Data adapted from Bullock RM, et al. and Guidelines, 3rd ed.
PaCO2, arterial carbon dioxide tension; SaO2, arterial oxygen saturation; PaO2, arterial oxygen tension; GCS, Glasgow Coma Scale; CT, computed tomography; CPP, cerebral perfusion pressure; ARDS, adult respiratory distress syndrome; TBI, traumatic brain injury.
Evidence-based guidelines for prehospital management of TBI and for pediatric brain injury have also been published, and in March 2006, surgical management guidelines were published. Compared with the evidence informing Guidelines for the Management of Severe Traumatic Brain Injury, however, there are no controlled clinical trials in the literature to support different forms of surgical management or surgical versus conservative therapy. As with the other guidelines in severe TBI, the authors of the surgical management guidelines stated in 2006 that “this is a document in evolution,” and revisions will be made as new knowledge is gained.
II. CLASSIFICATION OF HEAD INJURY INTO PRIMARY INJURY AND SECONDARY INJURY IS USEFUL WHEN CONSIDERING THERAPEUTIC STRATEGIES
A. Primary injury is the damage produced by the direct mechanical impact and the acceleration–deceleration stress onto the skull and the brain tissue, which results in skull fractures (cranial vault, skull base) and intracranial lesions. The intracranial lesions are further classified into two types: diffuse injury and focal injury.
1. Diffuse brain injury
a. Brain concussion: loss of consciousness lasting < 6 hours
b. Diffuse axonal injury: traumatic coma lasting > 6 hours
2. Focal brain injury
a. Brain contusion: located either below or opposite the region of impact
b. Epidural hematoma (EDH): often caused by skull fracture and laceration of the middle meningeal artery
c. Subdural hematoma (SDH): usually caused by the tearing of the bridging veins between the cerebral cortex and the draining sinuses. Acute SDH is often associated with high mortality.
d. Intracerebral hematoma is usually located in the frontal and the temporal lobes and visualized as a hyperdense mass on a computed tomographic (CT) scan. Brain tissue destroyed by the primary impact will not be saved. Therefore, functional outcome is improved by prompt surgical intervention and medical therapy.
3. Indications for surgery. Presently, no definitive therapeutic measure exists to treat diffuse axonal injury. Most open and depressed skull fractures and compound skull fractures with dural laceration require early surgical repair. Uncomplicated basal fractures usually do not require operation. The presence of compression of the basal cisterns from a brain contusion necessitates operative intervention because of the risk of herniation (usually of the temporal lobe). Intracranial hematoma is the most common sequela of head trauma requiring surgical treatment. An EDH > 30 cm3 should be surgically evacuated regardless of the GCS score. Patients who have an acute EDH, a GCS score < 9, and anisocoria (inequality of pupillary diameter) should undergo urgent surgical evacuation. An acute SDH > 10 mm thick or a midline shift > 5 mm on CT scan requires evacuation. If the GCS score is < 9 in patients who have an SDH < 10 mm thick, intracranial pressure (ICP) monitoring must be instituted.
B. Secondary injuries develop within minutes, hours, or days of the initial injury and cause further damage to nervous tissue. The common denominators of secondary injury are cerebral hypoxia and ischemia. Secondary injuries are caused by the following disorders:
1. Respiratory dysfunction: hypoxemia, hypercapnia
2. Cardiovascular instability: hypotension, low cardiac output (CO)
3. Elevation of ICP
4. Metabolic derangements
III. PATHOPHYSIOLOGY OF HEAD TRAUMA
A. Systemic effects
1. Cardiovascular responses commonly observed in the early stage after TBI include hypertension, tachycardia, and increased CO. Patients who have severe TBI and those suffering from multiple systemic injuries with substantial blood loss may, however, develop hypotension and a decrease in CO. Systemic hypotension (systolic blood pressure [SBP] < 90 mm Hg) at the time of hospital admission is associated with significantly increased morbidity and mortality.
