Although it is simplistic, the statement by the British neurosurgeon J. Gillingham that “in the early years anesthetists spent their time pushing the brain out of the skull while in recent times they have been sucking it back in” underscores the importance and contribution of neuroanesthesia to the improved results of the surgical treatment of cerebral aneurysms. Other advances are the improvements in microsurgical instrumentation such as the operating microscope, neuroradiology, neurophysiologic monitoring, and the development of specialized centers with surgeons and anesthesiologists dedicated to the treatment of patients with cerebral aneurysms. However, data published from the International Cooperative Study on the Timing of Aneurysm Surgery (Cooperative Study) in 1990 indicated that overall surgical mortality was high, at approximately 20%. , To a certain extent these results might have been biased because of the trend toward early operative intervention in high-risk patients who were previously considered unsuitable for surgery. Nonetheless, even patients admitted in good condition with a level of consciousness score equivalent to Hunt and Hess grades I and II (see later discussion) have a “good” recovery rate of only 58% and a mortality rate of 26%. Thus, much room exists for improvement in all aspects of cerebral aneurysm treatment. Efforts at improving outcome and mortality seem to be making some progress: in 2002 mortality rates were about 8%; however, the rates for those with a good outcome remained about the same at 64–75%. Considerable variations in mortality and morbidity exist among centers of the Cooperative Study, the reasons for which are not apparent. The leading causes of death and disability were, in descending order, vasospasm, the direct effects of the initial bleed (massive subarachnoid, subdural, or intracerebral hematoma, permanent ischemic effects of increased intracranial pressure [ICP]), rebleeding, and surgical complications. Successful anesthetic management of patients with cerebral aneurysms requires a thorough understanding of the natural history, pathophysiology, and surgical requirements of the procedures.
Endovascular treatment using thrombogenic coils is an alternative to surgical treatment. The results of the International Subarachnoid Trial (ISAT) showed that when an aneurysm is amenable to either endovascular coiling or surgical clipping, outcomes favor the coiling. , Longer term follow-up studies have questioned this result and have raised questions as to which modality is superior, , and there is interest in establishing in which cohort one treatment is favored over the other. Multiple factors must be evaluated, including aneurysm factors such as location and anatomy, and patient factors such as age, comorbidities, and patient wishes.
Preoperative considerations
The main steps in preoperative evaluation are as follows:
- 1.
Assessment of the patient’s neurologic condition and clinical grading of the subarachnoid hemorrhage (SAH)
- 2.
A review of the patient’s intracranial pathologic condition, including the performing of computed tomography (CT) and angiograms
- 3.
Monitoring of ICP and transcranial Doppler ultrasonography (TCD) if available
- 4.
Evaluation of other systemic functions, premorbid as well as current condition, with emphasis on systems affected by SAH
- 5.
Communication with the neurosurgeon regarding positioning, anticipated difficulty/technique to clip and special monitoring requirements
- 6.
Optimization of the patient’s condition by correcting any existing biochemical and physiologic disturbances.
The preoperative assessment allows appropriate planning of an anesthetic regimen with consideration of the pathophysiology of all organ systems as well as the surgical and monitoring requirements. This approach facilitates the goals of smooth anesthesia for an uncomplicated aneurysm and ensures a heightened level of preparedness for a complicated one.
The Central Nervous System
To allow better assessment of surgical risk and prognosis, Botterell and colleagues in 1956 first proposed the grading of subarachnoid hemorrhage, which was later modified by Hunt and Hess ( Table 13.1 ). In the 1980s a grading scale based on the Glasgow Coma Scale was introduced by the World Federation of Neurological Surgeons ( Table 13.2 ). In the World Federation classification, the most important correlate with outcome is the preoperative level of consciousness. These clinical grading schemes allow evaluation of operative risk, communication among physicians about a patient’s condition, and conduct of comparative studies of therapy on outcome. The modified Hunt and Hess grading scale is still the most commonly used, because of both familiarity and ease of application.
Grades | Criteria |
---|---|
0 | Unruptured aneurysm |
I | Asymptomatic or minimal headache and slight nuchal rigidity |
II | Moderate to severe headache, nuchal rigidity, but no neurologic deficit other than cranial nerve palsy |
III | Drowsiness, confusion, or mild focal deficit |
IV | Stupor, mild or severe hemiparesis, possible early decerebrate rigidity, vegetative disturbance |
V | Deep coma, decerebrate rigidity, moribund appearance |
∗ Serious systemic disease such as hypertension, diabetes, severe arteriosclerosis, chronic pulmonary disease, and severe vasospasm seen on arteriography result in assignment of the patient to the next less favorable category.
Grade | Glasgow Coma Scale Score | Motor Deficit |
---|---|---|
I | 15 | Absent |
II | 14–13 | Absent |
III | 14–13 | Present |
IV | 12–7 | Present or absent |
V | 6–3 | Present or absent |
Despite successful surgical treatment, delayed ischemic neurologic deficits (DIND) resulting in permanent neurologic injury or death can occur in patients in whom the complication vasospasm develops. Because the incidence and severity of vasospasm is related to the amount of subarachnoid blood present, computed tomography (CT) findings are often graded according to the Fisher’s grading system ( Table 13.3a and b ). Despite criticisms of Fisher grading and proposed modifications, it remains the primary method of describing the CT findings with regard to clot burden and the risk of vasospasm in aneurysmal SAH.
