Technical advances in neuroimaging are occurring rapidly. Combination modalities such as single photon emission computed tomography/computed tomography (SPECT/CT) are being used with increasing frequency.
Anesthesia care must often be provided far from the operating room (OR) and under challenging circumstances.
Excellent communication between the radiology and anesthesia teams is paramount.
Anesthesiologists must be aware of potential contrast reactions and appropriate therapy.
The introduction of the first CT scanners in 1975 revolutionized the imaging of neurosurgical lesions. Since that time, the diagnostic armamentarium of the neuroradiologist in terms of neuroimaging has expanded progressively. Diagnostic procedures usually involve limited pain so that the neuroradiologist or a sedation team may administer sedation. The anesthesiologist is involved, however, in cases for which either difficulty with sedation or the need for general anesthesia is anticipated for a myriad of reasons. These include extremes of age, claustrophobia, serious neurologic or systemic illness, and the inability of patients to remain motionless to be able to achieve high-quality images.
Many radiology departments have recently adopted the guidelines for the sedation of children formulated by the American Academy of Pediatrics Workgroup on Sedation. These guidelines address patient selection, dietary precautions, equipment, monitoring, and discharge criteria. Furthermore, strict selection criteria minimize sedation-related side effects. The screening of patients before the procedure often reveals those who have either anticipated airway difficulties or serious medical conditions for whom the presence of an anesthesiologist becomes advisable. In one study, 13.1% of patients were inadequately sedated for their procedure, resulting in failure of 3.7% of procedures. Older children and those undergoing MRI and CT scans experienced higher failure rates. In addition, Malviya found that 5.5% of children sedated by non-anesthesiologists experienced an untoward respiratory event. These events occurred more commonly in American Society of Anesthesiologists (ASA) status III and IV patients.
I. GENERAL CONSIDERATIONS
Once involved in the neuroradiologic procedure, the anesthesiologist faces the usual problems of providing anesthesia in an environment foreign to and far from the OR. Non-ferromagnetic equipment and monitoring modalities are required in the MRI suite, and most neuroradiographic procedures require that the anesthesiologist be positioned at a distance from the patient. Additionally, the issue of contrast media with their attendant osmolar effects and potential for toxic and allergic reactions must be considered. Finally, the design of modern medical facilities often requires that patients be either recovered by qualified personnel in an area adjacent to the radiology suite or transported over relatively large distances through the medical center to the regular PACU.
A. Evaluation. A thorough preoperative evaluation should be performed for each patient scheduled for a neurodiagnostic procedure. In addition to elucidation of the patient’s medical and surgical history, allergies, medications, and experience with previous sedatives and anesthetics, particular attention must be directed to the neurologic examination. Patients are carefully evaluated for signs of increased intracranial pressure (ICP), preexisting neurologic deficits, and, in the case of the emergency head-injured patient, concomitant (and sometimes undiagnosed) injury to the spine and other major organ systems.
B. Equipment available in the radiology suite should include compatible anesthesia delivery systems, adequate supplies of medical gases, suction apparatus, age-appropriate drugs and airway equipment, and monitors for oxygen saturation, end-tidal carbon dioxide (ETCO2), electrocardiogram (ECG), and blood pressure (BP) compatible with the imaging modality.
II. COMPUTED TOMOGRAPHY
A. Basic considerations. Ionizing radiation is delivered to the patient during CT scanning. Radiation detectors are permanently fixed all of the way around the CT gantry. For each image, an x-ray tube rotates in a circle within the detector ring emitting a beam of ionizing radiation that passes through the body. The multiple detectors record the quantity of the x-ray beam that is attenuated or absorbed as it passes through the patient’s tissues. This information from various angles is electronically integrated and the average attenuation value of each point in space is expressed in Hounsfield units.
Attenuation by a substance is directly related to its electronic density. Therefore, structures are hypodense (of lower attenuation), isodense (of similar attenuation), or hyperdense (of higher attenuation) relative to the brain parenchyma. The attenuation of vascular structures is increased by the use of iodinated contrast drugs. While CT axial images are displayed initially, high-resolution thin images can be transformed into coronal and sagittal sections as well as 3-dimensional representations through computer reformatting.
