1. Equipment-related adverse events are rare. Even if equipment is faulty, user error or unfamiliarity often plays a part.
2. Critically ill children with very noncompliant lungs require minimal circuit and ventilator compression volumes. For these patients, critical care ventilators are often a better choice.
3. High-quality digital images can now be transmitted to a viewing screen from the tip of the laryngoscope and an improved view often results, favorably shifting the Cormack score.
4. While the EEG is a random, continuous signal from the ongoing activity of the outer layers of the cortex, an evoked potential (EP) is the brain’s response to a repetitive stimulus along a specific nerve pathway. EPs help to monitor the functional integrity of neural structures that may be at risk during a particular surgical approach or involving a particular anesthetic technique.
5. By comparison to pulse oximetry, cerebral oximeters trend venous-weighted measurements because the entire returned signal is measured rather than just the pulsatile measurements that make pulse oximetry specific to arterial blood oxygen saturation.
6. Notwithstanding more robust and newer cardiac rhythm management devices (CRMDs) in pediatric patients (bipolar compared to unipolar leads, e.g., better shielding), the risk of electromagnetic interference still exists, and therefore, clinicians still need to know as much about the CRMD as possible.
7. Ultrasound imaging has been shown to improve the success of internal jugular vein catheterization.
8. High-frequency transducers produce beams that are more unidirectional and focused with shorter wavelengths, so they yield high-resolution images but attenuate more quickly, and thus penetrate less deeply. Superficial structures, especially in small people, are ideally suited for this technology. Lower-frequency transducer beams are more multidirectional and have longer wavelengths; therefore, they attenuate less quickly and penetrate deep tissues better; however, there is less resolution.
9. Pediatric anesthesia lends itself perfectly to the use of specialized apps because of the two-order-of-magnitude variation among patients.
INTRODUCTION
Equipment-related adverse events are rare. Even if equipment is faulty, user error or unfamiliarity often plays a part (1). Although the majority of these cases reflect errors in judgment as well as equipment failure or misapplication, in a procedure- and equipment-dependent specialty like anesthesiology, a careful consideration of special equipment needs and clinical circumstances is mandatory. Moreover, the American Society of Anesthesiologists Closed Claims Study found a greater incidence of equipment-related problems in children compared to adult patients, almost half occurring in children less than 2 years of age (2).
When adult equipment is simply made smaller, “miniaturization” of anesthetic equipment may impose a loss of functionality, like the loss of a suction channel on a bronchoscope. In addition, children’s developing organ systems will often have qualitative and quantitative differences. Ironically, however, advances in technology often have specific appeal because of the special challenges in taking care of children. The largest areas of interest to emerge in the last several years are noninvasive monitoring devices, point-of-care instrumentation, and imaging technology applied to practical, clinical use (3). I will therefore avoid in-depth discussions of pediatric anesthesia equipment covered in more general reviews and focus more on “developing” areas of equipment and devices for the care of children.
GAS DELIVERY AND EXCHANGE
BREATHING CIRCUITS
1. The circle anesthesia system or variations of Ayre T-piece (Mapleson D, Bain circuit, or the Mapleson F Jackson-Rees modification of Ayre T-piece) (4) are the most common breathing circuits for pediatric patients. Advances in circuit design, materials utilized, and the training of pediatric anesthesiologists have rendered most of the strident arguments about partial rebreathing versus nonrebreathing circuits of more historical than practical importance.
2. A conventional circle system breathing circuit has two unidirectional valves which make it possible for anesthetic gases to flow in one direction, thus preventing rebreathing of carbon dioxide while allowing rebreathing of the bulk of the anesthetic gas mixture through the rebreathing (reservoir) bag.
3. Dr. Phillip Ayre created the T-piece that bears his name eponymously (5). When the fresh gas flow is high enough that rebreathing does not occur, the PaCO2 is determined by the minute ventilation. When the minute ventilation is significantly greater than the fresh gas flow, it is the fresh gas flow that becomes the principal determinant of the PaCO2 and the basis for controlled partial rebreathing.