Grade | CT Finding(s) |
---|---|
1 | No blood detected |
2 | Diffuse thin layer of subarachnoid blood (vertical layers < 1 mm thick) |
3 | Localized clot or thick layer of subarachnoid blood (vertical layers ≥ 1 mm thick) |
4 | Intracerebral or intraventricular blood with diffuse or no subarachnoid blood |
No SAH | Focal or diffuse thin SAH | Focal or diffuse thick SAH | IVH | ||
---|---|---|---|---|---|
0 | + | – | – | – | No subarachnoid hemorrhage (SAH); no intraventricular blood |
1 | – | + | – | – | Thin diffuse or focal subarachnoid blood but no intraventricular blood |
2 | – | + | – | + | Thin diffuse or local subarachnoid blood with intraventricular blood |
3 | – | – | + | – | Thick focal or diffuse subarachnoid blood but no intraventricular blood |
4 | – | – | + | + | Thick local or diffuse subarachnoid blood with intraventricular blood |
Although the surgical mortality and morbidity vary with different institutions, patients in good preoperative condition (assigned to clinical grades I and II) can be expected to do well; patients with grade V status have a high mortality and morbidity, but aggressive management has resulted in substantial improvement ( Table 13.4 ). The clinical grade also indicates the severity of associated cerebral pathophysiology. The higher the clinical grade, the more likely the occurrence of vasospasm, elevated ICP, , impairment of cerebral autoregulation, , and a disordered cerebrovascular response to hypocapnia. A worse clinical grade is also associated with a higher incidence of cardiac arrhythmia and myocardial dysfunction. ,
Grade (Hunt and Hess) | Mortality (%) | Morbidity (%) |
---|---|---|
0 | 0–2 | 0–2 |
I | 2–5 | 0–2 |
II | 5–10 | 7 |
III | 5–10 | 25 |
IV | 20–30 | 25 |
V | 30–40 | 35–40 |
∗ Pooled from the literature and experience in the author’s (A.M.L.) institution.
Patients with worse clinical grades have a tendency to become hypovolemic and hyponatremic. , Thus, understanding the grading scale allows the anesthesiologist to communicate effectively with other physicians and facilitates assessment of pathophysiologic derangements and the planning of perioperative anesthetic management.
Intracranial Pressure
ICP increases rapidly after an SAH and may approach the levels of the systemic blood pressure. This phase lasts minutes and is thought to limit the amount of blood leakage through the ruptured aneurysm. With recurrent rupture of the aneurysm, ICP increases further from mass effect (clot), cerebral edema, or hydrocephalus due to a blocked aqueduct. ICP that reaches at least 20 mmHg is present in 36–67% of patients. ,
ICP correlates well with clinical grade. It is generally normal in patients with grade I and II status but is elevated in those with grade IV and V status ( Fig. 13.1 ). However, a normal ICP does not necessarily imply normal intracranial compliance (elastance). It is important not to normalize the ICP too rapidly, because doing so may increase the transmural pressure (TMP) gradient across the aneurysm wall and cause further hemorrhage. A cerebral perfusion pressure (CPP) value of 60 to 80 mmHg is a reasonable goal.

A communicating hydrocephalus may later develop because of arachnoidal adhesions from the extravascular blood that interferes with reabsorption of cerebrospinal fluid (CSF). In several large studies the incidence of hydrocephalus ranged from 15% to 41%. Clinically, hydrocephalus is characterized by progressive obtundation and nonreactive small pupils. The clinical features are present in only about 50% of cases, and therefore radiologic diagnosis is essential. Elevated ICP with hypovolemia may increase the likelihood of delayed cerebral ischemia and infarction. Patients with SAH generally have decreased cerebral blood flow (CBF) and cerebral metabolic rate. , The development of vasospasm can also exacerbate a rise in ICP because the reduction in CBF resulting from vasoconstriction of large conductance vessels is accompanied by vasodilation in the distal vessels, leading to an increase in cerebral blood volume (CBV) and a subsequent rise in ICP. Another factor that would contribute to increases in ICP is an intracerebral (17%) or intraventricular (17%) hematoma. It is possible that there is a causal relationship between acute hydrocephalus and delayed cerebral ischemia, but this remains to be established.
Impairment of Autoregulation and Carbon Dioxide Reactivity
Patients with SAH have both an impairment of autoregulatory capacity and a rightward shift in the lower limit of autoregulation. The severity of autoregulatory impairment correlates directly with the clinical grade. , , Nornes and colleagues observed that the lower limit of autoregulation was significantly higher in patients with clinical grade III than in those with clinical grade I and II during intracranial surgery. The development of impaired autoregulation closely correlates with the occurrence of vasospasm. This impaired autoregulation in the presence of vasospasm predicts delayed ischemic deficits and is associated with an unfavorable outcome.
If neurologic deterioration occurs, it is vital to review the hemodynamic measurements for any associated relationship. Many well-documented cases describe patients with SAH in whom a new neurologic deficit developed in association with a decrease in blood pressure and a subsequent reversal of the deficit with a pharmacologically induced increase in blood pressure. Thus, the anesthesiologist must not allow the perfusion pressure to decrease below this lower limit perioperatively. This situation represents a relative contraindication to induced hypotension during surgery, as discussed later. One study looked at outcome in relation to the incidence and magnitude of decrease in blood pressure and failed to find any difference in poor outcomes. While seemingly tolerated, induced hypotension may predispose to inadequate CPP values as well as exacerbate elevated ICP, and so is best avoided.
The cerebrovascular response to hyperventilation is generally preserved after SAH. , Although impairment of autoregulation may occur in patients assigned relatively good clinical grades, a decline in CO 2 reactivity does not occur until there is severe damage. Thus, hyperventilation remains effective in reducing CBF and CBV during perioperative management for most patients and potentially could improve autoregulation in those compromised by the SAH.