CT scanning is relatively insensitive for viewing structures within the posterior fossa because of image degradation by the artifact produced by the interface of bone and brain parenchyma. This modality remains the choice, however, for the detection of skull fractures and acute subarachnoid hemorrhage in the emergent setting. The helical or spiral acquisition CT scan is also popular because larger anatomic regions can be imaged quickly, which is particularly useful in the trauma situation. For example, visualization from the aortic arch to the circle of Willis can be accomplished in < 1 minute of scan time. With spiral acquisition scanning, unlike conventional CT scans, the patient is moved at a constant speed through the scanning field while the x-ray tube rotates continuously. CT angiography utilizes spiral acquisition scanning during the administration of iodinated contrast as an intravenous bolus. Complex intracranial aneurysms < 2 mm in diameter can be visualized with CT angiography.
B. Management
1. Sedation. With the current rapid CT scanners, very few adults or school-aged children require sedation for CT scanning. Occasionally, either intravenous midazolam in titrated doses of 0.5 mg or an infusion of propofol at a dose of 25 to 100 mcg/kg/minute may be used for conscious sedation in an anxious adult patient for the brief procedure.
2. General anesthesia. If an adult or older child manifests signs and symptoms of increased ICP or serious airway compromise, it is better to proceed with general anesthesia rather than attempt sedation. Several suggested techniques for the sedation of small children are listed in Table 18.1. In addition to concerns about equipment, monitoring, and airway management, it must be remembered that the temperature of children within the cold radiology suite environment must be carefully maintained.
TABLE 18.1 Sedation Techniques for Small Children
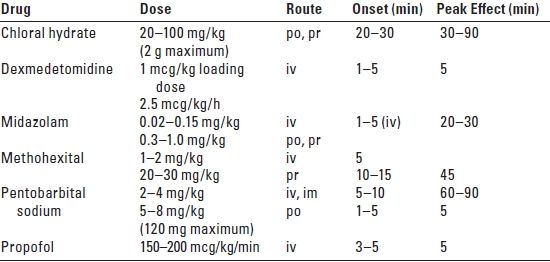
po, by mouth; pr, by rectum; iv, intravenous; im, intramuscular.
In the clinical situation where increased ICP is a critical factor, the intravenous induction of general anesthesia can be accomplished in children after the application of EMLA (lidocaine 2.5% and prilocaine 2.5%) cream to the dorsum of the patient’s hands and insertion of an intravenous catheter. The issue of undiagnosed muscular dystrophy mitigates against the use of succinylcholine in children. An induction utilizing rocuronium, 0.6 to 1 mg/kg, for relaxation is often indicated. Anesthesia in children is maintained with an intravenous infusion of propofol, 25 to 100 mcg/kg/ minute, or low concentrations of inhaled anesthetics.
In adults, an intravenous induction utilizing thiopental, 3 to 4 mg/kg, or propofol, 1 to 2 mg/kg, followed by succinylcholine, 1 mg/kg, with the addition of a small dose of narcotic such as fentanyl, 50 to 100 mcg, and lidocaine, 1 mg/kg, to deepen the anesthetic is appropriate for endotracheal intubation. Rapid awakening at the end of the procedure is desirable. An infusion of remifentanil, 0.1 to 0.2 mcg/kg/minute, is an alternative in adults and children.
III. MAGNETIC RESONANCE IMAGING
MRIs are acquired when the protons on water molecules within the patient’s body are excited through a combination of a strong magnetic field and intermittent radio frequency (RF) pulses. Additional magnetic fields are applied to create gradients and excite the protons to different orientations within the basic magnetic field. In the excited state, these protons gain energy and shift from a low energy state to a high-energy state. This process is termed resonance. When the RF pulse is turned off, the protons lose energy in two ways: T1, the longitudinal relaxation time, and T2, the transverse relaxation time. Fortunately, no ionizing radiation is employed with MRI, but a large, powerful, external magnetic field is necessary.
