The effects of cardiac antiarrhythmic drugs on the action potential and effective refractory period of the cardiac action potential determine the clinical effect of these drugs. Drugs that primarily block inward sodium ion flow will slow conduction and result in suppression of the maximum upstroke velocity (Vmax) of the cardiac action potential. Potassium channel blocking drugs prolong repolarization by increasing the duration of the cardiac action potential and the effective refractory period resulting in prolongation of the QTc interval on the electrocardiogram (ECG). Calcium channels are present in myocardial cells, and the α subunit of L and T calcium ion channels is the site of action of some cardiac antiarrhythmic drugs.
Classification
Cardiac arrhythmic drugs are most commonly classified into four groups based primarily on the ability of the drug to control arrhythmias by blocking specific ion channels and currents during the cardiac action potential (Tables 21-1 and 21-2).8,9 Few cardiac antiarrhythmic drugs demonstrate completely specific effects on cardiac ion channels. Other characteristics including the impact of the drug on autonomic nervous system activity and myocardial contractility may be more important clinically. Antiarrhythmic drugs also differ in their pharmacokinetics and efficacy in treating specific types of arrhythmias (Tables 21-3 and 21-4).7




Class I Drugs
Class I drugs inhibit fast sodium channels during depolarization (phase 0) of the cardiac action potential with resultant decreases in depolarization rate and conduction velocity (see Fig. 21-1).8
Class IA Drugs
Class IA drugs (quinidine, procainamide, disopyramide, moricizine) lengthen both the action potential duration and the effective refractory period reflecting sodium channel inhibition and prolonged repolarization owing to potassium channel blockade.
Class IB Drugs
Class IB drugs (lidocaine, mexiletine, tocainide, phenytoin) are less powerful sodium channel blockers and, unlike class IA drugs, shorten the action potential duration and refractory period in normal cardiac ventricular muscle. In ischemic tissue, lidocaine may also block adenosine triphosphate (ATP)–dependent channels, thus preventing ischemia-mediated shortening of ventricular depolarization.
Class IC Drugs
Class IC drugs (flecainide, propafenone) are potent sodium channel blockers and markedly decrease the rate of phase 0 depolarization and speed of conduction of cardiac impulses. These drugs have little effect on the duration of the cardiac action potential and the effective refractory period in ventricular myocardial cells but do shorten the duration of the action potential in Purkinje fibers. This inhomogeneity of effects on the rate of cardiac depolarization plus the slowing of cardiac conduction may contribute to the proarrhythmic effects of these drugs.
Class II Drugs
Class II drugs are β-adrenergic antagonists. β-Adrenergic antagonists decrease the rate of spontaneous phase 4 depolarization resulting in decreased autonomic nervous system activity, which may be important in suppression of ventricular arrhythmia during myocardial ischemia and reperfusion. Drug-induced slowing of heart rate with resulting decreases in myocardial oxygen requirements is desirable in patients with coronary artery disease. β-Adrenergic antagonists slow the speed of conduction of cardiac impulses through atrial tissues resulting in prolongation of the P-R interval on the ECG, whereas the duration of action of the cardiac action potential in ventricular myocardium is not altered. These drugs are effective in decreasing the incidence of arrhythmia-related morbidity and mortality although the exact mechanism for this beneficial effect remains unclear.
Class III Drugs
Class III drugs (amiodarone, sotalol, bretylium) block potassium ion channels resulting in prolongation of cardiac depolarization, action potential duration, and the effective refractory period. These effects are beneficial in preventing cardiac arrhythmias by decreasing the proportion of the cardiac cycle during which myocardial cells are excitable and thus susceptible to a triggering event. Reentrant tachycardias may be suppressed if the action potential duration becomes longer than the cycle length of the tachycardia circuit.
In addition to class III effects, amiodarone exhibits sodium channel blockade (class I), β blockade (class II), and calcium channel blockade (class IV). Although this drug is U.S. Food and Drug Administration (FDA) approved for the treatment of refractory ventricular arrhythmias, it has become a widely used drug for the acute treatment and prevention of supraventricular and ventricular arrhythmias both in the operating room and the intensive care unit (see the following texts).
Sotalol is a long-acting, noncardioselective β-blocking drug consisting of a racemic mixture of levorotatory (L) and dextrorotatory (D) isomers that possess similar class III effects. The L isomer of sotalol acts as a β-adrenergic antagonist, whereas the D isomer may increase mortality in patients with ventricular dysfunction and recent myocardial infarction. The reduced incidence of proarrhythmia effects seen with amiodarone or racemic sotalol treatment may be related to beneficial class II effects.