Systemic Effects
Intravascular Volume Status and Hyponatremia
The intravascular volume status has been found to be abnormally low in 36% to 100% of patients with SAH, and the level of hypovolemia correlates with the clinical grade. , Moreover, patients with signs of increased ICP on CT scan have a greater likelihood of systemic hypovolemia. The reasons are multifactorial and probably include bed rest, supine diuresis, negative nitrogen balance, decreased erythropoiesis, and iatrogenic blood loss. Hypovolemia may exacerbate the clinical effects of vasospasm and is associated with cerebral ischemia and infarction. , ,
Paradoxically, hypovolemia has often been observed to be associated with hyponatremia, which occurs in approximately 30–57% of cases of SAH. The etiology of hyponatremia is still a matter of debate. The syndrome of inappropriate antidiuretic hormone (SIADH) secretion has been implicated, treatment for which consists of fluid restriction. An alternative etiology is cerebral salt wasting syndrome (CSWS), which involves natriuretic peptides being released from the walls of the myocardium or from the hypothalamus in response to hydrocephalus, which causes distention of the cerebral ventricles. The release of brain natriuretic peptide has been correlated with cerebral vasospasm and hyponatremia , as well as cerebral infarction independent of vasospasm. What distinguishes CSWS from SIADH is the difference in intravascular volume, which is relatively low with the former and high in the latter. As hypovolemia can aggravate vasospasm, it is probably safer to err on the hypervolemic side, although normovolemia should be the goal. Hypertonic saline is the treatment of choice for both conditions.
Other significant electrolyte abnormalities are hypokalemia and hypocalcemia. In a series of 406 patients with SAH, both hypokalemia (serum K + value < 3.4 mmol/L) and hypocalcemia (serum Ca 2 + value 2.2 mmol/L) were noted (in 41% and 74% of cases, respectively).
Cardiac Effects
The effects of SAH on the myocardium can range from alterations in the electrocardiogram, to leakage of cardiac troponins, to wall motion abnormalities evident on echocardiography. Preexisting coronary artery disease in the setting of the profound stress induced by SAH may result in myocardial ischemia. However, the majority of patients with cardiac dysfunction secondary to SAH have normal coronary artery anatomy and suffer from a neurogenic stressed myocardium.
Electrocardiographic Changes
Electrocardiographic (ECG) abnormalities occur in 40–100% of patients with SAH. These abnormalities include sinus bradycardia, sinus tachycardia, atrioventricular dissociation, and bradycardia-tachycardia, in addition to more serious and potentially life-threatening rhythms such as ventricular tachycardia and fibrillation. Morphologic changes in the ECG tracing include T-wave inversion, depression of the ST segment, the appearance of U waves, prolonged QT interval, and, rarely, Q waves. A prolonged QT interval may occur in 20–41% of patients predisposing them to dangerous ventricular arrhythmias. Atrial arrhythmias including fibrillation and flutter occur in 4% of patients and have been found to be associated with a higher risk of severe disability and death. The incidence of ECG changes correlates with the amount of intracranial blood, and patients with Fisher grades 3 and 4 CT findings having more abnormalities. Potassium and calcium abnormalities may contribute to the ECG changes.
Myocardial Function
After SAH, damage to the myocardium can be indicated by an increase in circulating levels of cardiac troponin I (cTi) found in 17% to 68% of patients. , This cTi elevation following SAH-induced myocardial insult is much less than that related to myocardial infarction. Elevation of cTi has been found to be associated with regional wall motion abnormalities and left ventricular dysfunction, as well as hypotension, delayed cerebral ischemia from vasospasm, and death and disability at 90 days. ,
Echocardiography of patients with SAH has shown depressed left ventricular function and regional wall motion abnormalities in 13–18% of cases. , Predictors of ventricular dysfunction include elevated cTi, , , poor clinical grade (Hunt and Hess III to V), , and female gender.
The mechanism of myocardial dysfunction has garnered much attention lately, leading to the proposal of several mechanisms. The arterial supply to the heart has been implicated. Multivessel coronary artery spasm seems unlikely, given that the available coronary angiographic studies demonstrate normal coronary structure even in the presence of ongoing ECG and echocardiographic evidence of myocardial dysfunction. , Currently the favored mechanism is increased release of localized catecholamines in the myocardium. This intense stimulation leads to contraction band necrosis and subsequent myocardial dysfunction. The fact that regional wall motion abnormalities span the myocardium in a pattern outside the territories of known coronary artery distributions lends credence to this theory.
Takotsubo cardiomyopathy (TTC) has been reported in aneurysmal subarachnoid hemorrhage, but typically, ventricular dysfunction secondary to SAH is associated with apical sparing. In a multicenter study on patients with SAH, neurogenic stress cardiomyopathy consistent with TTC was observed in 10% of the cases. Although the prognosis of SAH-induced ventricular dysfunction is good and generally considered reversible, it is associated with increased risk for delayed cerebral ischemia and poor outcome in this study.
Anesthetic Implications
Patients with prolonged QT interval, T-wave abnormalities, and Q waves should undergo prompt correction of electrolyte disturbances. Experimentally, pharmacologic or surgical blockade of the sympathetic nervous system prevents or abolishes these ECG changes. However, no evidence exists that the prophylactic administration of a β-adrenergic or other autonomic antagonist significantly alters the outcome in such patients, and the use of these agents for this purpose is probably not warranted.
Q waves and other ECG evidence of ischemia are always worrisome and, when observed in a patient with SAH, pose a diagnostic dilemma. Although most ECG abnormalities after SAH appear to be neurogenic rather than cardiogenic in nature, diagnostic difficulty has on occasion led to a delay in surgery. Moreover, microscopic hemorrhages and myocytolysis have been observed in a postmortem study, although other studies have reported no signs of myocardial damage.