Standard MRI sequences include T1-weighted images, proton density, and T2-weighted images, as well as T1 images with the intravenous administration of gadolinium contrast material. Fluid attenuated inversion recovery (FLAIR) utilizes T2 weighting, but suppresses the cerebrospinal fluid (CSF) signal, increasing the visibility of lesions in the cortex, subarachnoid space, and perivertebral region. Gradient recalled echo
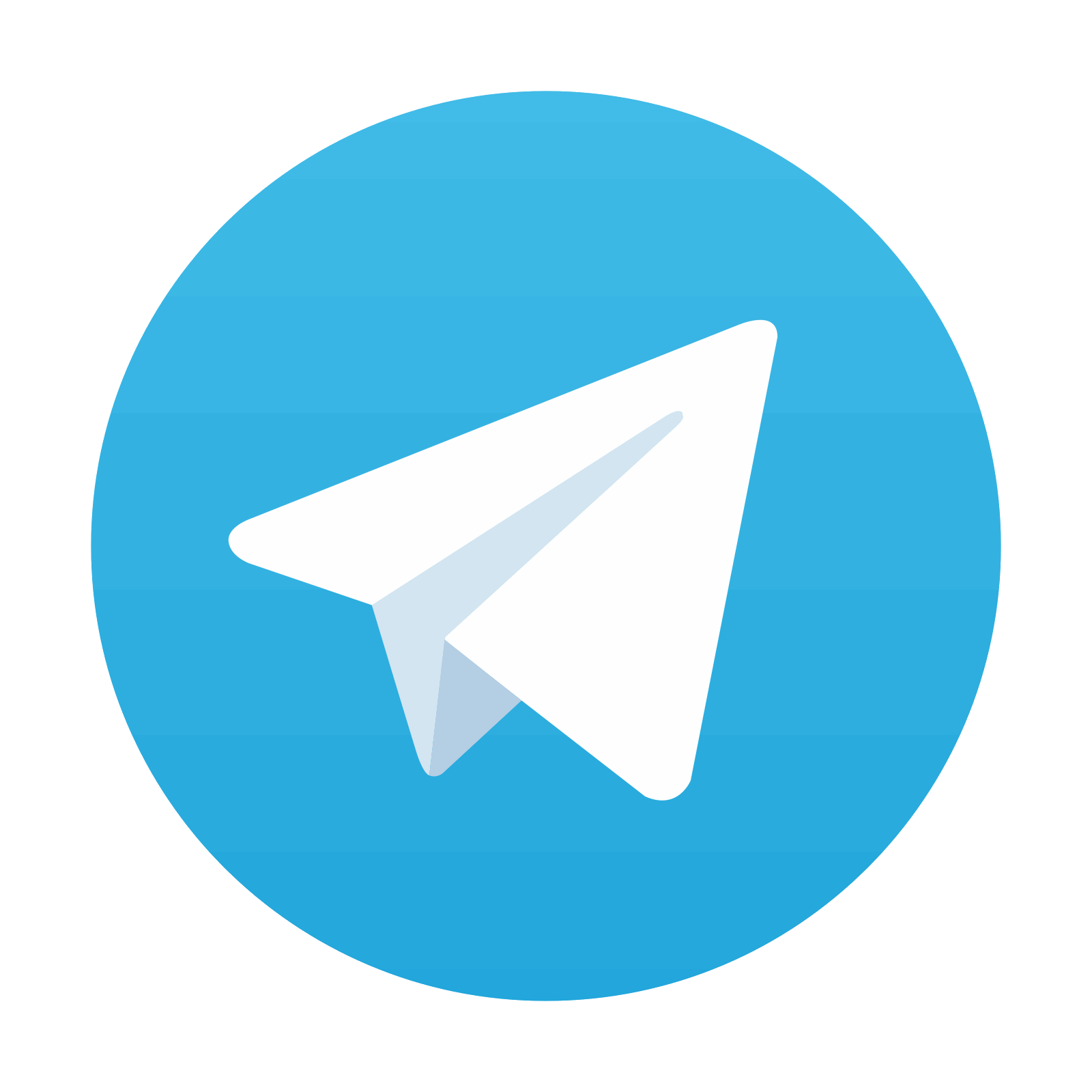
Stay updated, free articles. Join our Telegram channel

Full access? Get Clinical Tree
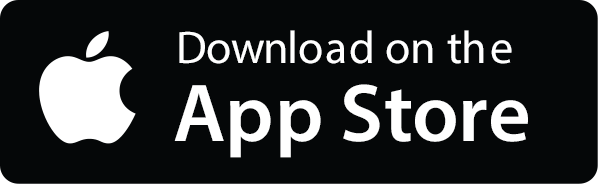
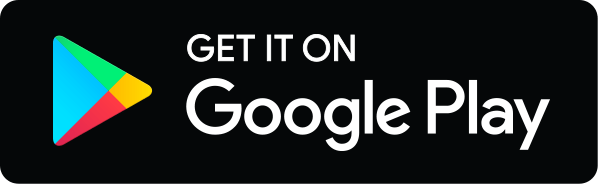