisoning appear to be related to hypoxic sequelae from respiratory failure, aspiration, or terminal cardiorespiratory arrest [35,50,51,52,57]. Although it has been speculated that VA has a direct, irreversible, neurotoxic effect, this has not been substantiated and it is indistinguishable from hypoxic injury [52].
Patients ingesting greater than 200 mg per kg are at high risk for significant CNS depression, but poor correlation exists between peak serum level and dose of VA ingested [57]. Patients who die from acute VA poisoning have had peak serum VA levels ranging from 106 to 2,728 μg per mL, whereas survival has been reported in a patient with a peak serum level of 2,120 μg per mL [40,49,56]. Although serum VA levels may not correlate with clinical effect, in general, serum levels of 180 μg per mL are usually associated with serious CNS toxicity (e.g., coma and apnea) and significant metabolic derangement (e.g., acidosis and hypocalcemia) [40,49,56,58,59]. The duration of toxicity is proportional to the peak serum VA level.
On the basis of endogenous VA clearance, it will take 3 days for the serum level to drop within the therapeutic range in a patient with a serum level greater than 1,000 μg per mL.
Clinical Manifestations
In acute intoxication, hypotension, mild tachycardia, decreased respiratory rate, and elevated or depressed temperature may be seen. Miosis may be present. The hallmarks of VA toxicity are global CNS-related depression in conjunction with unique metabolic changes. The mental status varies on a continuum from confusion and disorientation to obtundation and deep coma with respiratory failure. Tremor, hallucinations, and hyperactivity have been reported, but there is a notable lack of cerebellar–vestibular effects. Patients with an underlying seizure disorder may have breakthrough seizures. Most patients with serious acute VA poisoning manifest CNS toxicity for at least 24 hours and this may extend to several days. Laboratory abnormalities observed in patients with high serum VA levels include an anion gap metabolic acidosis, hypocalcemia, hyperosmolality, and hypernatremia. Transient rises in serum transaminase levels have been observed without evidence of functional liver toxicity. Hyperammonemia associated with vomiting, lethargy, and encephalopathy may occur at therapeutic serum levels. Although rare, complications or delayed sequelae associated with severe VA intoxication include optic nerve atrophy, cerebral edema, acute respiratory distress syndrome, and hemorrhagic pancreatitis.
Non–dose-related toxicity (e.g., hepatic failure, pancreatitis, red blood cell aplasia, neutropenia, and alopecia) has not been reported in acute overdoses with high serum levels of VA. Pancreatitis is usually considered a non–dose-related effect but has been observed [55]. Alopecia, thrombocytopenia, leukopenia, and anemia have been associated with acute and chronic VA intoxication.
The differential diagnosis should include opioid toxicity and a list of substances causing an increased anion gap metabolic acidosis. VA intoxication can be indistinguishable from opioid poisoning by signs and symptoms, and VA-poisoned patients may occasionally respond to naloxone. VA may cause a false-positive urine ketone determination, thereby misdirecting the clinician to causes of ketosis [40].
Diagnostic Evaluation
Essential laboratory studies should include sequential serum VA levels and levels of other anticonvulsant medications, particularly when the enteric-coated dosage form is involved. It should be recognized that VA metabolites are highly cross-reactive on enzyme-multiplied immunoassay technique assay for VA [35], and there may be an overestimation of serum VA levels as high as 50%. Recommended laboratory studies include complete blood cell count, reticulocyte count, serum electrolytes, blood urea nitrogen, creatinine, glucose, calcium, ammonia, and liver function tests. In addition, serum amylase and lipase levels should be obtained to rule out pancreatitis.
In all deliberate overdoses, an ECG and acetaminophen and salicylate levels should be obtained. Arterial blood gas, chest radiograph, head computed tomography, and lumbar puncture should be obtained as clinically indicated.
Management
As with any consequential CNS depressant ingestion, the patient’s airway and respiratory status should be frequently assessed; early intubation and ventilation help prevent hypoxic sequelae. Vascular access and continuous cardiac monitoring should be established. Patients with altered mental status should have a fingerstick blood sugar determination or receive IV dextrose, followed by naloxone and thiamine as clinically indicated.
Naloxone (0.8 to 2.0 mg) has been reported to be effective in increasing the level of consciousness of patients with signs and symptoms of opioid toxicity and serum VA levels between 185 and 190 μg per mL [58,59]. Patients with higher VA serum levels have not responded to larger doses of naloxone [56,60]. Naloxone has been shown experimentally to antagonize GABA, the inhibitory neurotransmitter increased by VA [19,46]. It is therefore worth trying naloxone (up to 10 mg) all comatose patients with suspected VA poisoning. Flumazenil, the benzodiazepine antagonist, should be avoided in patients with a preexisting seizure disorder.