Class IV Drugs
Class IV drugs are the calcium blockers verapamil and diltiazem, which act by inhibiting inward slow calcium ion currents that may contribute to the development of tachycardias. As such, these drugs may be useful in the treatment of both supraventricular tachyarrhythmias and idiopathic ventricular tachycardia. The dihydropyridine calcium blockers (nifedipine, nicardipine, nimodipine) do not have antiarrhythmic action.
Proarrhythmic Effects
Proarrhythmia effects describe bradyarrhythmias or tachyarrhythmias that represent new cardiac arrhythmias associated with antiarrhythmic drug treatment.3 These include torsades de pointes (most common), incessant ventricular tachycardia, and wide complex ventricular rhythm.8
Torsades de Pointes
Torsades de pointes is triggered by early afterdepolarizations in a setting of delayed repolarization and increased duration of refractoriness manifesting as prolongation of the QTc interval on the ECG. Class IA (quinidine and disopyramide) and class III drugs (amiodarone) prolong the QTc interval by potassium channel blockade providing the setting for torsades de pointes. Drug-induced torsades de pointes is often associated with bradycardia because the QTc interval is longer at slower heart rates. Exacerbating factors such as hypokalemia, hypomagnesemia, poor left ventricular function, and concomitant administration of other QT-prolonging drugs are important predisposing factors in the development of this life-threatening rhythm.
Incessant Ventricular Tachycardia
Incessant ventricular tachycardia may be precipitated by drugs that slow conduction of cardiac impulses (class IA and class IC drugs) sufficiently to create a continuous ventricular tachycardia circuit (reentry). Incessant ventricular tachycardia is more likely to occur with high doses of class IC drugs and in patients with a prior history of sustained ventricular tachycardia and poor left ventricular function. Ventricular tachycardia due to this mechanism is generally slower because of the drug effect but may be resistant to drugs or electrical therapy. This rhythm is rarely associated with class IB drugs, which have a weaker blocking effect of sodium channels.
Wide Complex Ventricular Rhythm
Wide complex ventricular rhythm is usually associated with class IC drugs in the setting of structural heart disease. Excessive plasma concentrations of the drug or an abrupt change in the dose may result in this arrhythmia. Wide complex ventricular rhythm is thought to reflect a reentrant tachycardia and easily degenerates to ventricular fibrillation.
Efficacy and Results of Treatment with Cardiac Antiarrhythmic Drugs
Chronic suppression of ventricular ectopy with an antiarrhythmic drug other than amiodarone does not prevent future life-threatening arrhythmias and may increase mortality.8 In fact, patients treated with class IC drugs experienced a higher incidence of sudden cardiac arrest reflecting the proarrhythmia effects of these drugs. Conversely, β-adrenergic antagonists that do not typically suppress ventricular arrhythmias appear to decrease mortality and the risk of life-threatening ventricular arrhythmias. In patients with a history of myocardial infarction and ventricular arrhythmias, mortality was increased in those who received class IA and class IC drugs, whereas mortality was decreased with amiodarone and β-adrenergic antagonists.10 Survivors of cardiac arrest have a high risk of subsequent ventricular fibrillation and treatment of these patients with amiodarone results in fewer life-threatening cardiac events. The proarrhythmic and negative inotropic effects of class IA and class IC drugs precludes their administration to patients with congestive heart failure. In these patients, administration of amiodarone appears to be safe and effective.
Prophylactic Antiarrhythmic Drug Therapy
Although commonly used in the past, lidocaine is no longer recommended as prophylactic treatment for patients in the early stages of acute myocardial infarction and without malignant ventricular ectopy.11 In fact, lidocaine does not decrease and may increase mortality because of an increase in the occurrence of fatal bradyarrhythmias and asystole.
Calcium channel antagonists are not recommended as routine treatment of patients with acute myocardial infarction because mortality is not decreased by these drugs. Calcium channel blockers may be administered to patients in whom myocardial ischemia persists despite treatment with aspirin, heparin, nitroglycerin, and β-adrenergic antagonists.
