Antiarrhythmic Agents
Michael Ganetsky
The therapeutic use, misuse, and intentional overdose of antiarrhythmic drugs are associated with severe morbidity and mortality [1]. The recognition, management, and prevention of antiarrhythmic toxicity require an understanding of the pharmacology of these drugs as they are related to cardiac electrophysiology. A general review of the mechanisms involved as well as the principles of management of poisoning is followed by a discussion of individual agents.
Pharmacology
Antiarrhythmic drugs are most commonly classified on the basis of their predominant physiologic effect and mechanism of action as originally proposed by Vaughan Williams [2] and Campbell [3] (Tables 120.1 and 120.2; Fig. 120.1).
The major effect of class I agents is blockade of the fast inward sodium current responsible for the rapid upstroke and conduction of the action potential [4] (see Fig. 120.1). This effect is also known as local anesthetic or membrane stabilizing action. Class I drugs depress automaticity, particularly in Purkinje fibers. Class I drugs comprise a large group of antiarrhythmic agents, many of which have diverse electrophysiologic properties; consequently, this class has been subdivided into classes IA, IB, and IC (see Table 120.2) [3].
The class II antiarrhythmic drugs are β-adrenergic antagonists; they inhibit the proarrhythmic effects of catecholamines, which shorten refractory periods and facilitate reentrant circuits. The slowly conducting, calcium-channel–dependent action potentials of the normal sinoatrial (SA) and atrioventricular (AV) nodes (see Fig. 120.1) rely partially on sympathetic tone. Class II drugs depress conduction and automaticity through these specialized tissues, leading to bradycardia and AV block. Toxicity due to beta-blockers is covered further in Chapter 125.
Class III agents prolong the refractory period by increasing the cardiac action potential duration (APD), especially in phases 2 and 3 (see Fig. 120.1). This effect is produced by blockade of the major outward potassium-rectifying (repolarizing) current. Amiodarone is the prototypic class III agent, whereas ibutilide and dofetilide are newer class III agents.
Table 120.1 Vaughan Williams Classification of Antiarrhythmic Actions | |||||||||||||||||||||||||||||||||||||||||||||||||||
---|---|---|---|---|---|---|---|---|---|---|---|---|---|---|---|---|---|---|---|---|---|---|---|---|---|---|---|---|---|---|---|---|---|---|---|---|---|---|---|---|---|---|---|---|---|---|---|---|---|---|---|
|
Class IV drugs (calcium antagonists or calcium channel blockers) antagonize the slow inward calcium current responsible for the slow upstroke and conduction of the action potentials of SA and AV nodal cells [4] (see Fig. 120.1). Verapamil, diltiazem, and nifedipine represent the three subclasses of calcium channel antagonists. Both verapamil and diltiazem have negative inotropic and chronotropic properties and are useful for slowing the ventricular response rate in patients with atrial
fibrillation. In therapeutic dosing, calcium channel antagonists such as nifedipine have little effect on cardiac conduction or inotropic state and are, therefore, not used for their antiarrhythmic properties. Calcium channel blocker toxicity is discussed in Chapter 126.
fibrillation. In therapeutic dosing, calcium channel antagonists such as nifedipine have little effect on cardiac conduction or inotropic state and are, therefore, not used for their antiarrhythmic properties. Calcium channel blocker toxicity is discussed in Chapter 126.
Table 120.2 Subgroups of Class I Drugs | ||||||||||||||||
---|---|---|---|---|---|---|---|---|---|---|---|---|---|---|---|---|
|
Adenosine and digoxin are two drugs with antiarrhythmic effects that do not fall within the Vaughan Williams classification. Adenosine is an endogenous nucleoside that produces AV nodal conduction block and vasodilation via specific adenosine-sensitive receptors. The antiarrhythmic properties and toxicity of digoxin are discussed in Chapter 127.
