Heart failure is increasingly being recognized as a growing health problem worldwide. It is estimated that the lifetime risk of developing heart failure is approximately 20% for patients older than 40 years.1 Clinical anesthesiologists can therefore expect to see cases involving patients suffering from heart failure with increasing frequency. Symptomatic heart failure is associated with high risk of morbidity and mortality as a result of perioperative ischemia/infarction, dysrhythmias, worsening heart failure, postoperative cognitive dysfunction, and stroke.2,3 Asymptomatic left ventricular dysfunction, which is considered a precursor of symptomatic heart failure and is assumed to have similar prevalence as symptomatic heart failure, is also associated with high perioperative mortality.4 Since most anesthetics interfere with cardiovascular performance, either by direct myocardial depression or by depression of sympathetic activity, on which these patients rely, an appropriate anesthetic technique must be selected to minimize hemodynamic changes and maintain a near-normal physiologic status. This chapter discusses the aspects of dose reduction, titration of drugs, and the pharmacodynamic effects of each class of anesthetic drugs. As an example, the chapter provides a practical guide to the selection and use of general anesthetic agents in patients with poor cardiac function undergoing colectomy and discusses the pharmacologic management of acute cardiac deterioration.
HEART FAILURE: DEFINITION, PATHOPHYSIOLOGIC CHANGES, AND THEIR PHARMACOKINETIC IMPLICATIONS
Heart failure is a complex clinical syndrome, but the basic problem is the heart’s inability to pump blood at a rate commensurate with the requirements of the metabolizing tissues or ability to do so only at elevated filling pressures. It can be broadly subdivided into 2 distinct forms. The first form, termed diastolic dysfunction, and is due to inadequate ventricular relaxation preventing adequate end-diastolic filling.5,6 The second, termed systolic dysfunction, is due to inadequate force generation to eject blood normally. Therefore, it is important to be aware of the influence of anesthetics on both systolic and diastolic dysfunction.
In the failing ventricle, various adaptive mechanisms are initiated to help maintain arterial pressure and cardiac output (CO). The body activates several neurohumoral pathways to increase circulating blood volume. The sympathetic nervous system increases heart rate and contractility, causes arteriolar vasoconstriction in nonessential vascular beds, and stimulates secretion of renin from the juxtaglomerular apparatus of the kidney. Stimulation of the renin–angiotensin system results in further arteriolar vasoconstriction, sodium and water retention, and release of aldosterone. The increased aldosterone, in turn, also leads to sodium and water retention. Additionally, baroreceptor and osmotic stimuli lead to vasopressin release from the hypothalamus, causing reabsorption of water in the renal collecting duct. Although these neurohumoral pathways initially are beneficial, eventually they become deleterious and aggravate ischemia, potentiate dysrhythmias, cause endothelial dysfunction, promote cardiac remodeling, and are directly toxic to myocytes (Figure 22–1).
The pathophysiologic changes that occur in heart failure result in hepatic, gastrointestinal, and renal congestion, as well as hypoperfusion. These changes directly alter drug pharmacokinetics (absorption, distribution, metabolism, and excretion).7
Liver function abnormalities are common. There is impairment of hepatic microsomal function, which affects drugs with low a hepatic extraction ratio, such as chlordiazepoxide, diazepam, lorazepam, methadone, and pentobarbital.
Activation of the renin–angiotensin system leads to vasoconstriction of the efferent arterioles, thereby maintaining glomerular capillary pressure and preserving glomerular filtration rate despite severe impairment of renal perfusion. Hepatic blood flow and enzymatic activity are more markedly reduced than renal function. Because anesthetic agents are primarily metabolized in the liver, drug toxicity with anesthetics occurs more readily in patients with heart failure.
It might be expected that the volume of distribution of drugs is increased in congestive heart failure as the condition is associated with fluid retention. However, this is not the case, because excess fluid is retained interstitially and is not in direct communication with the cardiovascular system.8 In fact, patients may have relative intravascular volume depletion resulting in higher drug concentrations after a loading dose of a drug.