Magnesium is involved in many enzymatic reactions, produces systemic and coronary vasodilation, inhibits platelet aggregation, and decreases myocardial reperfusion injury. Data on the ability of magnesium to decrease mortality following myocardial infarction are conflicting.12 Treatment with magnesium is indicated in patients following an acute myocardial infarction who develop torsades de pointes ventricular tachycardia.13
In patients with heart failure, amiodarone reduces the risk of sudden cardiac death by 29% and therefore represents a viable alternative in patients who are not eligible for or who do not have access to implanted cardiac defibrillator (ICD) therapy for the prevention of sudden cardiac death from arrhythmias.14 Amiodarone can be considered as an adjuvant therapy to ICD in preventing recurrent shocks. However, amiodarone therapy is neutral with respect to all-cause mortality and is associated with a two- and fivefold increased risk of pulmonary and thyroid toxicity respectively.14 Prophylactic dofetilide and azimilide did not demonstrate a mortality benefit either.15 In summary, there is little role for prophylactic antiarrhythmic medications for the primary prevention of sudden cardiac death in patients with heart failure with the exception of amiodarone.
Atrial fibrillation after heart surgery is a common complication that has been associated with prolonged hospitalization and cardiovascular morbidity. Prophylactic therapy with amiodarone, β blockers, sotalol, and magnesium has been effective in reducing the occurrence of atrial fibrillation, length of hospital stay, and cost of hospital treatment and may be effective in reducing the risk of stroke.16
Decision to Treat Cardiac Arrhythmias
Drug treatment of cardiac arrhythmias is not uniformly effective and frequently causes side effects (see the section “Proarrhythmic Effects”).1,17 The benefit of antiarrhythmic drugs is clearest when it results in the immediate termination of a sustained tachycardia. There is no doubt that the termination of ventricular tachycardia by lidocaine or supraventricular tachycardia by adenosine or verapamil is a true benefit of antiarrhythmic therapy. Furthermore, when given for a limited period, side effects are less likely. Conversely, it has been difficult to demonstrate that antiarrhythmic drugs alleviate symptoms related to chronic cardiac arrhythmias, a situation in which the risk of side effects is greater. The increase in long-term mortality associated with certain drugs (Cardiac Arrhythmia Suppression Trial [CAST] and other trials) raises the possibility that some antiarrhythmics result in sensitization of the myocardium to concurrent triggering factors (myocardial ischemia, neurohumoral activation, myocardial stretch, slow healing process after a myocardial infarction) that then elicit cardiac arrhythmias.17 The mechanism by which β-adrenergic antagonists decrease mortality after an acute myocardial infarction is not known.
The value of monitoring plasma drug concentrations in minimizing the risks associated with therapy is not established. In fact, many side effects appear to depend as much on the nature and extent of the underlying heart disease as on increased plasma drug concentrations.17
Antiarrhythmic Drug Pharmacology
Quinidine
Quinidine is a class IA drug that is effective in the treatment of acute and chronic supraventricular arrhythmias (Fig. 21-2).18 Due to its side effect profile and low therapeutic index (see the following texts), and the availability of newer agents, quinidine is rarely used. It can prevent recurrence of supraventricular tachyarrhythmias or suppress premature ventricular contractions and can slow the ventricular rate in the presence of atrial fibrillation, and about 25% of patients with new-onset atrial fibrillation will convert to normal sinus rhythm when treated with quinidine. Supraventricular tachyarrhythmias associated with Wolff-Parkinson-White syndrome are effectively suppressed by quinidine.

Quinidine is most often administered orally in a dose of 200 to 400 mg four times daily. Oral absorption of quinidine is rapid, with peak concentrations in the plasma attained in 60 to 90 minutes and an elimination half-time of 5 to 12 hours. The therapeutic blood level of quinidine is 1.2 to 4.0 µg/mL. Intravenous (IV) quinidine is rarely used due to vasodilation and myocardial depression.
Mechanism of Action
Quinidine is the dextroisomer of quinine and, like quinine, has antimalarial and antipyretic effects. Unlike quinine, however, quinidine has intense effects on the heart. For example, quinidine decreases the slope of phase 4 depolarization, which explains its effectiveness in suppressing cardiac arrhythmias caused by enhanced automaticity. Quinidine increases the fibrillation threshold in the atria and ventricles. Quinidine-induced slowing of the conduction of cardiac impulses through normal and abnormal fibers may be responsible for the ability of quinidine to occasionally convert atrial flutter or fibrillation to normal sinus rhythm. This drug can abolish reentry arrhythmias by prolonging conduction of cardiac impulses in an area of injury, thus converting one-way conduction blockade to two-way conduction blockade. A decrease in the atrial rate during atrial flutter or fibrillation may reflect slowed conduction velocity, a prolonged effective refractory period in the atria, or both.