4. Regardless of the circuit chosen, breathing circuits with large compression volumes will be “ventilated” far in excess of the volume delivered to the patient. The adequacy of pulmonary ventilation is monitored by physical examination (adequacy of chest wall excursion, auscultation), end-tidal respiratory gas analysis, manometry, spirometry, and, when necessary, blood gas analysis. For small children, spirometer placement between the patient and the Y-piece is optimal. In this location, spontaneous and controlled ventilation volumes will be measured more accurately; however, additional dead space as well as kinking of the endotracheal tube (ETT) is possible. Pediatric circle breathing systems, smaller versions of adult circle systems, have the specific advantage of lower circuit volume and a smaller radius of curvature of the tubing, which, according to Laplace’s law, makes them less distensible and, therefore, decreases the compression volume. Distinct from compression volume, dead space only exists when fresh and exhaled gases are mixed. The proper size rebreathing bag for children has a volume approximately equal to the patient’s vital capacity, or three times the tidal volume. The same calculation is applicable for the doubly open (Boothby-Lovelace-Bourbillion) rebreathing bags used on the Jackson-Rees modification of Ayre T-piece.
CLINICAL PEARL Cold, anhydrous gases delivered to a breathing circuit adversely affect mucociliary transport and contribute to tracheal tube obstruction because of dried or thickened secretions (6–10). This is of particular importance for the smaller tubes utilized in children.
Cold, anhydrous gases delivered to a breathing circuit adversely affect mucociliary transport and contribute to tracheal tube obstruction because of dried or thickened secretions (6–10). This is of particular importance for the smaller tubes utilized in children. Moreover, the latent heat of vaporization supplied by the patient to these cold, dry gases results in additional heat loss. Heated humidifiers and passive heat and moisture exchangers are effective strategies for guarding against heat and moisture loss, as evaporative cooling via the airways is minimized. Controlled partial rebreathing in Mapleson D or Bain systems can achieve an airway humidity of 24 to 26 mg H2O per L within 30 minutes without the use of external humidifiers or heat and moisture exchangers (11). A final alternative is low-flow or closed-circuit anesthesia using the circle system (12).
Anesthesia Ventilators
1. The mechanical ventilator portion of the circuit replaces the rebreathing bag. With the adult bellows in place, the tidal volume may usually be adjusted between 50 and 1,500 mL; small volumes may be delivered with an adult bellows by altering the fresh gas flow rate and I:E ratio. However, the use of a pediatric bellows (typically 300 mL maximum tidal volume) will reduce the bellows’ contribution to the compression volume of the breathing circuit. Critically ill children with very noncompliant lungs require minimal circuit and ventilator compression volumes. For these patients, critical care ventilators are often a better choice.
2. Piston-driven ventilators are replacing bellows-driven anesthesia ventilators because of their greater precision with regard to the volume of gas delivered (improved volume-controlled ventilation) and the improvement in accuracy of pressure controlled ventilation because of the greater rigidity of coupling between the piston and its drive mechanism.
3. Modern anesthesia ventilators eliminate many of the above drawbacks. For example, to ensure that the set tidal volume or pressure in the breathing circuit will remain constant even if fresh gas flow is altered, fresh gas flow decoupling is utilized. Furthermore, to ensure that the set tidal volume is delivered to the patient even if inspiratory pressures change, compliance compensation is incorporated into the system (13).
SUPRAGLOTTIC AIRWAYS
Laryngeal Mask Airways
1. Pediatric laryngeal mask airways (LMAs) are available in a range of sizes. (Table 6.1) They are made of medical-grade silicone rubber and are latex free. The no. 1 LMA, which is a miniaturized version of the adult LMA, has worked satisfactorily even in premature infants of 1 kg, in newborn resuscitation, and in airway maintenance for infants with upper airway congenital anomalies (e.g., Pierre–Robin, Goldenhar, Treacher Collins, and Schwartz–Jampel syndromes) (14–16). Frequently, the trachea can be intubated through the LMA without fiberoptic laryngoscopy, spontaneous breathing helping to direct the tracheal tube.
2. Second-generation LMAs were designed to improve safety by providing gastric access to reduce aspiration. The LMA Supreme is similar to the adult Proseal in intent, making it safer to deliver positive pressure ventilation because of the extraoral gastric vent, and has high reliability as far as success of insertion and gastric tube placement. It comes in pediatric sizes one and two.