Heart failure can lead to changes in the elimination half-life. The changes are a function of alterations in drug clearance and/or volume of distribution. Elimination half-life of agents that have a large volume of distribution and are highly cleared by the liver (ie, fentanyl, morphine, ketamine) may be twice as long in patients with heart failure compared to otherwise healthy patients. For example, in a simulation of fentanyl bolus (Figure 22–2), a doubling of the elimination half-life slows the decline in plasma and effect-site concentrations, prolonging the effect. As illustrated in the top plot, the duration of effect, assuming an analgesic threshold for fentanyl of 1 ng/mL is extended from 31 to 48 minutes for a 2-mcg/kg bolus. A slower elimination half-life has an even more pronounced impact on continuous fentanyl infusions. As illustrated in the bottom plot, the duration of effect is almost doubled, going from 48 to 90 minutes for a 1-hour fentanyl infusion dose at 3 mcg/kg/h. As with all simulations, they are based on mathematical equations and not measured drug concentrations. They are primarily used to illustrate a point, in this case decreased elimination. Estimates presented in these simulations are likely to have considerable variability in patients with poor cardiac function.
Figure 22–2
Simulations of a fentanyl bolus (top plot) and 1-hour infusion (bottom plot) administered to a 75-kg male with prolonged (dark red lines) and normal (red lines) elimination. Prolonged elimination was defined as a doubled elimination half-life. The gray line represents the effect-site concentration (Ce) threshold for analgesia.

This section will describe the effects of the changes in pharmacokinetic parameters that result from cardiac failure on the clinical profiles of the most common anesthetic drugs. Effects of these drugs on the failing heart are described in the next section.
Variation in CO has limited effect on the rate of rise of alveolar–to–inspired concentration ratio of the anesthetic (FA/FI) during the initial transfer of sparingly soluble agents (desflurane, sevoflurane, and nitrous oxide) as the rate of rise in FA/FI for these agents is not affected much by local alveolar perfusion. A decreased CO is likely to cause a concentrating effect9 where a significant fraction of the inspired anesthetic is transported into the blood for soluble anesthetic agents (isoflurane, enflurane, and halothane). For example, consider the difference in the isoflurane, sevoflurane, and desflurane uptake for a CO of 6 L/min compared to 1.5 L/min during a 1-hour administration (Figure 22–3). These simulations illustrate how the more soluble agent (isoflurane) leads to a higher uptake over the duration of the 1-hour administration in comparison to less soluble agents (sevoflurane and desflurane).
Figure 22–3
Anesthetic uptake for sevoflurane, desflurane, and isoflurane under normal and low cardiac output states. [With permission of the owner, James H. Philip, and the licensee, Med Man Simulations, Inc. (www.gasmanweb.com), a nonprofit charitable organization, PO Box 67160, Chestnut Hill MA 02467. Gas Man is a registered trademark of James H. Philip and Med Man Simulations, Inc., a nonprofit charitable organization.]

In this case, arterial anesthetic partial pressure would be doubled. The doubling in anesthetic concentration would mean ultimately that the brain will “see” double the number of anesthetic molecules transferring in, and so there is a greater likelihood of anesthetic-mediated depression of the central nervous system. Also, the increase in anesthetic concentration causes further reduction in CO by depressing contractility and slowing the heart rate. An established vicious cycle can cause a potentially lethal increase in alveolar concentration. Therefore, in order to manage patients who exhibit ventricular dysfunction, agents with relatively low blood/gas solubility might be preferable, as the alveolar concentration of these agents would not be especially sensitive to cardiopulmonary changes. If more soluble agents are used, then they must be administered using gradual increments and their end-tidal concentrations should be monitored.