Metabolism and Excretion
Quinidine is hydroxylated in the liver to inactive metabolites, which are excreted in the urine. About 20% of quinidine is excreted unchanged in the urine. Enzyme induction significantly shortens the duration of action of quinidine. The concurrent administration of phenytoin, phenobarbital, or rifampin may lower blood levels of quinidine by enhancing liver clearance. Because of its dependence on renal excretion and hepatic metabolism for clearance from the body, accumulation of quinidine or its metabolites may occur in the presence of impaired function of these organs. About 80% to 90% of quinidine in plasma is bound to albumin. Quinidine accumulates rapidly in most tissues except the brain.
Side Effects
Quinidine has a low therapeutic ratio, with heart block, hypotension, and proarrhythmia being potential adverse side effects. As the plasma concentration increases to more than 2 µg/mL, the P-R interval, QRS complex, and QTc interval on the ECG are prolonged. Patients with preexisting prolongation of the QTc interval or evidence of atrioventricular heart block on the ECG should not be treated with quinidine.
Patients in normal sinus rhythm treated with quinidine may show an increase in heart rate that is a result of presumably either an anticholinergic action and/or a reflex increase in sympathetic nervous system activity. This atropine-like action of quinidine opposes its direct depressant actions on the sinoatrial and atrioventricular nodes and is why digitalis is often given before quinidine therapy is initiated.
Allergic reactions may include drug rash or a drug fever that is occasionally associated with leukocytosis. Thrombocytopenia is a rare occurrence that is caused by drug–platelet complexes that evoke production of antibodies. Discontinuation of quinidine results in return of the platelet count to normal in 2 to 7 days. Nausea, vomiting, and diarrhea occur in about one-third of treated patients.
Like other cinchona alkaloids and salicylates, quinidine can cause cinchonism. Symptoms of cinchonism include tinnitus, decreased hearing acuity, blurring of vision, and gastrointestinal upset. In severe cases, there may be abdominal pain and mental confusion.
Because quinidine is an α-adrenergic blocking drug, it can interact in an additive manner with drugs that cause vasodilation. Quinidine also interferes with normal neuromuscular transmission and may accentuate the effect of neuromuscular blockings drugs. Recurrence of skeletal muscle paralysis in the immediate postoperative period has been observed in association with the administration of quinidine.19
Procainamide
Procainamide is as effective as quinidine for the treatment of ventricular tachyarrhythmias but less effective in abolishing atrial tachyarrhythmias (Fig. 21-3). Premature ventricular contractions and paroxysmal ventricular tachycardia are suppressed in most patients within a few minutes after IV administration, which is better tolerated than IV quinidine but may still cause hypotension. Procainamide can be administered IV at a rate not exceeding 100 mg every 5 minutes until the rhythm is controlled (maximum 15 mg/kg). When the cardiac arrhythmia is controlled, a constant rate of infusion (2 to 6 mg per minute) is used to maintain a therapeutic concentration of procainamide. The systemic blood pressure and ECG (QRS complex) are monitored continuously during infusion of this drug. The therapeutic blood level of procainamide is 4 to 8 µg/mL.

Mechanism of Action
Procainamide is an analogue of the local anesthetic procaine. Procainamide possesses an electrophysiologic action similar to that of quinidine but produces less prolongation of the QTc interval on the ECG. As a result, paradoxical ventricular tachycardia is a rare feature of procainamide therapy. Procainamide has no vagolytic effect and can be used in patients with atrial fibrillation to suppress ventricular irritability without increasing the ventricular rate. Like quinidine, procainamide may prolong the QRS complex and cause ST-T wave changes on the ECG.
Metabolism and Excretion
Procainamide is eliminated by renal excretion and hepatic metabolism. In humans, 40% to 60% of procainamide is excreted unchanged by the kidneys. The dose of procainamide must be decreased when renal function is abnormal. In the liver, procainamide that has not been excreted unchanged by the kidneys is acetylated to N-acetyl procainamide (NAPA), which is also eliminated by the kidneys. This metabolite is cardioactive and probably contributes to the antiarrhythmic effects of procainamide. In the presence of renal failure, plasma concentrations of NAPA may reach dangerous levels. Eventually, 90% of an administered dose of procainamide is recovered as unchanged drug or its metabolites.
The activity of the N-acetyltransferase enzyme response for the acetylation of procainamide is genetically determined. In patients who are rapid acetylators, the elimination half-time of procainamide is 2.5 hours compared with 5 hours in slow acetylators. The blood level of NAPA exceeds that of procainamide in rapid but not slow acetylators. Unlike its analogue, procaine, procainamide is highly resistant to hydrolysis by plasma cholinesterase. Evidence of this resistance is the fact that only 2% to 10% of an administered dose of procainamide is recovered unchanged in the urine as paraaminobenzoic acid.