2. Respiratory responses to head trauma include apnea and abnormal respiratory patterns. Respiratory insufficiency and spontaneous hyperventilation often occur. Patients may also suffer from central neurogenic pulmonary edema and aspiration of blood, teeth, and vomitus.
3. Temperature regulation may be disturbed, and hyperthermia, if it occurs, can provoke further brain damage.
B. Changes in cerebral circulation and metabolism
1. In focal brain injury, cerebral blood flow (CBF) and cerebral metabolic rate for oxygen consumption (CMRO2) decrease in the core area of injury and in the penumbra, the area of hypoperfused tissue that surrounds the damaged tissue. When ICP increases, the cerebral perfusion pressure (CPP), the difference between the mean arterial pressure (MAP) and the ICP, decreases and diffuse and more marked hypoperfusion and hypo-metabolism ensue.
2. In diffuse brain injury, hyperemia may occur. In most cases, however, CBF decreases within a few hours of head trauma. The combination of hypotension and impaired autoregulation exacerbates cerebral ischemia. The chemical-metabolic regulation of CBF may also be impaired.
C. Acute brain swelling and cerebral edema
1. Acute brain swelling is provoked by a decrease in vasomotor tone and a marked increase in the volume of the cerebral vascular bed. In this situation, increases in BP can easily lead to further brain swelling and an increase in ICP.
2. Cerebral edema after head trauma is often a mixture of vasogenic and cytotoxic types caused by blood–brain barrier (BBB) disruption and ischemia, respectively.
Both acute brain swelling and edema ensue concurrently after TBI. When these pathologic conditions occur in association with an intracranial hematoma, the resultant intracranial hypertension causes a further reduction in CBF and exacerbation of cerebral ischemia. Eventually, intracranial hypertension, if untreated, leads to herniation of the brain stem through the foramen magnum.
D. Excitotoxicity. Head trauma causes excessive release of glutamate from neurons and glia, increasing the concentration of glutamate in the cerebrospinal fluid (CSF). The biochemical changes associated with the excessive release of glutamate and the activation of glutamate receptors are closely related to an increase in intracellular calcium, which triggers a number of events that lead to cellular damage. These include the activation of phospholipase, protein kinase, proteases, nitric oxide synthase (NOS), and other enzymes. Activation of these enzymes also leads to lipid peroxidation, proteolysis, free-radical formation, deoxyribonucleic acid (DNA) damage, and, finally, neuronal death (Fig. 7.1).
FIGURE 7.1 Excitotoxicity in head injury. AMPA, alpha-amino-3-hydroxy-5-methyl-4-isoxazole propionate; NMDA, N-methyl-D-aspartate; [Ca]i, intracellular calcium; DNA, deoxyribonucleic acid.
E. Inflammatory cytokines and mediators. The cytokines are major mediators in the initiation of inflammatory and metabolic responses to injury. Cytokines increase in response to cerebral ischemia. Interleukin-6 (IL-6) and tumor necrosis factor alpha are known to be released after TBI. Patients who have GCS scores < 8 show a higher and more sustained elevation of IL-6. The cytokines released after TBI stimulate the production of free radicals and arachidonic acids and upregulate the activity of the adhesion molecules, which produce disturbances in the microcirculation. All of these changes contribute to secondary brain injury.
IV. EMERGENCY MANAGEMENT
A. Initial assessment of the patient’s condition
1. Neurologic assessment. There is usually little time initially for thorough evaluation of the patient’s neurologic condition. However, a quick neurologic assessment can be performed while achieving adequate ventilation (oxygenation and carbon dioxide [CO2] elimination) and hemodynamic stability.
a. GCS (Table 7.2) is a simple and universally accepted method for assessing consciousness and neurologic status in patients after TBI. The GCS has low interobserver variability and is a good predictor of outcome. Even patients who have good GCS scores need to be closely monitored. Patients may deteriorate rapidly, especially those who have medial temporal lesions as even slight expansion in this region may result in herniation. CT scans are obtained in patients who have GCS score < 15 or if loss of consciousness has occurred.