The cellular electropharmacology of antiarrhythmic agents involves suppression of automaticity, decreased cardiac conduction, and refractory period prolongation. Automaticity, the spontaneous depolarization of pacemaker myocytes, occurs in SA and AV nodes as well as in Purkinje fibers. In SA and AV nodal cells, the rate of firing depends on several different inward and outward currents; the combination of currents renders these cells relatively insensitive to depression by antiarrhythmic drugs [1,4,5]. In Purkinje fibers, however, automaticity occurs as an escape phenomenon that arises in the presence of AV block. Escape beats probably result from the action of a single inward sodium channel (the “pacemaker current”) and are suppressed by therapeutic concentrations of most class I antiarrhythmic agents. Therefore, Purkinje fiber automaticity is more susceptible to depression by antiarrhythmic agents than is sinus node automaticity. Nonetheless, clinical suppression of the sinus node leading to asystole, particularly in the presence of the high vagal tone commonly seen in the early phases of acute myocardial infarction, is an uncommon but well-recognized complication of therapy with antiarrhythmic agents such as lidocaine.
Reentrant circuit arrhythmias depend on conduction rates around the circuit and the refractory periods of pathway components. If the conduction time falls below the refractory period of part of the circuit, the “excitable gap” disappears, the advancing wavefront meets only refractory tissue, and the arrhythmia terminates. An ideal antiarrhythmic agent would, therefore, accelerate conduction and prolong refractoriness within the substrate for reentry. Many antiarrhythmic agents prolong refractory periods in myocardium, but none accelerates conduction in therapeutic use. Almost invariably, conduction tends to slow. This combination of decreasing conduction and refractory period prolongation can be either proarrhythmic or antiarrhythmic [5]. However, clinicians cannot predict which outcome is likely for a given drug in a given patient. Some antiarrhythmic agents (in particular, class IB drugs and amiodarone [3]) show selectivity for depressing conduction in ischemic or otherwise abnormal myocardium by binding preferentially to the inactivated state of the sodium channel. A complete conduction block through an ischemic segment of a reentrant circuit may be the mechanism of arrhythmia termination; this could occur without slowing conduction in healthy myocardium. Other drugs tend to show less selectivity and depress conduction in normal myocardium at therapeutic
concentrations, probably explaining the greater propensity of class IC drugs to be proarrhythmic both in therapeutic use and in overdose [3].
concentrations, probably explaining the greater propensity of class IC drugs to be proarrhythmic both in therapeutic use and in overdose [3].
Although most antiarrhythmic agents prolong refractoriness, lidocaine, mexiletine, and tocainide tend to shorten it, particularly in low concentrations; this may explain some cases of drug-associated arrhythmogenesis in patients with reentrant tachycardias. Lengthening of refractoriness should be proarrhythmic, but if conduction is slowed simultaneously, the net effect on the reentrant circuit determines the outcome.
Antiarrhythmic drugs suppress most forms of automaticity known to cause tachyarrhythmias. The major exception to this rule is the form of triggered automaticity due to early after-depolarizations (EADs). EADs can be defined as a marked slowing of repolarization, visible on the action-potential recording and due to reduction of the normal repolarizing outward potassium current. If voltage conditions are appropriate, prolonged depolarization may trigger a series of automatic action potentials. The upstrokes of these action potentials are due to inward current flow through the normal calcium channels that had been inactivated, had recovered from inactivation, and had found the membrane potential still within their activation range. The channels then reactivate and produce a secondary upstroke. Increased intracellular calcium concentrations activate calcium-sensitive potassium channels and accelerate repolarization. This process can occur as a single event or as an oscillatory series of action potentials, depending on the prevailing conditions of voltage and calcium levels [6,7,8].
The induction of EADs may be the basis of arrhythmias, including torsade de pointes associated with long QT syndromes [5,6,9]. According to this theory, the slowing of repolarization leads directly to the QT wave prolongation, often with associated prominent, bizarre TU waves. Any triggered activity, should it occur, results in ventricular tachyarrhythmias.