3. LMAs designed to facilitate tracheal intubation are available, the most recent of which are the Air-Q (Cookgas, St. Louis, MO) and the Ambu Aura-I (Ambu USA, Glen Burnie, MD). The Ambu Aura-I does not have a detachable proximal connection; therefore, it has a narrower orifice for the tracheal tube and pilot balloon to pass through, which may prove problematic in smaller children, especially when cuffed ETTs are desired (17).
OTHER INTRAORAL DEVICES
Oral Airway
An oral airway that is too small will displace the base of the tongue inferiorly toward the pharynx, whereas an airway that is too large may reach to the laryngeal inlet and cause trauma and/or laryngeal stimulation and laryngospasm. Correct airway size can be assessed by a careful external examination of the child and estimation of the distance from the teeth to the base of the tongue. Oral airways are also often used as “bite blocks” to prevent the clenching of teeth on the ETT, a practice that may be hazardous in children between 5 and 10 years of age who are likely to have loose deciduous teeth. Oral airways are responsible for up to 55% of dental complications (18). Furthermore, an oral airway used as a bite block in long cases may cause tongue necrosis or edema, uvular edema, or lip damage. A dental roll placed between the upper and lower molars will also prevent clenching while minimizing dental trauma.
CLINICAL PEARL Oral airways are also often used as “bite blocks” to prevent the clenching of teeth on the ETT, a practice that may be hazardous in children between 5 and 10 years of age who are likely to have loose deciduous teeth.
NASAL AIRWAY
Nasopharyngeal airways are generally constructed from rubber or polyvinyl chloride and are miniaturized for pediatric use. Care must be exercised when using nasopharyngeal airways in children with a bleeding diathesis or congenital abnormalities of the midface such as choanal stenosis or frontonasal dysplasia. The appropriate length for a nasopharyngeal airway may be estimated from the distance between the auditory meatus to the tip of the nose, and the size of the airway may be estimated from the calculated ETT size converted to French size (approximately the internal diameter in millimeter multiplied by four, or the external diameter in millimeter multiplied by three). If the nasopharyngeal airway is too long, it may induce coughing or laryngospasm; if too short, the upper airway obstruction may not be relieved.
ENDOTRACHEAL TUBES
1. Formulas for appropriate ETT size and length are based either on age or direct examination of the patient (Table 6.2). An emerging role for ultrasound imaging of the airway to guide tube size selection is taking place, but it is unclear that it will replace the previously established methods (19), although there may be compelling reasons to study this technology further for patients with airway anomalies or significant comorbidities related to airway development. A deflated cuff adds about 0.5 mm to the tube’s external diameter when calculating ETT sizes for children. In addition, since nitrous oxide enters the closed air space of a cuff when the valve on the pilot tube is competent, the intracuff pressure should be monitored and maintained at less than 25 mm Hg.
2. A reasonable leak (between 10 and 30 cm H2O) may be maintained following endotracheal intubation to minimize the risk of postintubation croup (20), atmospheric pollution, or failure of the ventilated volume to return through the exhalation limb of the breathing circuit. Typically, uncuffed tracheal tubes have been used in infants and small children for fear of tracheal damage from high intracuff pressures. Recent experience, however, with the new low-pressure, high-volume cuffs has shown no indication that they cause harm to children. They can be used routinely as long as prolonged high pressures are avoided (21,22). Circumstances specific to the procedure (abdominal or thoracic surgery) or to the patient (poor pulmonary compliance) may require higher peak inspiratory pressures to assure adequate minute ventilation; therefore, tube selection should be individualized. Likewise, children easily susceptible to croup during viral illnesses of the respiratory tract may benefit from tracheal intubation with a smaller tube, as long as the lungs can be ventilated adequately. Intriguingly, it is not at all clear that lower cuff pressures reduce mucosal damage (23).
3. Endotracheal tubes without a hole opposite the bevel are known as Magill tubes; those with the hole (the Murphy eye) are called Murphy tubes. The Murphy eye was designed to provide an alternate pathway for gas flow if the end-hole of the ETT was occluded, an important consideration for infants, especially those undergoing thoracic surgery.