Only about 15% of procainamide is bound to plasma proteins. Despite this limited binding in plasma, procainamide is avidly bound to tissue proteins with the exception of the brain.
Side Effects
Similar to quinidine, use of procainamide has dramatically decreased due to its side effect profile and availability of newer agents. Hypotension that results from procainamide is more likely to be caused by direct myocardial depression than peripheral vasodilation. Indeed, rapid IV injection of procainamide is associated with hypotension, whereas higher plasma concentrations slow conduction of cardiac impulses through the atrioventricular node and intraventricular conduction system. Ventricular asystole or fibrillation may occur when procainamide is administered in the presence of heart block, as associated with digitalis toxicity. Direct myocardial depression that occurs at high plasma concentrations of procainamide is exaggerated by hyperkalemia. As with quinidine, ventricular arrhythmias may accompany excessive plasma concentrations of procainamide.
Chronic administration of procainamide may be associated with a syndrome that resembles systemic lupus erythematosus. Serositis, arthritis, pleurisy, or pericarditis may develop, but unlike systemic lupus erythematosus, vasculitis is not usually present. Patients with this lupus-like syndrome often develop antinuclear antibodies (positive antinuclear antibody test). Slow acetylators are more likely than rapid acetylators to develop antinuclear antibodies. Symptoms disappear when procainamide is discontinued.
As with many drugs, procainamide may cause drug fever or an allergic rash. Although agranulocytosis is rare, leukopenia and thrombocytopenia may be seen after chronic use of procainamide, often in association with the lupus-like syndrome. The most common early, noncardiac complications of procainamide are gastrointestinal disturbances, including nausea and vomiting.
Disopyramide
Disopyramide is comparable to quinidine in effectively suppressing atrial and ventricular tachyarrhythmias (Fig. 21-4). Absorption of oral disopyramide is almost complete, resulting in peak blood levels within 2 hours of administration. Therapeutic plasma concentrations of disopyramide are 2 to 4 µg/mL. About 50% of the drug is excreted unchanged by the kidneys. As a result, the typical elimination half-time of 8 to 12 hours is prolonged in the presence of renal dysfunction. A dealkylated metabolite with less antiarrhythmic and atropine-like activity than the parent drug accounts for about 20% of the drug’s elimination. Disopyramide is not available in an IV formulation.

Side Effects
The most common side effects of disopyramide are dry mouth and urinary hesitancy, both of which are caused by the drug’s anticholinergic activity. Some patients taking disopyramide also experience blurred vision or nausea. Prolongation of the QTc interval on the ECG and paradoxical ventricular tachycardia (similar to quinidine) may occur. For this reason, disopyramide should be administered cautiously if patients have known cardiac conduction effects. Disopyramide has significant myocardial depressant effects and can precipitate congestive heart failure and hypotension. The potential for direct myocardial depression, especially in patients with preexisting left ventricular dysfunction, seems to be greater with this drug than with quinidine and procainamide.
Moricizine
Moricizine is a phenothiazine derivative with modest efficacy in the treatment of sustained ventricular arrhythmias. In view of its proarrhythmic effects, this drug is reserved for the treatment of life-threatening ventricular arrhythmias when other drugs such as amiodarone are not available or contraindicated (e.g., allergy). It is not effective in the treatment of atrial arrhythmias. Moricizine decreases the fast inward sodium ion current and also decreases automaticity.
Side Effects
Proarrhythmic effects occur in 3% to 15% of patients treated chronically with moricizine. Patients with poor left ventricular function tolerate moricizine and small increases in systemic blood pressure and heart rate may accompany therapy. Plasma concentrations of theophylline may increase in patients treated with moricizine.
Lidocaine
Lidocaine is used principally for suppression of ventricular arrhythmias, having minimal if any effect on supraventricular tachyarrhythmias (see Chapter 10). This drug is particularly effective in suppressing reentry cardiac arrhythmias, such as premature ventricular contractions and ventricular tachycardia. The efficacy of prophylactic lidocaine therapy for preventing early ventricular fibrillation after acute myocardial infarction has not been documented and is no longer recommended (see earlier discussion).