The morphologic and functional liver changes caused by the reduced blood flow to the hepatocytes in patients with poor cardiac function lead to alterations in the pharmacokinetics of midazolam; these are reflected in the prolonged plasma half-life and increased serum levels of its metabolically active molecules as a result of the deceleration of its systemic clearance.7 This deceleration results from a reduction in hydroxylation process which results in prolonged and more intense pharmacologic effects. Specifically, the half-life is prolonged by 50% and clearance is lowered by 32%.10 Studies show that a 14.3% reduction in sedative dose of midazolam is required in patients with ejection fraction less than 55% to achieve the desired clinical effects without incurring toxic effects.11
Although experimental evidence supports an influence of CO on kinetics, the number of drugs studied has been limited. Propofol is highly lipid soluble, has a very large volume of distribution, redistributes rapidly, and is eliminated via both hepatic and extrahepatic routes. In patients with ventricular dysfunction, the central volume of distribution is decreased, and both systemic and intercompartmental clearance is reduced. Thus, initial arterial concentration after intravenous bolus administration of propofol is particularly high, and greater adverse hemodynamic effects can be expected if a normal dose of propofol is injected into patients with a low CO.12 Bolus doses should therefore be reduced when propofol is used for induction in patients with poor cardiac function. Also, the hemodynamic instability can be minimized if the bolus is given in a titrated manner over a longer period of time and laryngoscopy performed after reaching a pharmacodynamic end point, such as a bispectral index (BIS) less than 40.13 However, this has been contradicted by a recent study, which found that the pharmacokinetic model significantly underestimates the plasma propofol concentration in patients with impaired left ventricular (LV) function. The predictive value of BIS for the desired level of sedation was insufficient in this patient cohort.14 These findings suggest that widely used models of propofol kinetics are mis-specified when cardiac function is depressed, and in this setting they should not be used to formulate dosages or drive a target-controlled infusion pump.
Dose adjustments are also required during continuous infusion for the maintenance phase of an anesthetic; it has been shown in a swine model that a 42% decrease in CO from baseline results in 70% increase in propofol concentrations from baseline during a continuous infusion of propofol at 6 mg/kg/h.15 This, combined with a prolonged context-sensitive half-time, can result in delayed emergence from anesthesia unless the dose is reduced during prolonged infusions.