TABLE 7.2 Glasgow Coma Scale
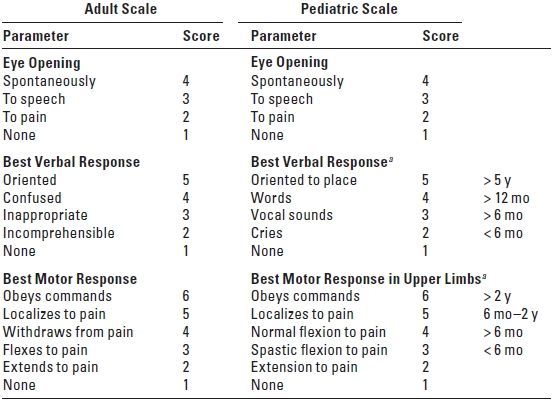
aScore highest appropriate for age.
1. GCS score < 8 characterizes severe head injury.
2. GCS score of 9 to 12 represents moderate injury.
3. GCS score of 13 to 15 represents mild injury.
b. Pupillary responses (size, light reflex, accommodation) and symmetry of motor function in the extremities should be quickly assessed.
2. Assessment of injuries to other organs. Trauma patients often suffer from injuries to multiple organ systems, and therefore require early diagnosis of intrathoracic and intraperitoneal (intrapelvic) injuries. If bleeding is suspected, the thorax or the abdomen should be explored without delay. Treatment of hemorrhagic shock takes precedence over neurosurgical procedures. The patient’s hemoglobin must be measured immediately and blood and fresh-frozen plasma ordered for infusion.
B. Establishment of airway and ventilation
1. Tracheal intubation. The first step in emergency therapy is to secure an open airway and ensure adequate ventilation. Because all trauma patients are considered to have a “full stomach” and approximately 10% have an associated cervical spine injury as well, cricoid pressure and manual inline stabilization of the cervical spine are used during laryngoscopy and intubation.
If facial fractures and soft tissue edema prevent direct visualization of the larynx, either fiberoptic intubation or intubation with an illuminated stylet may be attempted. In the presence of either severe facial injuries or laryngeal trauma, a cricothyrotomy may be required. Nasal intubation is avoided in the presence of a suspected basilar skull fracture, severe facial fractures, and a bleeding diathesis. Nasal intubation also adds the risk of introducing contaminated material into the brain when there is a basilar skull fracture. Hemorrhage of the tympanic cavity, otorrhea, petechiae on the mastoid process (Battle’s sign), and petechiae around the eyes (panda sign) are all indications of a basilar skull fracture.
2. Drugs to facilitate laryngoscopy and intubation (see Section V)
3. Mechanical ventilation. As soon as the trachea has been intubated, a nondepolarizing muscle relaxant is administered and mechanical ventilation to an arterial carbon dioxide tension (PaCO2) of approximately 35 mm Hg is instituted. Aggressive hyperventilation to a PaCO2 < 30 mm Hg is avoided unless transtentorial herniation is suspected. Hypoxemia, if present, is corrected immediately. Hyperoxia may be recommended. If massive aspiration is suspected, bronchial suctioning using a fiberscope is advisable before transferring the patient to either the neuroradiology suite or the OR.
C. Cardiovascular stabilization. Systemic hypotension is one of the major contributors to poor outcome after head trauma. When necessary, fluid resuscitation is initiated immediately and inotropic and vasopressor drugs are administered as required to stabilize the BP.