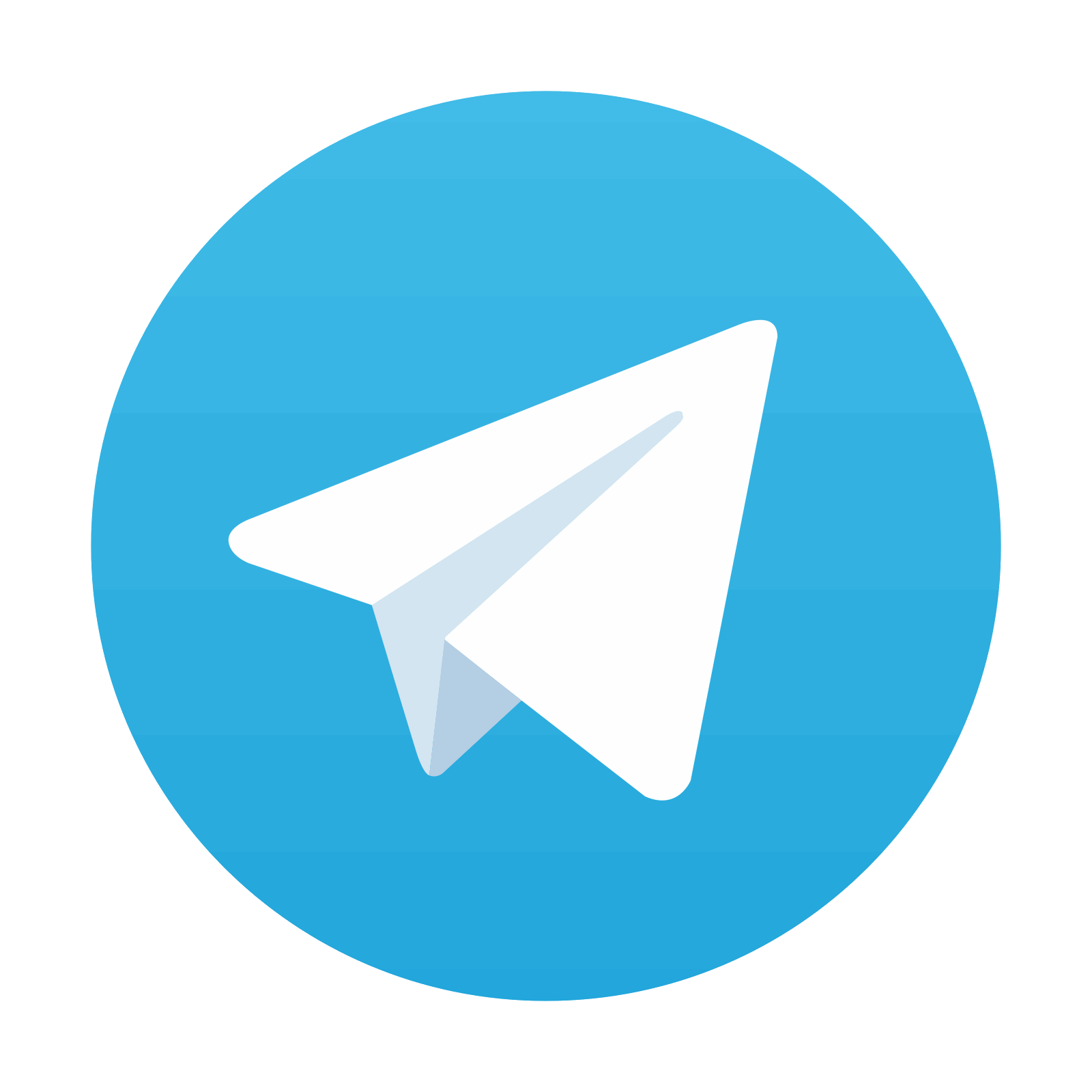
Stay updated, free articles. Join our Telegram channel

Full access? Get Clinical Tree
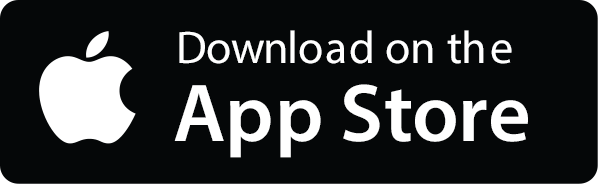
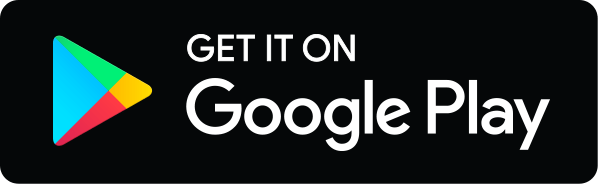