In adult patients with a normal cardiac output, hepatic function, and hepatic blood flow, an initial administration of lidocaine, 2 mg/kg IV, followed by a continuous infusion of 1 to 4 mg per minute should provide therapeutic plasma lidocaine concentrations of 1 to 5 µg/mL. Decreased cardiac output and/or hepatic blood flow, as produced by anesthesia, acute myocardial infarction, or congestive heart failure, may decrease by 50% or more of the initial dose and the rate of lidocaine infusion necessary to maintain therapeutic plasma levels. Concomitant administration of drugs such as propranolol and cimetidine can result in decreased hepatic clearance of lidocaine. Advantages of lidocaine over quinidine or procainamide are the more rapid onset and prompt disappearance of effects when the continuous infusion is terminated, greater therapeutic index, and a much reduced side effect profile. Lidocaine for IV administration differs from that used for local anesthesia because it does not contain a preservative. Lidocaine is also well absorbed after oral administration but is subject to extensive hepatic first-pass metabolism. As a result, only about one-third of an oral dose of lidocaine reaches the circulation. Mexiletine (see the following texts) is an oral analogue of lidocaine. Intramuscular (IM) absorption of lidocaine is nearly complete. In an emergency situation, lidocaine, 4 to 5 mg/kg IM, will produce a therapeutic plasma concentration in about 15 minutes. This level is maintained for about 90 minutes.
Mechanism of Action
Lidocaine delays the rate of spontaneous phase 4 depolarization by preventing or diminishing the gradual decrease in potassium ion permeability that normally occurs during this phase. The effectiveness of lidocaine in suppressing premature ventricular contractions reflects its ability to decrease the rate of spontaneous phase 4 depolarization. The ineffectiveness of lidocaine against supraventricular tachyarrhythmias presumably reflects its inability to alter the rate of spontaneous phase 4 depolarization in atrial cardiac cells.
In usual therapeutic doses, lidocaine has no significant effect on either the QRS or QTc interval on the ECG or on atrioventricular conduction. In high doses, however, lidocaine can decrease conduction in the atrioventricular node as well as in the His–Purkinje system.
Metabolism and Excretion
Lidocaine is metabolized in the liver, and resulting metabolites may possess cardiac antiarrhythmic activity.
Side Effects
Lidocaine is essentially devoid of effects on the ECG or cardiovascular system when the plasma concentration remains less than 5 µg/mL. In contrast to quinidine and procainamide, lidocaine does not alter the duration of the QRS complex on the ECG, and activity of the sympathetic nervous system is not changed. Lidocaine depresses cardiac contractility less than any other antiarrhythmic drug used to suppress ventricular arrhythmias. Toxic plasma concentrations of lidocaine (>5 to 10 µg/mL) produce peripheral vasodilation and direct myocardial depression, resulting in hypotension. In addition, slowing of conduction of cardiac impulses may manifest as bradycardia, a prolonged P-R interval, and widened QRS complex on the ECG. Stimulation of the central nervous system (CNS) occurs in a dose-related manner, with symptoms appearing when plasma concentrations of lidocaine are greater than 5 µg/mL. Seizures are possible at plasma concentrations of 5 to 10 µg/mL. CNS depression, apnea, and cardiac arrest are possible when plasma lidocaine concentrations are greater than 10 µg/mL. The convulsive threshold for lidocaine is decreased during arterial hypoxemia, hyperkalemia, or acidosis, emphasizing the importance of monitoring these parameters during continuous infusion of lidocaine to patients for suppression of ventricular arrhythmias.
Mexiletine
Mexiletine is an orally effective amine analogue of lidocaine that is used for the chronic suppression of ventricular cardiac tachyarrhythmias. Combination with a β blocker or another antiarrhythmic drug such as quinidine or procainamide results in a synergistic effect that permits a decrease in the dose of mexiletine and an associated decrease in the incidence of side effects. Electrophysiologically, mexiletine is similar to lidocaine. The addition of the amine side group enables mexiletine to avoid significant hepatic first-pass metabolism that limits the effectiveness of orally administered lidocaine. The usual adult dose is 150 to 200 mg every 8 hours. As it is a lidocaine analog, mexiletine may be effective in decreasing neuropathic pain for patients in whom alternative pain medications have been unsatisfactory.20
Side Effects
Epigastric burning may occur and is often relieved by taking the drug with meals. Neurologic side effects include tremulousness, diplopia, vertigo, and occasionally slurred speech. Cardiovascular side effects resemble lidocaine. Increases in liver enzymes may occur especially in patients manifesting congestive heart failure. Blood dyscrasias occur rarely. Proarrhythmic effects may manifest in occasionally treated patients. Toxic effects may develop at plasma concentrations only slightly above therapeutic levels.