The three possibilities for ECG abnormalities consistent with infarction are: (1) coincidental acute myocardial infarction, (2) SAH-induced myocardial infarction, and (3) ECG changes without infarction. Cardiac enzyme measurements and echocardiography should be obtained in suspicious cases. As with any surgical procedure, the decision to proceed with surgery should be based on a risk–benefit analysis and, therefore, depends on the urgency of the situation. Because of the risk of rebleeding, surgical therapy of a ruptured aneurysm is almost always considered urgent.
In summary, ECG changes are prevalent after SAH and likely represent hyperactivity of the sympathetic system with increased levels of norepinephrine. Although in some patients there is no myocardial pathologic condition, in others there may be ventricular dysfunction, and in rare cases necrosis and other myopathology can occur. In suspicious cases, serial cTi measurements should be obtained. Because the electrocardiographic changes reflect the severity of neurologic damage and have not been shown to materially contribute to perioperative mortality or morbidity, the decision to operate should not be influenced by these ECG changes. These considerations, however, may influence the decision about the choice of invasive monitoring. The various ECG changes and their potential correlation with ventricular dysfunction and pathology are summarized in Table 13.5 .
Benign changes | Sinus bradycardia |
Sinus tachycardia | |
Atrioventricular dissociation | |
Premature ventricular contractions | |
Nonspecific ST segment depression | |
T wave inversion | |
U wave | |
Possible and actual wall motion abnormalities | Symmetrical T wave inversion , Prolonged QT interval > 500 msec |
ST segment elevation | |
Left ventricular dysfunction with apical sparing | |
Regional wall motion abnormalities | |
Possible and actual myocardial injury | Q wave ST segment elevation |
Elevated myocardial enzyme values | |
Elevated troponin I value |
Respiratory System
Pulmonary edema has been observed to accompany SAH in 8% to 28% of cases , leading to an acute lung injury (PaO2-FiO2 ration < 300) in 27%. This may be due to pulmonary congestion from myocardial dysfunction or directly mediated at the lung via a sympathetic mechanism, and an inflammatory component has also been implicated. Parallel to myocardial dysfunction, the incidence of pulmonary edema is correlated closely with clinical grade. Aspiration and hydrostatic pneumonia are other potential complications.
Other Major Medical Problems
On the basis of the findings of the Cooperative Study, other major medical problems associated with SAH are systemic hypertension (21%), heart disease (3%), and diabetes mellitus (2%).
Concurrent Medical Treatments
Patients receiving diuretic therapy for chronic hypertension may have preexisting fluid and electrolyte problems before SAH.
Anticonvulsant medications such as phenytoin and carbamazepine antagonize the actions of nondepolarizing agents such as pancuronium and vecuronium (and atracurium to a lesser extent) as well as fentanyl requirements. A higher dose requirement and shortened duration of action for these drugs might be anticipated if the anticonvulsant therapy has been greater than 7 days in duration. The effect of the newer anticonvulsants lamotrigine and levetiracetam on nondepolarizing agents has not been rigorously studied. However, preliminary data indicate that higher doses are not needed and shortened duration of neuromuscular blockade does not occur.
Antifibrinolytic agents such as ε-aminocaproic acid and tranexamic acid to prevent rebleeding while the patient is waiting for surgery have been evaluated. Although these agents have been found to decrease the risk of rebleeding, they are associated with an increased risk of cerebral ischemia, and no overall benefit has been shown for their use. The anesthesiologist should be aware that patients on antifibrinolytics may have a greater incidence of vasospasm and hydrocephalus, and have a higher incidence of venous thrombosis and pulmonary embolism. Thus, the usage of antifibrinolytics cannot be recommended. Use of recombinant factor VII (rFVIIIa) to decrease the incidence of rebleeding has been deterred by the occurrence of critical thrombosis in an early trial.
Calcium channel antagonists (usually oral nimodipine) should be routinely administered for vasospasm prophylaxis. This therapy has specific anesthetic implications that are discussed later in the chapter.
Timing of Surgery
The two major complications contributing to significant morbidity and mortality after SAH are rebleeding and vasospasm, with each accounting for about 7% of mortality. Because the brain is acutely swollen with fresh clots following SAH, it was generally believed that early operation increases the incidence of postoperative vasospasm. The Cooperative Study data influenced most surgeons to wait 7–10 days for the acute inflammatory process to subside before any operative intervention. Indeed, results from the Cooperative Study indicate that the brain was considered “tight” in 50% of cases with early surgery (same day as SAH), but in only 20% of cases undergoing operation after 10 days ( Fig. 13.2 ). Although delaying surgery does allow brain swelling to decrease, both rebleeding and vasospasm can occur during this waiting period. When surgery was delayed, antifibrinolytic agents were usually given to prevent lysis of the clot and rebleeding from the rent of the aneurysm. However, randomized clinical trials showed that, although these agents are effective in reducing the incidence of rebleeding, the incidence of vasospasm increases, leaving the overall morbidity and mortality unchanged.

Outcome studies to guide practice in timing of surgery are lacking. While observational studies favor earlier surgery—with poor grade patients seemingly having the most benefit from early surgery , there is variation in when patients with poor clinical grade are taken to surgery. In an attempt to improve the overall outcome, there is a growing trend toward early operation, so that the risk of rebleeding can be eliminated and any vasospasm more aggressively treated. However, the results from the Cooperative Study showed that the overall management results for early (3 days or sooner) and late (after 10 days) surgery are not significantly different, although the results were the worst when surgery was performed between 7 and 10 days. A subsequent analysis of data derived from only North American centers varied from the overall findings and indicated that the best results were achieved when surgery was planned within 3 days of SAH, therefore arguing strongly in favor of early surgery. Although they should never be the primary consideration, substantial economic savings are also realized with early surgery. The trend toward early operation will probably continue, and patients in less-than-ideal condition for surgery will be coming to the operating room.