Patients with poor cardiac function appear to have loss of eyelash reflex at a lower concentration of thiopental.16,17 This increased sensitivity of patients with heart failure to thiopental is caused by a decreased central volume of distribution, resulting in higher effect-site concentration. In addition, the effect-site concentration of thiopental equilibrates faster with the arterial blood concentration. The faster rise observed in patients with low CO is a consequence of the slower distribution to the periphery. What is more worrisome is that the same would happen in the tissues of the cardiovascular system, leading to a more pronounced depression of the cardiovascular system in an already compromised patient. Therefore, extra caution should be exercised when inducing anesthesia with thiopental in such patients. The effect of thiopental is also prolonged because of slower elimination secondary to a decreased hepatic blood flow.
When opioids are used as an integral part of anesthesia (rather than for postoperative analgesia), patients with significant myocardial dysfunction appear to require lower doses. Due to a decrease in liver blood flow consequent to decreased CO and reduced plasma clearance, these patients develop higher plasma and brain concentrations for a given loading dose or infusion rate than do patients with adequate ventricular function. This may lead to decreased sympathetic nervous system activity, on which these patients are dependent, and result in hypotension, which may be misinterpreted as hypovolemia. This is important because they can be erroneously given fluid to restore systemic blood pressure and subsequently accumulate extravascular water. As sympathetic tone and systemic vascular resistance (SVR) return toward normal in the postoperative period, they may then develop pulmonary edema.18
This section describes the cardiovascular effects of the most commonly used anesthetic drugs in the setting of cardiac failure.
Early in vitro studies demonstrated that volatile anesthetics cause relatively greater decreases in contractility in failing versus normal isolated myocardium,19,20 and 21 suggesting that patients with underlying contractile dysfunction may be more sensitive to the myocardial depressant properties of volatile anesthetics. However, further studies demonstrated that volatile anesthetics may affect LV function by producing favorable alterations in loading conditions and diastolic performance in the presence of LV dysfunction.21,22,23 and 24 Isoflurane, but not halothane, improved indices of diastolic performance in dogs with pacing-induced LV dysfunction, despite producing simultaneous negative inotropic effects.22 These findings were attributed to favorable reductions in LV preload and not to direct lusitropic effects.22 They concluded that improvement of filling dynamics may partially offset the decrement in LV systolic function by isoflurane in the setting of LV dysfunction. Desflurane and sevoflurane were also shown to exhibit similar moderate beneficial actions on LV diastolic function, in the presence of severe abnormalities in systolic and diastolic functions during myocardial ischemia in dogs.24,25
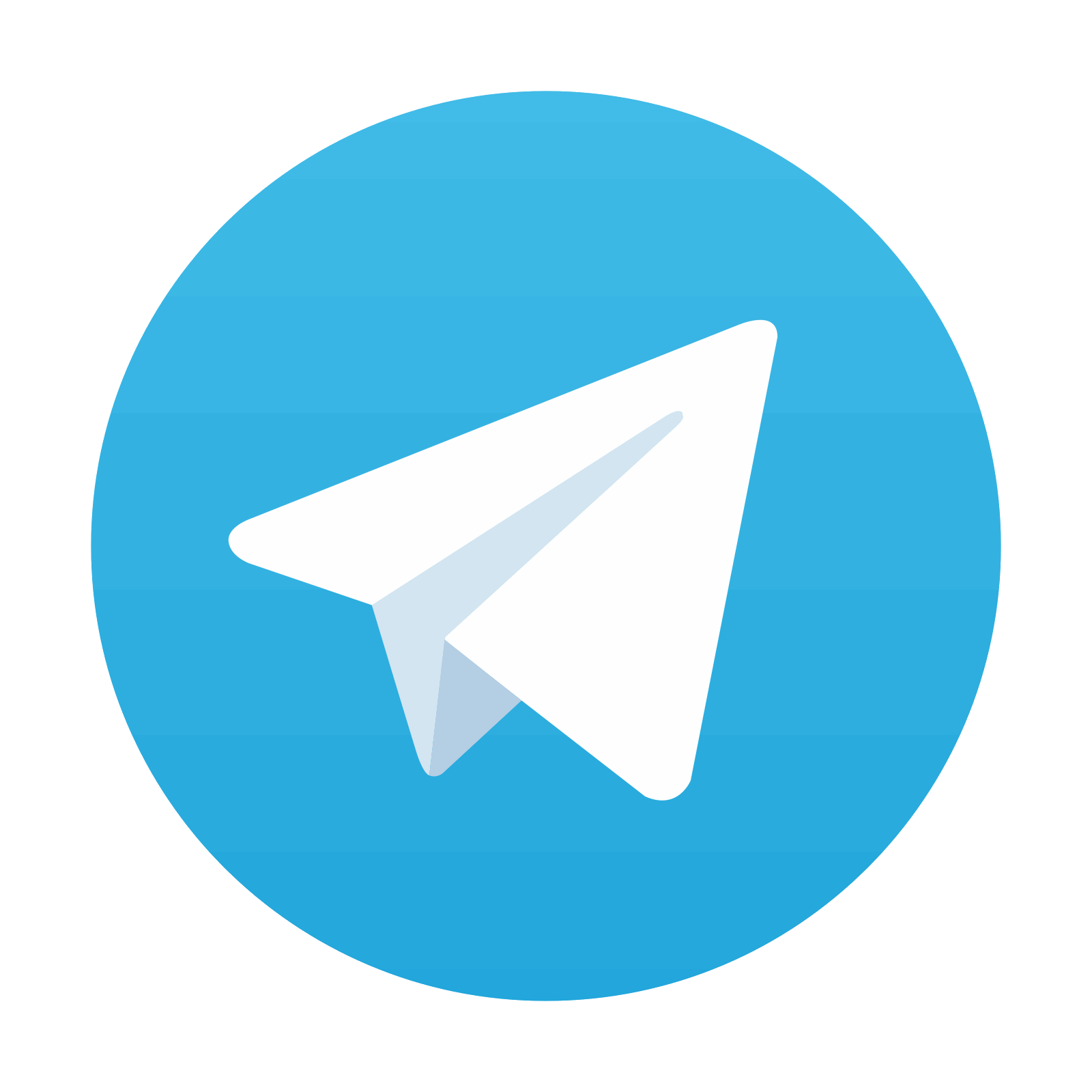
Stay updated, free articles. Join our Telegram channel

Full access? Get Clinical Tree
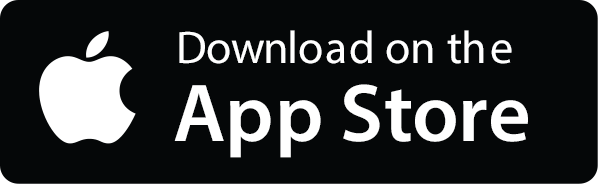
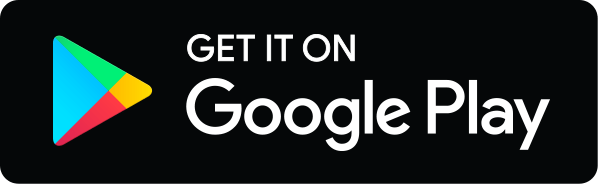