The class IA antiarrhythmic agents quinidine, disopyramide, and procainamide are all capable of producing EADs and torsade de pointes [6]. This is also true of the class III drugs, such as amiodarone, sotalol, ibutilide, and dofetilide. The class IB agents, lidocaine, mexiletine, and tocainide, do not produce EADs and do not cause torsade de pointes. Class IC compounds infrequently cause significant slowing of repolarization and have not been shown to cause torsade de pointes. Of the class IV agents, only mibefradil has an effect on repolarization, which usually manifests as TU-wave changes. Experimental models suggest that this effect is not proarrhythmic; however, there has been a report of torsade due to QT prolongation from therapeutic dosing of mibefradil [10,11].
Clinical Presentation
Toxicity common to therapeutic doses and overdoses of antiarrhythmic agents include depression of automaticity and cardiac conduction, which may be caused by a combination of direct electrophysiologic and secondary metabolic effects. Symptoms following acute overdose usually begin within 4 hours and can occur at any time during chronic therapy. Drug absorption may continue for many hours following the ingestion of large doses, sustained-release preparations, or agents with anticholinergic effects, resulting in delayed or progressive toxicity. Respiratory depression and hypotension produce acidosis and myocardial ischemia that further aggravate depressed conduction. Cardiac manifestations include QRS prolongation, QTc prolongation, sinus node dysfunction, bradycardia, AV block, ventricular arrhythmias, and poor ventricular function. These derangements can culminate in intractable arrhythmias, cardiogenic shock, or death. Manifestations of acute toxicity may also include dizziness, visual disturbances, psychosis, anticholinergic symptoms, hypoglycemia, hyperglycemia, and hypokalemia. Seizures may result from class I (particularly IB) toxicity. Procainamide and quinidine can cause hypotension if infused too rapidly.
Warning signs that indicate an increased risk of torsade include a QTc interval greater than 560 milliseconds, previous history of torsade, bradycardia, increased frequency and complexity of ventricular premature beats, or a ventricular premature beat falling on the T wave [12].
The electrocardiogram (ECG) may provide a clue to the agent or class involved in cases where the drug ingested is not known. Class IB drugs usually have no effect on the QT interval; whereas class IA, IC, and III agents prolong it. With class IA agents, QT prolongation is due to slowing of both depolarization and repolarization. Hence, both the QRS duration and JT interval are increased. In contrast, QT prolongation primarily results from slowed depolarization with class IC agents, resulting in an increased QRS (but not JT) duration and from prolonged repolarization with class III agents, resulting in an increased JT (but not QRS) interval.
The differential diagnosis of bradyarrhythmias includes beta-blocker, calcium channel blocker, cholinergic agent (carbamate and organophosphate insecticides), clonidine, cyclic antidepressant, and digitalis poisoning. Other agents that cause QRS and QT interval prolongation include antihistamines, antipsychotic agents, cyclic antidepressants, magnesium, and potassium. Ventricular tachyarrhythmias may occur in poisoning with sympathomimetics (see Chapter 144). Hypoglycemia, hypoxia, and metabolic disturbances should be considered in the differential diagnosis of patients with neurologic symptoms.
Diagnostic Evaluation
Physical examination should focus on vital signs and respiratory, cardiovascular, and central nervous system (CNS) function. Frequent vital signs and continuous cardiac monitoring should be performed. Essential tests include an ECG and serum electrolytes, blood urea nitrogen, creatinine, and magnesium measurement; liver function tests and serum drug levels, if available, may also be helpful. A chest radiograph should be obtained as clinically indicated. Patients with hypotension and hypoxemia should have arterial blood gas and serum lactate measurements.