ENDOBRONCHIAL TUBES
Endobronchial tubes are usually employed in adults in order to provide single-lung ventilation for lung isolation and to facilitate video-assisted thoracoscopic (VATS) procedures. While endobronchial tubes are manufactured from 28 to 41 Fr, alternate means have to be sought to provide single-lung ventilation in infants and small children. Conventional or Arndt endobronchial blockers or single-lumen tubes fiberoptically directed to one or the other mainstem bronchus are currently used to isolate the lungs (24,25).
AIRWAY VISUALIZATION EQUIPMENT
LARYNGOSCOPES
Developmental differences in the size, position, and dimensions of airway anatomy are influential in selecting airway equipment for normal children, and routine pediatric airway equipment of smaller size is sufficient. Standard pediatric laryngoscopes and justifications for choices for specific patient ages are readily available in standard textbooks and have been reviewed elsewhere (26). Most special laryngoscopes and airway techniques require practice for successful use.
RIGID FIBEROPTIC LARYNGOSCOPES
Older rigid fiberoptic scopes include the Bullard, Upsher, and Wu intubating laryngoscopes. As an example, the Bullard laryngoscope is a rigid curved blade available in neonatal, pediatric, and adult sizes. A working channel and two fiberoptic bundles for lighting and viewing are integrated into the blade. The working channel is useful for oxygen insufflation, which also reduces fogging. The laryngoscope is held parallel to the body axis, and the tip of the blade is guided into the mouth. After complete insertion, mild vertical pull is exerted on the laryngoscope, at which time the glottis can usually be seen through the fiberoptic bundle. The ETT is advanced into the glottic opening and trachea under visual control. In a simulated difficult infant airway comparison with residents as airway managers, higher success rates and shorter intubation times were achieved with optical-assisted laryngoscopes compared with a conventional Macintosh laryngoscope (27).
RIGID VIDEOLARYNGOSCOPES
High-quality digital images can now be transmitted to a viewing screen from the tip of the laryngoscope and an improved view often results (28), favorably shifting the Cormack score (29,30). These indirect videolaryngoscopes, all available in infant and toddler sizes, such as the Airtraq (Prodol Meditec, Vizcaya, Spain), GlideScope (Verathon, Bothell, WA), Storz DCI (Karl Storz, Tuttlingen, Germany), and the Truview PCD Infant (Truphatek, Netanya, Israel) have revolutionized airway management for children. The attributes of these laryngoscopes, feeling much the same in the hand as a conventional laryngoscope but with much better imaging of the difficult airway, have been very well reviewed by Holm-Knudsen and are summarized in Table 6.3 (31).
FLEXIBLE FIBERSCOPE
Numerous fiberscopes are now available in miniaturized versions for use in children. The smallest do not have suction channels. As an instrument, it is relatively simple but delicate, and the smaller it is, the more fragile. Larger scopes may have channels for biopsy, suction, and insufflation of oxygen or anesthetic mixtures.
THE LIGHTED STYLET AND OTHER TECHNIQUES
The “lightwand” is a malleable stylet with a small light at its tip. It is best used in the spontaneously breathing sedated or anesthetized patient. Patients whose tracheas were found extremely difficult to intubate by conventional means were intubated without difficulty using the lighted stylet (32,33). The pediatric optical telescope, typically inserted through a bronchoscope for examination of the airway, may also be used for difficult intubations by threading an ETT over the telescope and advancing the tube into the trachea (34).
MONITORING
RESPIRATORY GAS MONITORING EQUIPMENT
The adequacy of pulmonary ventilation with nonrebreathing or controlled partial rebreathing can be assured noninvasively with inspired and end-tidal carbon dioxide analysis. In addition, the measurement of inspired and end-tidal volatile anesthetic concentrations permits verification of the accuracy of a vaporizer’s output at varying flows, verification of the identity of the inhaled anesthetic, and the correlation of the clinical assessment of the anesthetic depth with the end-tidal anesthetic concentration.
Carbon Dioxide
CLINICAL PEARL The accuracy of ETCO2 measurement in children depends on the respiratory frequency (ƒ), variations in the aspiration flow rate, the sampling site (whether distal or proximal airway), and the type of capnometer.