Tocainide
Tocainide, like mexiletine, is an orally effective amine analogue of lidocaine that was formerly used for the chronic suppression of ventricular cardiac tachyarrhythmias, but is no longer available in the United States. Its side effects resemble those of mexiletine, but in rare patients, this drug has caused severe bone marrow depression (leukopenia, anemia, thrombocytopenia) and pulmonary fibrosis.20 The usual adult dose is 400 to 800 mg administered every 8 hours. As with mexiletine, the combination of tocainide with a β-adrenergic blocker or another antiarrhythmic drug has a synergistic effect.
Phenytoin
Phenytoin is particularly effective in suppression of ventricular arrhythmias associated with digitalis toxicity. This drug is effective, although to a lesser extent than quinidine, procainamide, and lidocaine, in the treatment of ventricular arrhythmias due to other causes. Phenytoin may be useful in the treatment of paradoxical ventricular tachycardia or torsades de pointes that is associated with a prolonged QTc interval on the ECG. Treatment of atrial tachyarrhythmias with phenytoin is not very effective.
Phenytoin can be administered orally or IV. Intramuscular administration is too unreliable to treat cardiac arrhythmias. The IV dose is 100 mg (1.5 mg/kg) every 5 minutes until the cardiac arrhythmia is controlled or 10 to 15 mg/kg (maximum 1,000 mg) has been administered. Because phenytoin can precipitate in 5% dextrose in water, it is preferable to give the drug via a delivery tubing containing normal saline. Slow IV injection into a large peripheral or central vein is recommended to minimize the likelihood of discomfort or thrombosis at the injection site. Therapeutic blood levels range from 10 to 18 µg/mL.
Mechanism of Action
The effects of phenytoin on automaticity and velocity of conduction of cardiac impulses resemble those of lidocaine. Phenytoin exerts a greater effect on the electrocardiographic QTc interval than does lidocaine and shortens the QTc interval more than any of the other antiarrhythmic drugs. Phenytoin has no significant effect on the ST-T waves or the QRS complex. It does not significantly depress the myocardium in usual doses but can cause hypotension when administered in high doses rapidly. Conduction of cardiac impulses through the atrioventricular node is improved, but activity of the sinus node may be depressed. The ability of some volatile anesthetics to depress the sinoatrial node is a consideration if administration of phenytoin during general anesthesia is planned.
Metabolism and Excretion
Phenytoin is hydroxylated and then conjugated with glucuronic acid for excretion in the urine. The elimination half-time is about 24 hours. Because phenytoin is metabolized by the liver, impaired hepatic function may result in higher than normal blood levels of the drug. Blood levels of phenytoin can be lowered by drugs, such as barbiturates, that enhance its rate of metabolism. Warfarin, phenylbutazone, and isoniazid may inhibit metabolism and increase phenytoin blood levels. Uremia increases the unbound fraction of phenytoin relative to the plasma-bound portion.
Side Effects
Phenytoin toxicity most commonly manifests as CNS disturbances, especially cerebellar disturbances. Symptoms include ataxia, nystagmus, vertigo, slurred speech, sedation, and mental confusion. Cerebellar symptoms correlate with phenytoin blood levels of greater than 18 µg/mL. Cardiac arrhythmias that have not been suppressed at this concentration are unlikely to respond favorably to further increases in the dosage of phenytoin. Phenytoin partially inhibits insulin secretion and may lead to increased blood glucose levels in patients who are hyperglycemic. Leukopenia, granulocytopenia, and thrombocytopenia may occur as a manifestation of drug-induced bone marrow depression. Nausea, skin rash, and megaloblastic anemia may occur.
Flecainide
Flecainide is a fluorinated local anesthetic analogue of procainamide that is more effective in suppressing ventricular premature beats and ventricular tachycardia than quinidine and disopyramide (Fig. 21-5). Flecainide is also effective for the treatment of atrial tachyarrhythmias. Because it delays conduction in the bypass tracts, flecainide can be effective for the treatment of tachyarrhythmias due to reentry mechanisms as associated with the Wolff-Parkinson-White syndrome. Chronic treatment of ventricular arrhythmias with flecainide after myocardial infarction is not recommended due to an increased incidence of sudden death in treated patients.21 Thus, flecainide should be reserved for the treatment of life-threatening arrhythmias.