Management
The general features of antiarrhythmic drug overdose and their management are discussed below (Table 120.3). Care of the antiarrhythmic poisoned patient centers on general supportive and critical care principles. Unique aspects pertinent to individual drugs are discussed in later sections. All patients suspected of ingesting an overdose of antiarrhythmic agents should receive oral activated charcoal. Patients with complications of therapeutic dosing may also benefit from oral activated charcoal to reduce absorption of a recently administered drug dose. The greatest amount of absorption to charcoal will occur if given within 1 to 2 hours of ingestions. CNS and respiratory depression commonly require airway support by endotracheal intubation. Seizures are managed by benzodiazepine therapy. Phenytoin should never be used to treat seizures secondary to drug toxicity because of the risk of increased mortality.
Initial therapy for hypotension involves administration of intravenous fluids. Because poisoned patients are infrequently hypovolemic, fluid administration should be monitored closely. In general, if a response in blood pressure is not seen with 2 L of intravenous fluids, pressors such as norepinephrine should be administered. Early consideration should be given to
circulatory assist devices for patients with cardiogenic shock. Intra-aortic balloon pump counterpulsation has been used successfully to treat patients with severe quinidine or disopyramide toxicity [13,14], and partial cardiac bypass has been used to maintain circulation during massive lidocaine or flecainide toxicity [15,16,17].
circulatory assist devices for patients with cardiogenic shock. Intra-aortic balloon pump counterpulsation has been used successfully to treat patients with severe quinidine or disopyramide toxicity [13,14], and partial cardiac bypass has been used to maintain circulation during massive lidocaine or flecainide toxicity [15,16,17].
Table 120.3 Management of Life-Threatening Antiarrhythmic Drug Overdose | |
---|---|
|
Decreased ventricular conduction, as measured by QRS prolongation in quinidine, procainamide, flecainide, and encainide toxicity, are often treated with sodium bicarbonate infusion [18,19,20,21,22]. In animals, hypertonic sodium bicarbonate reverses ventricular arrhythmias caused by flecainide toxicity [23] and reverses hypotension due to tricyclic antidepressants with class IA antiarrhythmic effects [20]. Hypertonic sodium bicarbonate should be considered for the treatment of QRS widening greater than 100 milliseconds or ventricular tachyarrhythmias in the setting of class IA or IC drug toxicity. Common practice is to administer intravenous boluses of sodium bicarbonate (50 mEq of 1 mEq per mL solution) as needed to increase and maintain blood pH between 7.45 and 7.55. As an alternative, a continuous infusion of 1,000 mL of 5% dextrose in water containing 2 to 3 amps of sodium bicarbonate and potassium chloride is an option. Bicarbonate should be administered for 12 to 24 hours and then gradually withdrawn while watching for QRS lengthening to recur. At present, there is no evidence that prophylactic alkalinization before QRS widening changes outcome. In the most severely poisoned patients, however, alkalinization may be ineffective, especially if there is persistent metabolic acidosis. In a series of patients with class I antiarrhythmic drug overdose requiring cardiopulmonary resuscitation, only 2 of 29 survived despite the use of hypertonic sodium bicarbonate [24].
Sodium bicarbonate appears to act by increasing the extracellular sodium concentration and reducing the drug-induced sodium channel blockade [25]. Hypertonic sodium chloride has proven effective in animals and, anecdotally, in humans, but sodium bicarbonate is generally preferable because increasing pH is equally or more important in some models [20,25,26,27] (see Chapter 123 for more detail).
The treatment of recalcitrant ventricular tachycardia typically consists of repeated cardioversions, cardiopulmonary resuscitation, vasopressor support, and mechanical ventilation. Treatment with other class IA and IC antiarrhythmic drugs is contraindicated, given the potential for further arrhythmia aggravation [28]. Lidocaine may be considered because it does not depress conduction, but it is often ineffective. Suppression of ventricular tachycardia and hemodynamic improvement has been anecdotally described with sodium bicarbonate [21,22]. Overdrive pacing may also be effective.