1. Artifactual widening of the alveolar-arterial CO2 (A-aCO2) gradient is a particular problem with children because of the use of uncuffed ETTs as well as aspiration flow rates that may exceed the maximum expiratory flow rate of the patient.
a. The accuracy of ETCO2 measurement in children depends on the respiratory frequency (ƒ), variations in the aspiration flow rate, the sampling site (whether distal or proximal airway), and the type of capnometer.
b. As ƒ increases, measured values of inspired CO2 increase in the Bain and circle breathing systems. The ETCO2 decreases as ƒ increases in circle anesthesia systems, but it remains constant in Bain systems.
c. Parabolic distortion of CO2 “plugs” makes up a large percentage of this elevated baseline in aspiration capnography, depending on f and breathing circuit type (35). In patients less than 6 kg, differences have been found in the accuracy of ETCO2 measurement at the distal end of the ETT compared with the proximal end, the proximal sidestream connector, and the Y-piece of the ventilator.
d. Alterations in tidal volume made less of a difference in comparing end-tidal CO2 with capillary CO2 than alterations in respiratory rate.
2. High respiratory rates may contribute to inadequate ventilation of lung regions with long time constants (36,37). When an Ayre T-piece is used to ventilate the lungs of children weighing less than 10 kg, distal gas sampling for ETCO2 measurement is more accurate (38).
a. Capnography may be used with LMAs; however, patients may have a higher ETCO2 than PaCO2 (negative P(a-ET)CO2 gradients) values when measured at distal airway sites (39).
3. The flow rate for gas sampling should not be reduced out of concern for the loss of circuit volume. A faster sampling rate enhances the accuracy of measurement because the response time is decreased, and at higher respiratory rates, an artifactually lower ETCO2 and higher inspired CO2 are avoided (40,41). Strategies that enhance the accuracy of capnography in children include a fast sampling rate and distal airway sampling.
4. Sampling errors can occur as a result of the dilution of exhaled gases with fresh gas devoid of CO2, which may occur while using high flows in nonrebreathing systems.
a. The degree of dilution depends on the relationship between fresh gas flow rate, the aspiration sampling rate, and the expiratory flow rate of the patient.
b. Sample dilution may also be seen when ETTs in small children have a very low (i.e., less than 10 cm H2O) “leak,” as exhaled gas is preferentially vented around the ETT rather than through its lumen.
c. Sampling within the endotracheal tube or at the ETT connector generally helps with this problem. Although sampling within the tracheal tube has the advantage of better accuracy, the sampling port can easily become obstructed by secretions or humidity.
5. ETCO2 measurements for pediatric patients with cyanotic congenital heart disease tend to be lower in the presence of reduced pulmonary blood flow or right-to-left shunts, and the A-aCO2 gradient will be wider in these cases. In children with cyanotic-shunting congenital heart lesions, the ETCO2-PaCO2 gradient is not stable, and ETCO2 cannot be used to reliably estimate the PaCO2 (42).
6. This may also be true for pediatric patients with pulmonary disease, in whom the large differences between PaCO2 and ETCO2 were comparable to those observed in children with cyanotic congenital heart disease (43).
7. The interpretation of the capnograph waveform and value measured in children has been called into question, but generally is found to be reliable (44). Nevertheless, there are several specific situations with regard to children that merit special mention.
a. The tracheas of most children less than 10 years of age are still intubated with a cuffless tube which generally has a leak and, therefore, may produce an incomplete, nonsquare wave capnograph trace.
b. In addition, the capnograph trace will typically show a double hump (rebreathing of CO2 and ETCO2) with an elevated baseline when patients are ventilated with nonrebreathing systems at partial rebreathing flows.
c. Respiratory rate, frequently higher in pediatric patients, may result in an increased FICO2.
d. The maximum expiratory flow rate of the patient may be far lower than the sampling flow rate of the capnography system; therefore, the patient’s ETCO2 will have a tendency to be washed out and artifactually lowered. This can be compensated for by delivering a large tidal breaths, a throat pack to seal the escaping patient gases, intratracheal sampling with a thin catheter, airway sampling at the 15-mm connector, or a decrease in respiratory rate.