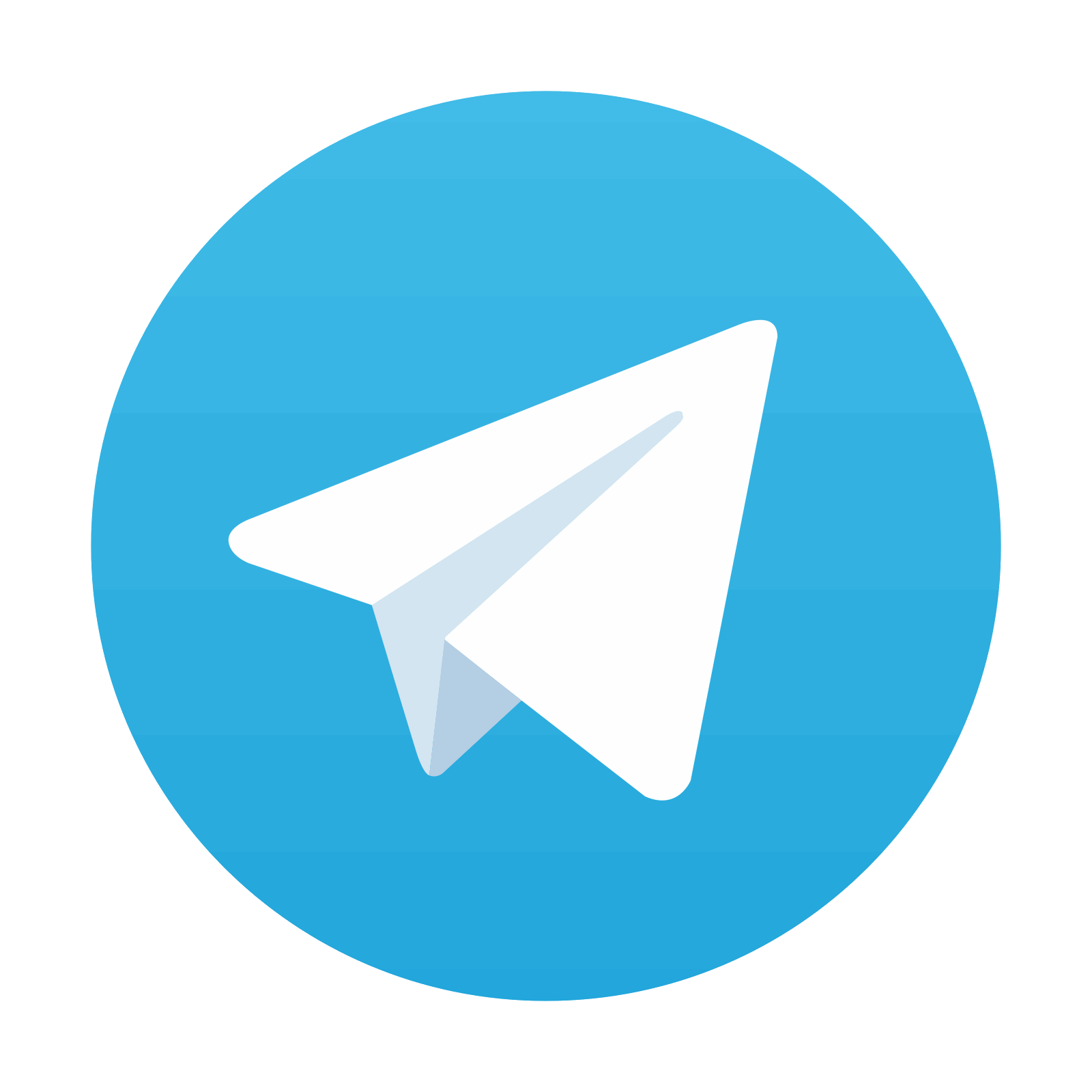
Stay updated, free articles. Join our Telegram channel

Full access? Get Clinical Tree
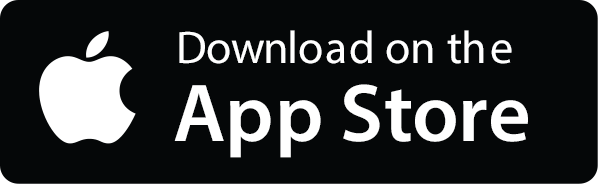
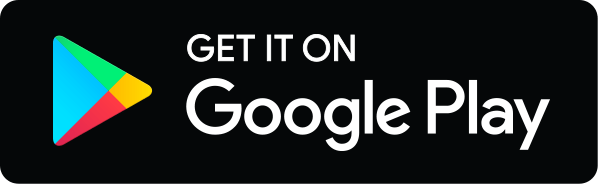