The treatment of torsade de pointes should include 1 to 2 g of a 25% solution of intravenous magnesium sulfate. Direct-current cardioversion is often effective in terminating torsade de pointes, but it frequently recurs. Increasing the ventricular rate to greater than 90 to 110 beats per minute by an infusion of isoproterenol or ventricular pacing may also be effective [29,30]. In one study, infusion of potassium chloride at 0.5 mEq per kg for 60 to 90 minutes normalized excessive quinidine-induced QT prolongation, but simply correcting hypokalemia did not suppress torsade de pointes [31]. Lidocaine is inconsistently effective [32,33]. Treatment with class IA or III antiarrhythmic drugs is contraindicated because further prolongation of repolarization and the QT interval may exacerbate torsade de pointes. Magnesium therapy should also be considered in patients at increased risk for this arrhythmia (see earlier); it has been found to prevent occurrence of torsade in a dog model (dose of 30 to 60 mg per kg) [34].
Although most antiarrhythmic drugs are weak bases, urine acidification is contraindicated because systemic acidosis may aggravate cardiotoxicity [20]; treatment with hypertonic alkaline solution to reduce cardiotoxicity is likely to be of greater benefit. Hemodialysis is of limited benefit for antiarrhythmic toxicity because drug clearance is limited by protein binding and high lipid solubility [26,35,36]. Hemoperfusion using charcoal resin is more effective in removing drugs with high protein binding and high lipid solubility; however, this modality is rarely available. Hemoperfusion is of greatest value for disopyramide [37] or N-acetylprocainamide (NAPA) toxicity [38].
Individual Agents
Class IA Agents
Quinidine