Rebleeding
Previous studies suggest that rebleeding following the initial SAH peaks at the end of the first week. The Cooperative Study data indicate that rebleeding peaks at 4% during the first 24 hours and then levels off at 1.5% per day on subsequent days. The overall incidence is 11%, , which accounts for 8% of the mortality and disability. The incidence is lower in patients receiving antifibrinolytic agents. Rebleeding remains a major threat in hospitals where delayed surgery is the standard practice. With the trend toward early operation, the risk of rebleeding is reduced but not eliminated. A combination of antifibrinolytic therapy for rebleeding and a calcium channel blocker for vasospasm has been suggested as a possible remedy.
Vasospasm
Incidence
In patients who initially survive a SAH, cerebral vasospasm causing ischemia or infarction remains an important cause of morbidity and mortality. In the Cooperative Study, vasospasm accounted for 13.5% of the overall mortality and major morbidity. Not all patients with SAH have vasospasm, and the severity, time course, and prognosis of vasospasm are largely unpredictable. The incidence and severity of delayed cerebral vasospasm have been shown to correlate with the amount and location of blood in the basal cisterns. The frequency of occurrence as determined by angiography is estimated to be 40–60%. However, clinically significant and symptomatic vasospasm occurs at a lower frequency (20–30%). The difference may be explained by the varying severity of vasospasm. Significant narrowing of the major blood vessels can occur without an overall reduction in distal perfusion. Furthermore, CBF can be reduced significantly by 20–25% without compromising normal brain function. Thus, considerable reduction in CBF can occur from vasospasm without clinical symptoms. Of the patients in whom symptomatic vasospasm develops, approximately 50% die or are left with a serious residual neurologic deficit. Typically, angiographically detectable vasospasm is not seen until 72 hours after SAH, the incidence peaks 7 days after SAH, and the problem is seldom seen after 2 weeks. When comparing endovascular aneurysm therapy and surgical clipping of the aneurysm with regards to vasospasm—endovascular therapy appears to have either the same or reduced incidence of vasospasm.
Pathogenesis
The vasospastic artery has structural and pathologic changes within the vessel wall, such as swelling and necrosis of the smooth muscle cells. Although the exact mechanism and cause of spasm have not been completely elucidated, a reasonable hypothesis is that one or more vasoactive substances contained in the blood in the basal cisterns induce inflammatory changes in the cerebral arteries to cause severe constriction. The component in the blood implicated in the pathogenesis of vasospasm is currently thought to be oxyhemoglobin. The normal cerebrovascular tone is regulated by a balance between vasodilating and vasoconstricting factors. The suppressive interaction of oxyhemoglobin with endothelium-derived relaxing factor or nitric oxide (potent vasodilator) coupled with stimulated production of endothelin (potent vasoconstrictor) is the postulated cause of cerebral vasospasm. In experimental vasospasm, the rise in perivascular concentrations of oxyhemoglobin and deoxyhemoglobin parallel the time course of vasospasm. The presence of hemoglobin in the extravascular space incites an inflammatory reaction including such molecules as free radicals, haptoglobin, cell-adhesion molecules, cytokines which act to recruit macrophages and leukocytes into the extravascular areas containing blood.
Clinical Manifestations
Delayed cerebral ischemia from vasospasm after SAH is a multivascular or diffuse process in most patients. The clinical manifestations of vasospasm include a decrease in the level of consciousness, new onset of focal signs, and mutism. In a prospective study, Hijdra and associates found that the majority of patients with delayed cerebral ischemia from vasospasm had a decrease in level of consciousness that was sometimes accompanied, but never preceded, by focal signs. Clinical manifestations most commonly appear gradually but may also occur abruptly.
Diagnosis
After the appearance of new focal signs or a decrease in level of consciousness, the diagnosis of cerebral vasospasm is confirmed by angiography. A CT scan may show hypodense lesions in brain areas that are consistent with the clinical signs; however, these have been found to be not sensitive and potentially unreliable. The diagnosis of vasospasm may also be predicted or made before the onset of clinical vasospasm with the use of transcranial Doppler ultrasonography (TCD). With vasospasm and narrowing of the conductance arteries of the circle of Willis, cerebral artery flow velocities increase, although confirmation with angiography is definitive. The noninvasive technology of TCD also allows continual evaluation of the patient in vasospasm without resorting to frequent angiographic investigations. However, in patients treated with hypertensive therapy, increase in flow velocity may represent increase in local cerebral blood flow instead of worsening vasospasm. , Radiologic confirmation with angiography, single-photon emission CT (SPECT), CT perfusion, or MR perfusion studies may be necessary. CT angiography and MR angiography have also been used to diagnose vasospasm, but the sensitivity and specificity have not been established. Digital subtraction angiography remains the gold standard for diagnosis of vasospasm.
Treatment
Pharmacologic
Numerous drugs have been investigated for the prevention or treatment of vasospasm, but most are ineffective. Calcium channel blockers, of which nimodipine has been most extensively studied, are the only class of drugs that have been shown to consistently reduce the morbidity and mortality from vasospasm. Depending on the study, the incidence of poor outcome is reduced by 40–70%. Interestingly, none of these studies with favorable results for calcium channel blocker prophylaxis was able to demonstrate any significant change in the incidence or severity of vasospasm, suggesting that the beneficial effects of nimodipine may be occurring at either a distal vessel site or a cellular level. The only study that demonstrated a significant improvement in angiographic vasospasm with a calcium channel blocker used fasudil hydrochloride (not approved for use in the United States). Its use has yielded clinical outcomes similar to those for nimodipine. High-dose intravenous nicardipine has been investigated for the prevention of vasospasm. Although the incidence of symptomatic vasospasm was reduced from 38% to 25%, there was no difference in outcome at 3 months, presumably because hypervolemic hypertensive therapy is effective in ameliorating the ischemic deficits from vasospasm.