1. Fluid resuscitation. Hypovolemia is often masked by a relatively stable BP secondary to either sympathetic hyperactivity or the reflex response to increased ICP. Therefore, fluid resuscitation should be guided not only by BP but also by urinary output and central venous pressure (CVP). Total osmolality is the most important factor in determining brain edema formation. Colloid oncotic pressure has a very minor effect on pressure gradients for diffusion of water across the BBB. Care should be taken during fluid resuscitation as these patients are at risk for pulmonary edema, which may worsen hypoxia.
a. Crystalloid and colloid solutions. Isotonic and hypertonic crystalloid solutions and colloid solutions may be given to maintain adequate intravascular volume. Normovolemia is the intended fluid management goal. Any negative fluid balance from the use of diuretics may have a deleterious effect on a brain that has impaired autoregulation.
1. Lactated Ringer’s solution is slightly hypotonic (273 mOsm/L) relative to plasma, which precludes the use of a substantial amount. If administered, serum osmolality should be measured periodically. When large-volume resuscitation with crystalloid is required, an isotonic crystalloid, such as normal saline (308 mOsm/L), is preferable.
2. Hypertonic saline (HS) (3%, 7.5%) can be beneficial in small amounts after TBI. HS was shown to reduce brain water content in non-traumatic brain tissue in an animal model. A rebound phenomenon, as occurs with mannitol, is not seen with HS given to patients after TBI. Pre-existing hyponatremia should be excluded as there is a risk of central pontine myelinolysis after rapid correction with HS. Infusion of HS should be carefully monitored in patients who have cardiac problems as it may aggravate pulmonary edema. HS may be more effective in patients who have refractory intracranial hypertension. Research is still needed to determine the optimal administration and concentration of HS.
3. Hydroxyethyl starch (HES) and albumin can be administered to maintain intravascular volume for longer periods. No more than 1.5 L of HES should be administered in conjunction with careful monitoring of coagulation parameters. The incidence of coagulopathy in patients after TBI is approximately 20%, and HES in large amounts is known to interfere with clotting. The reported incidence of coagulopathy is less with pentastarch. Dextrans are usually avoided owing to their effect on platelet function. Hypertonic saline/dextran combinations have been shown to reduce mortality and decrease the incidence of adult respiratory distress syndrome (ARDS) in the trauma patient.
b. Blood and blood products. Patients who have a low hematocrit may require a transfusion to optimize oxygen delivery; the hematocrit ideally is maintained at > 30%. Children require special attention because they can easily become hypovolemic by losing large but unsuspected volumes of blood into an intracranial or a subgaleal hematoma or through a scalp laceration, even without blood loss from other organs. Release of brain tissue thromboplastin may trigger disseminated intravascular coagulation (DIC) in which case fresh-frozen plasma, platelets, and cryoprecipitate may be required.
c. Adverse effect of glucose-containing solutions. Glucose-containing solutions are avoided because hyperglycemia is associated with worsening of neurologic outcome. Glucose should be used only to treat hypoglycemia. A plasma level of 80 to 150 mg/dL is desirable; values > 200 mg/dL should be avoided and treated with insulin. When insulin protocols are used, care is also taken to avoid hypoglycemia as it may have an even more harmful effect on outcome.