Quinidine is administered orally as sulfate or gluconate. The usual dose of immediate-release quinidine sulfate is 200 to 400 mg, four times per day, with gluconate doses being approximately 30% higher. Bioavailability is approximately 70% for both forms; peak plasma levels are reached earlier for the sulfate (60 to 90 minutes) than for the gluconate. Quinidine is metabolized by CYP3A4 to 3-OH quinidine and
quinidine-N-oxide; these metabolites have less electrophysiologic activity than quinidine [39,40]. Details of pharmacokinetics are listed in Table 120.4.
quinidine-N-oxide; these metabolites have less electrophysiologic activity than quinidine [39,40]. Details of pharmacokinetics are listed in Table 120.4.
Table 120.4 Dose and Pharmacokinetics of Class I and II Antiarrhythmic Agents | ||||||||||||||||||||||||||||||||||||||||||||||||||||||||||||||||||||||||||||||||||||||||||||||||||||||||||||||||||||||||||||||||||||||||||||||||||||||||||||||||||
---|---|---|---|---|---|---|---|---|---|---|---|---|---|---|---|---|---|---|---|---|---|---|---|---|---|---|---|---|---|---|---|---|---|---|---|---|---|---|---|---|---|---|---|---|---|---|---|---|---|---|---|---|---|---|---|---|---|---|---|---|---|---|---|---|---|---|---|---|---|---|---|---|---|---|---|---|---|---|---|---|---|---|---|---|---|---|---|---|---|---|---|---|---|---|---|---|---|---|---|---|---|---|---|---|---|---|---|---|---|---|---|---|---|---|---|---|---|---|---|---|---|---|---|---|---|---|---|---|---|---|---|---|---|---|---|---|---|---|---|---|---|---|---|---|---|---|---|---|---|---|---|---|---|---|---|---|---|---|---|---|---|---|
|
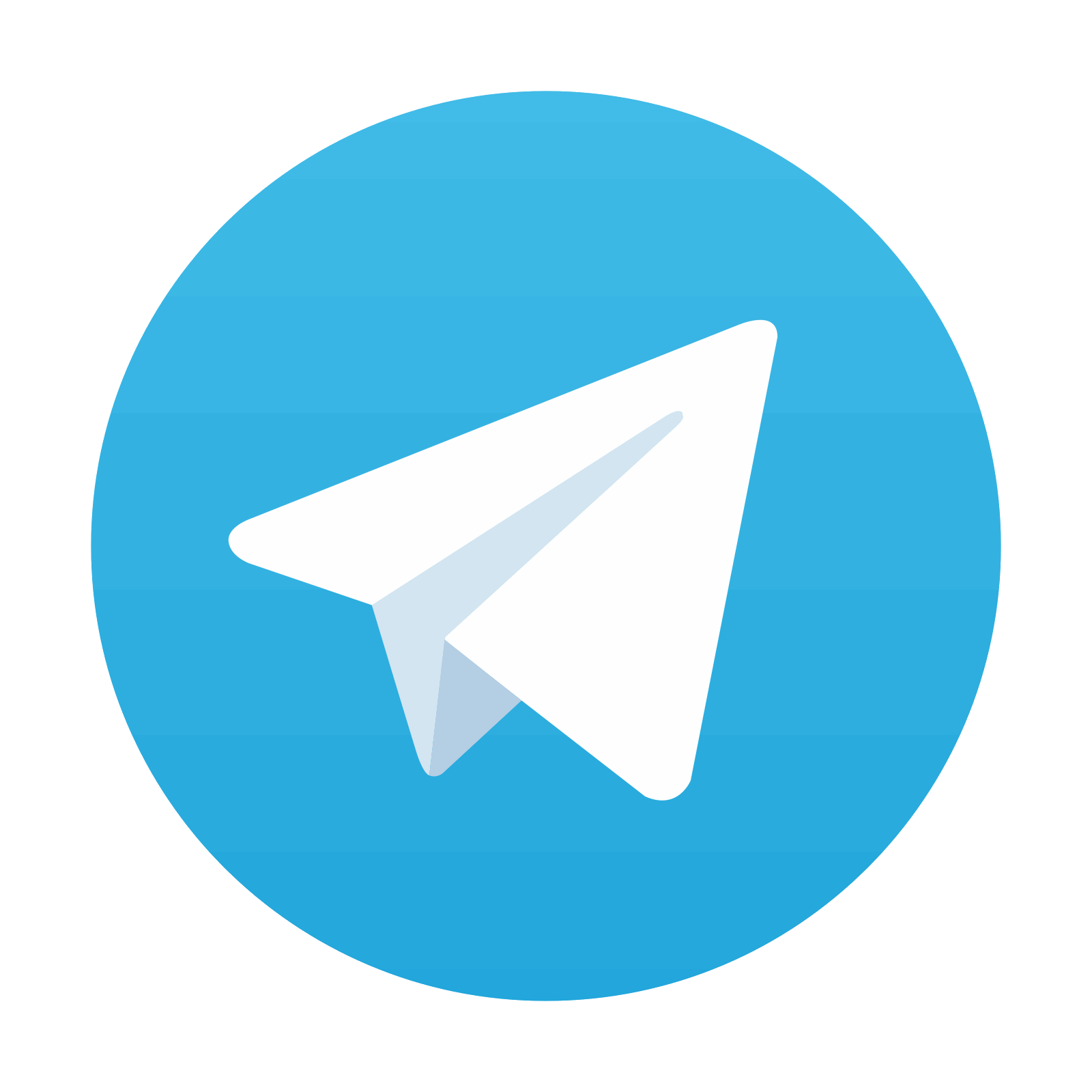
Stay updated, free articles. Join our Telegram channel

Full access? Get Clinical Tree
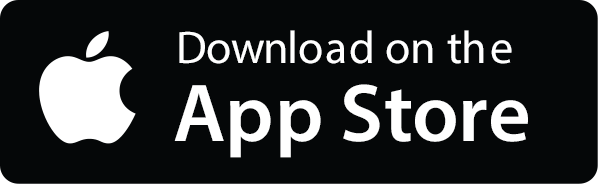
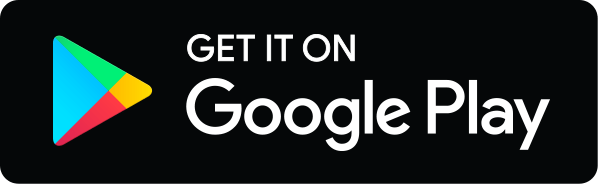