Because cerebral vasospasm is frequently the cause of poor outcomes after successful surgical or endovascular treatment, there are many investigations looking for new pharmacologic therapies. Magnesium sulfate is thought to be a cerebral vasodilator by way of blocking voltage-dependent calcium channels. This property, paired with its ability to antagonize N -methyl- d -aspartate receptors, thereby limiting the damaging excitatory glutamate stimulation, has led to many preliminary studies. So far, magnesium sulfate has shown a similar efficacy in replacing nimodipine, and use of these two agents in conjunction has led to positive results in decreasing vasospasm and improving outcome. The enthusiasm, however, must be tempered by the concern for hypotension and hypocalcemia that accompany its use and studies have not supported its use. The endothelin receptor antagonists clazosentan has been shown to be effective in the prevention as well as he treatment of established vasospasm. However, a multicenter clinical trial failed to demonstrate a significant effect on mortality or morbidity, mainly secondary to an increase in lung complications, anemia and hypotension. The use of statins to increase endothelial nitric oxide and thus treat vasospasm has received interest. A meta-analysis of trials to date suggests that statins may reduce the incidence of delayed ischemic deficits but does not seem to have an effect on long-term outcome.
Several other medications have shown early promise but subsequently failed to be adopted into clinical use. Of these, tirilazad, a potent lipid peroxidation inhibitor, initially showed promise, but further studies and a meta-analysis failed to show benefit. Nicaraven, a hydroxyl radical scavenger, afforded a 35% reduction in the incidence of delayed ischemic deficits at 1 month; however, the results were no longer favorable at 3 months.
Nonpharmacologic
Surgical
The presence of subarachnoid blood is related to the occurrence of vasospasm both qualitatively and quantitatively. By operating on patients with SAH within 48 hours of hemorrhage, Taneda reduced the incidence of delayed ischemic deficits from 25% (11 of 44 patients who underwent surgery 10 days or more after the hemorrhage) to 11% (11 of 101 patients). Thus, early operation with extensive irrigation of the cisterns may have lowered the incidence or severity of vasospasm.
Reduction of Intracranial Pressure
If the patient has elevated ICP, cerebral perfusion may be improved by lowering of the ICP. Improvement in neurologic status with this treatment alone has been reported.
Hypervolemic, Hypertensive, and Hemodilution Therapy
Historically the most consistently effective regimen available to treat ischemic neurologic deficits due to cerebral vasospasm uses hypervolemia, hypertension, and hemodilution (triple-H therapy). The rationale behind induced hypervolemia and hypertension is that in SAH the ischemic areas of the brain have impaired autoregulation and thus CBF depends on perfusion pressure, which partly depends on the intravascular volume and mean arterial blood pressure. , Although the triple H therapy may still be practiced in some centers, the latest guideline essentially focuses solely on the hypertensive therapy as repeated studies suggest that hypervolemia provides no additional benefit and might increase the risk of pulmonary dysfunction.
This classic triple H-therapy or, currently, hypertensive therapy, is most successful if instituted early, when the neurologic deficits are mild and before the onset of infarction. However, prophylactic treatment initiated before aneurysm clipping is associated with a significant risk of rebleeding (19% in one series). Other concerns are worsening of cerebral edema, increasing ICP, and hemorrhage into an infarcted area. With early surgery there is less likelihood of rebleeding from the hypervolemic, hypertensive therapy. Other systemic complications are pulmonary edema (7–17%), myocardial infarction (2%), dilutional hyponatremia (3–35%), and coagulopathy (3%).
To optimize therapy and minimize the potential cardiovascular and pulmonary complications, the use of invasive blood pressure monitoring is essential. Central venous pressure (CVP) or pulmonary artery catheter (PAC) was once commonplace but is increasingly being replaced by less invasive equivalents, including transpulmonary thermodilution monitoring as well as algorithmic-based derivations of stroke volume variation and cardiac output. Because a growing number of studies have implicated PACs as increasing complication rates with no improvement in outcome, it is rare to place a PAC to guide triple-H therapy. Select cases where neurogenic cardiac stunning may complicate triple-H therapy may warrant the addition of a PAC to guide fluid therapy, although hypervolemia is no longer the goal.