2. Inotropes and vasopressors.
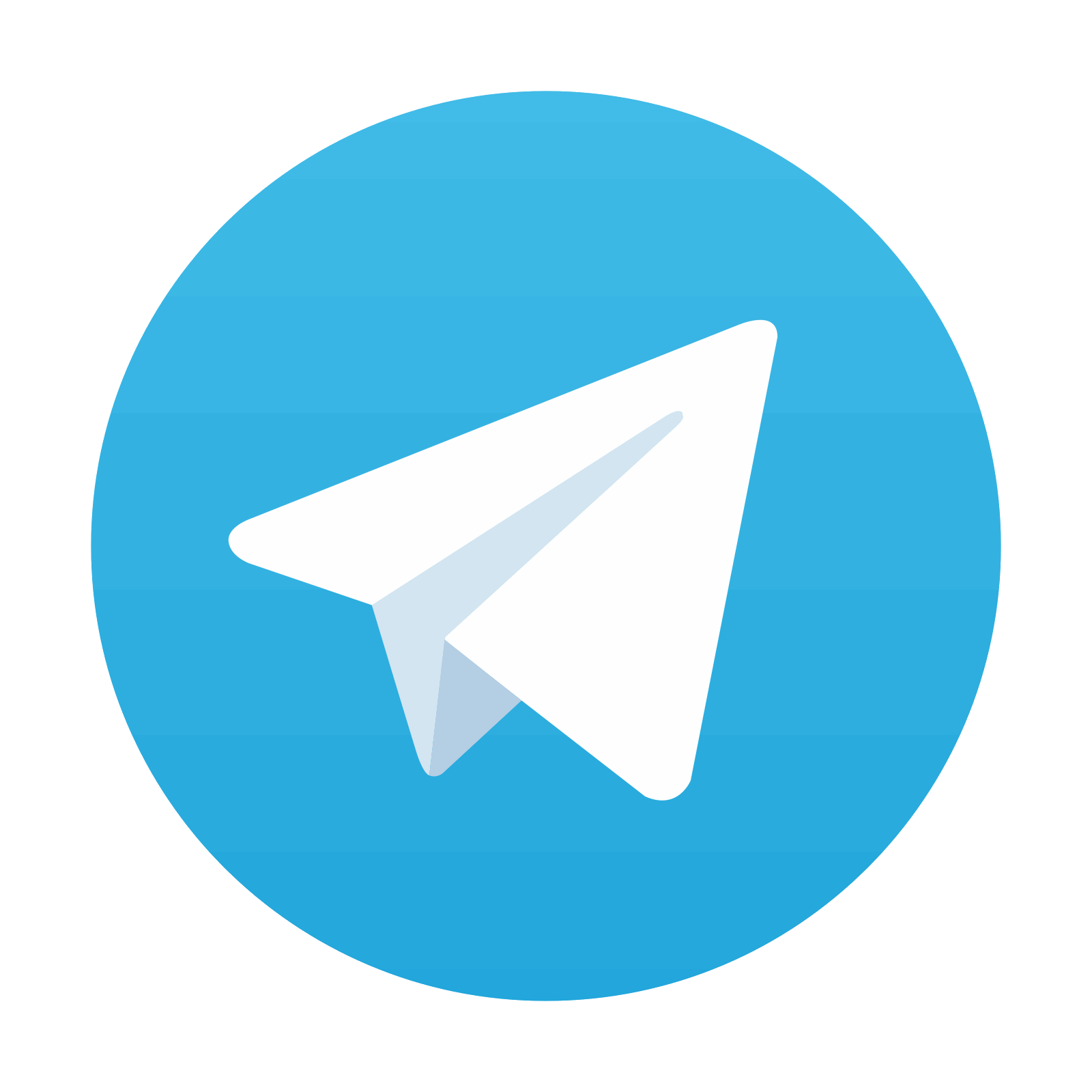
Stay updated, free articles. Join our Telegram channel

Full access? Get Clinical Tree
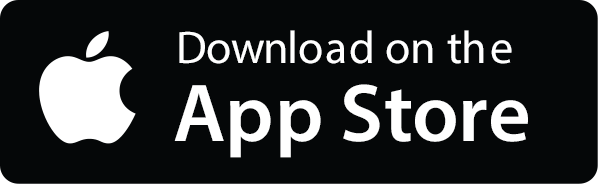
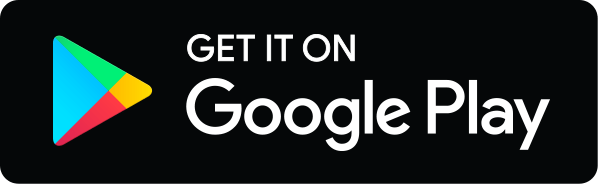