Hypervolemia or, more appropriately, normovolemia is generally achieved with infusions of crystalloids or colloids when indicated (e.g., 5% albumin). Hetastarch and dextran solutions probably should not be used because of the potential complication of coagulopathy through interference with platelets and factor VIII. , Trumble and colleagues examined the use of hetastarch for hypervolemic treatment of vasospasm and reported the occurrence of coagulation abnormalities in all patients. Thus they were unable to formulate any dose guideline for its safe use. The lower-molecular-weight (LMW) dextran (pentastarch) appears to be associated with fewer coagulation problems. Although intravenous fluid loading alone is often effective, it is at times insufficient to raise the blood pressure or reverse ischemic symptoms; vasopressors are then initiated to induce hypertension. The most widely used vasopressors are dopamine, dobutamine, norepinephrine (noradrenaline), and phenylephrine. The hypertensive therapy may induce a vagal response as well as a profound diuresis, requiring administration of large amounts of intravenous fluids. Atropine (1 mg intramuscularly every 3 to 4 hours) may be given to maintain the heart rate between 80 and 120 beats/min, and aqueous vasopressin (Pitressin) (5 units intramuscularly) may be administered to maintain the urine output at less than 200 mL/h. With this regimen, often only small amounts of vasopressor drugs are required. The blood pressure is titrated to a level necessary to reverse the signs and symptoms of vasospasm or to a maximum of 160 to 200 mmHg systolic in the patient whose aneurysm has been clipped. If the aneurysm has not been clipped, the systolic blood pressure is increased to only 120 to 160 mmHg. Blood pressure management should not be affected by the presence of incidental unruptured aneurysms, as the risk of hemorrhage from unsecured, unruptured aneurysms during hypertensive therapy is very low. The elevated blood pressure must be maintained until the vasospasm resolves, usually in 3 to 7 days. Response to therapy can now be monitored noninvasively with TCD. Improvement in vasospasm is generally clinically evident, and may or may not be associated with a decrease in flow velocity and, more importantly, a decrease in the Lindegaard ratio (MCA to extracranial ICA flow velocity ratio). Hemodilution, the last component of the traditional triple-H therapy, is based on the correlation of hematocrit and whole blood viscosity. As the hematocrit and viscosity diminish, the cerebrovascular resistance correspondingly decreases and CBF increases. One argument against hemodilution is that oxygen-carrying capacity is also reduced. Experimental studies have suggested that a hematocrit of 33% provides an optimal balance between viscosity and oxygen-carrying capacity, and this value has been applied clinically. With the growing concern about increased morbidity and mortality related to blood transfusion, this value is now often revised downwards to between 25% and 30%. Active hemodilution is currently seldom used, as most patients are at the target concentration at the onset of vasospasm.
Transluminal Angioplasty
In all major vessels that are accessible, transluminal angioplasty has been used in patients with vasospasm refractory to conventional treatment, with reversal of deficits being achieved in 65% of the patients treated. The time frame within which to institute angioplastic therapy has not yet been established, but prophylaxis is not warranted because of the small but inherent risk of rupture. In distal vessels that are not accessible for angioplasty, the administration of vasodilators (nicardipine, verapamil, papaverine, milrinone) via superselective intra-arterial infusion has been shown to be effective.
Improvement in cerebral venous oxygen saturation and metabolic profile with this treatment has been demonstrated. However, studies have failed to show a definitive difference between angioplasty and papaverine injection. Although rare, complications from intra-arterial papaverine infusion include severe thrombocytopenia, increase in ICP, and transient brainstem dysfunction (when infused into the posterior circulation). Intraoperative cisternal injection of papaverine to reduce the risk of vasospasm has also been reported to cause transient pupillary dysfunction, which may interfere with neurologic assessment. The newer vasodilators used for intra-arterial injection seem to decrease these side effects, with nicardipine showing better results than verapamil. A reduction in systemic blood pressure has been reported with the use of nicardipine, milrinone, and verapamil Intraventricular use of nicardipine and intrathecal nimodipine in initial studies has shown some benefit in the treatment of vasospasm. Intraventricular administration of sodium nitroprusside has also been reported to reverse vasospasm refractory to conventional triple-H therapy. However, none of this is currently considered to be accepted therapy.
Anesthetic Considerations
Hypertensive Therapy
The anesthetic management of patients who have or are at risk for vasospasm requires an understanding of the natural course of vasospasm, concurrent therapy, the importance of intravascular volume status, the changes in electrolyte concentration that occur with the treatment of vasospasm, and which hemodynamic variables are associated with vasospasm. Although it is generally believed that early surgery raises the risk of vasospasm, there are no substantiating data, and some data suggest otherwise. , Further investigations suggest that the interplay of vasospasm and surgical timing might account for the severity of the bleeding.
Asymptomatic patients at risk for vasospasm include all patients who undergo surgery before the onset of vasospasm. Although it is not possible to predict the occurrence of postoperative vasospasm, patients with good SAH grades and low Fisher grade tend to have a lower incidence. These patients should be kept in a normovolemic state, with volume loading initiated toward the end of the operation, after the aneurysm has been clipped. Intraoperatively, controlled hypotension can be provided safely, if so requested by the surgeon. Treatment of postoperative hypertension should not be too aggressive.
Some physicians consider patients who are symptomatic of vasospasm as being at high risk and not eligible for surgery. Many other surgeons, however, believe the most effective treatment of vasospasm is immediate clipping of the aneurysm so that aggressive hypertensive and hypervolemic therapy can be implemented. Symptomatic patients presenting for emergency clipping of aneurysms should be treated aggressively, and normovolemia should be maintained and guided with invasive or noninvasive monitoring. For patients who have already been treated with hypertensive therapy, the threshold blood pressure below which the patient becomes symptomatic must be noted, and the blood pressure should not be allowed to fall below this value. Buckland and coworkers have advocated intraoperative induced hypertension as a prophylactic measure. This approach, however, must be balanced against the risk of re-rupture of the aneurysm as well as increased brain swelling and difficulty with intraoperative brain retraction. Because the main aim must be to maintain adequate CPP, induced hypotension is contraindicated in these patients.
With respect to asymptomatic patients who undergo operation on a delayed basis, vasospasm seldom occurs more than 12 days after SAH. Therefore patients who have surgery later than 10 to 12 days after SAH have a low risk of vasospasm and can be managed in a normal fashion.
Calcium Channel Antagonists
Calcium channel antagonists have a proven efficacy in reducing the neurologic complications of vasospasm. In most institutions, all patients with SAH are prophylactically treated with nimodipine. Clinical experience suggests that these drugs do not present any difficulty for anesthetic management, although 5% of the patients who receive nimodipine and 23% of the patients who receive intravenous nicardipine demonstrate mild hypotension as a result of systemic vasodilation. A similar tendency toward lower systemic blood pressure was also observed intraoperatively, and there was a reduced demand for hypotensive agents when controlled hypotension was used.
Premedication
To allow accurate assessment of the patient’s immediate preoperative neurologic condition and clinical grade, preoperative medications are best omitted. A proper preoperative visit by the anesthesiologist with a thorough explanation usually obviates any need for preoperative medication. However, an anxious patient may become hypertensive, with greater risk of rebleeding. On the other hand, premedications such as barbiturates and narcotics may cause respiratory depression, resulting in an increase in CBF and CBV; therefore such medication must be used judiciously in patients with elevated ICP. Premedication should be individualized. Patients with a good clinical grade may receive fentanyl, 50–150 μg or morphine, 1–5 mg, and/or midazolam, 1–5 mg, intravenously for sedation. In general, premedication should be conservative, and narcotics are preferable to benzodiazepines. The best results are achieved when administration is titrated incrementally. Patients already treated with mechanical ventilation may receive higher doses (fentanyl 250–500 μg or morphine, 10–20 mg; midazolam, 5–10 mg) if hemodynamic stability is maintained. Muscle relaxants may also be required for transport of intubated patients. Patients should continue to receive their regular doses of nimodipine and dexamethasone.
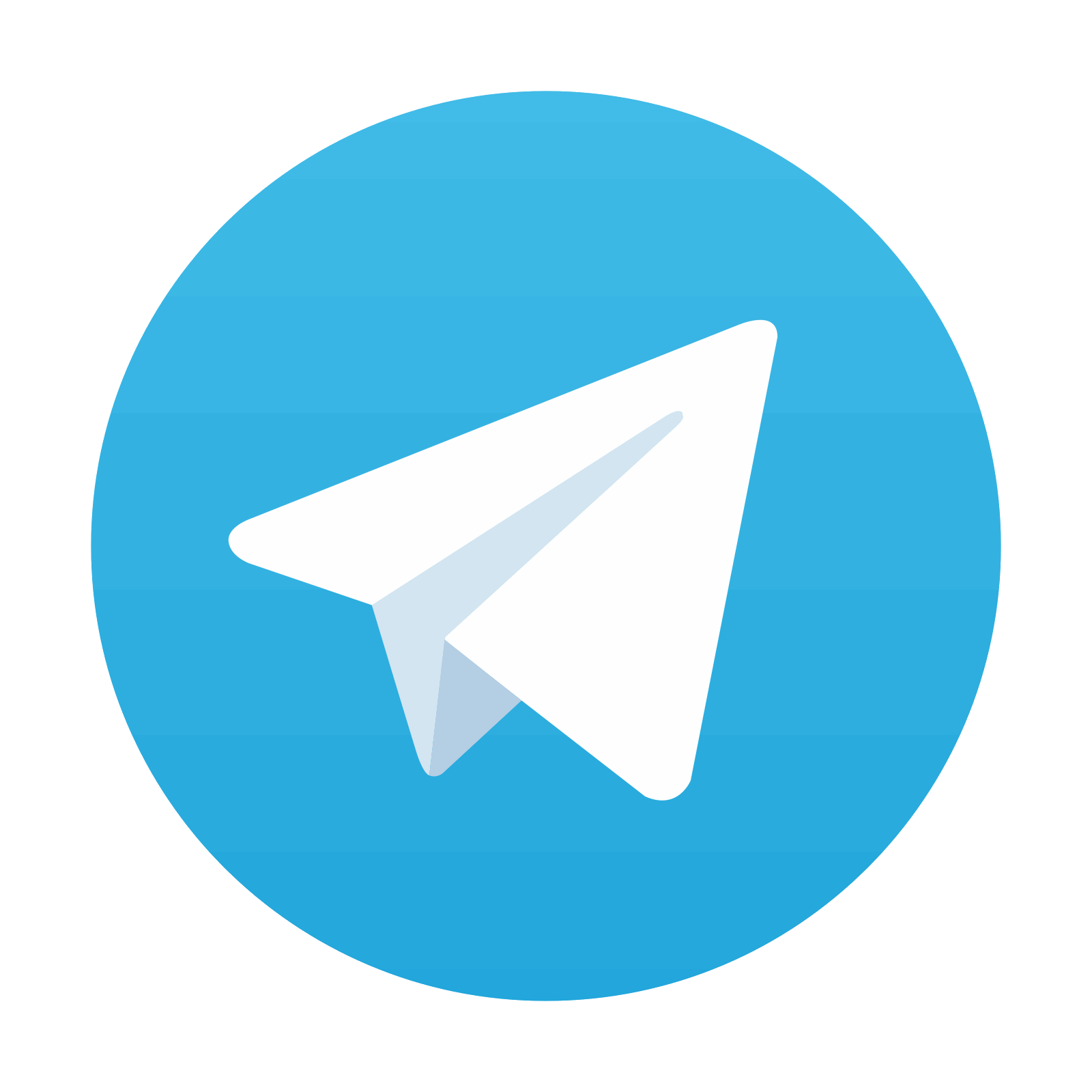
Stay updated, free articles. Join our Telegram channel

Full access? Get Clinical Tree
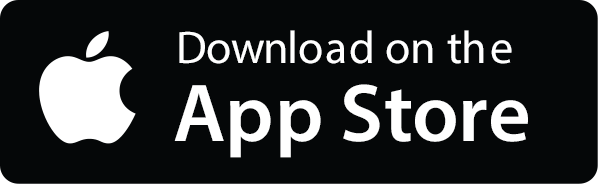
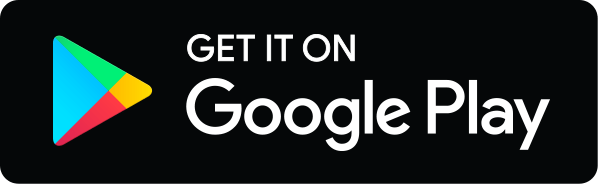