Anesthetic Agents
Volatile anesthetic concentrations may be measured by infrared absorption spectroscopy, mass spectrometry, spectral shift scattering (Raman spectrometer), or acoustic spectroscopy. The accuracy depends on the instrumentation as well as respiratory frequency. When extremely rapid respiratory rates are employed, it has been suggested that the ventilatory rate should be temporarily reduced and the end-tidal volatile anesthetic level measured (45). Because propofol has some volatility, it can also be detected in exhaled breath although the clinical correlates as well as the relationship between plasma and end-tidal levels remain unclear (46).
Additional Atmospheric Gases—Nitrogen
Routine nitrogen monitoring is not typical in most operating rooms (ORs) because tissues are progressively denitrogenated with the use of nitrous oxide and oxygen; if a nitrogen and oxygen mixture is selected in order to avoid the use of nitrous oxide, there is little mystery as to the percentage concentration of nitrogen in the anesthetic circuit. Nevertheless, when air embolism is a possibility, the measurement of end-tidal nitrogen will not only aid in the diagnosis but also in monitoring the resolution of acute symptoms and the potential for ongoing air entrainment (47).
CENTRAL NERVOUS SYSTEM
While it is clear that there are anatomic and neurotransmitter differences that develop during the first several years of life, it is not at all clear what implications this may have for intraoperative monitoring. This evolving science and technology is at the forefront of monitoring challenges in pediatric anesthesia. Monitors of cerebral well-being include intracranial pressure (ICP), 21-lead and processed electroencephalography (EEG), EP monitoring, cerebral oxygen monitoring via jugular bulb venous oxygen saturation (SjVO2), near-infrared spectroscopy (NIRS), and transcranial Doppler sonography (TCD) (48).
ICP Monitoring
Direct access is a reliable method of assessing ICP as well as an excellent route for drainage of cerebrospinal fluid to relieve pressure elevations. Furthermore, cerebral perfusion pressure (CPP = mean arterial pressure-ICP) can be calculated in an effort to try to optimize cerebral perfusion. A CPP of at least 40 mm Hg (range 40 to 65 mm Hg) is currently recommended as a guide to treatment following severe traumatic brain injury in children; however, this is based on scant evidence albeit a common sense guideline (49).
CLINICAL PEARL A CPP of at least 40 mm Hg (range 40 to 65 mm Hg) is currently recommended as a guide to treatment following severe traumatic brain injury in children; however, this is based on scant evidence albeit a common sense guideline.
Electroencephalography
EEG has been correlated with alertness in adults. Because a child’s developing brain is not identical to that of an adult, the use of cerebral function monitors in small children remains controversial. The BISpectral Array is probably the most well recognized of these monitors currently. Correlation with standard clinical assessments of sedation and anesthetic depth is less consistent at younger ages, especially less than 1 year (50–52). There may also be some specific differences in Bispectral index (BIS) values according to the anesthetic agent used; for example, BIS values with halothane are consistently higher than equi-MAC values of sevoflurane in children (53).
EP Monitoring
1. While the EEG is a random, continuous signal from the ongoing activity of the outer layers of the cortex, an EP is the brain’s response to a repetitive stimulus along a specific nerve pathway. EPs help to monitor the functional integrity of neural structures that may be at risk during a particular surgical approach or involving a particular anesthetic technique. The EP usually is recorded from the specific cortical area corresponding to the stimulus input.
a. If a small electrical current applied to the overlying skin stimulates a nerve on the arm or the leg, it is called the somatosensory EP (SSEP).
b. If, however, the retina is stimulated by means of flicker light or a sudden change in a checkerboard pattern, the EP recorded over the corresponding cortical area is called the visual EP (VEP).
c. If the auditory nerve is stimulated by “clicks” from headphones, it is called the auditory EP (AEP).
d. The early part of the AEP waveform (less than 10 ms) is called the brainstem AEP (BAEP) since it reflects the passing of the impulse through the brainstem. BAEP monitoring is utilized most frequently in children for correct implantation and verification of electrode placement of cochlear implants. It may also be utilized for brainstem surgery, such as monitoring of the auditory nerve during vestibular Schwannoma resection.
1. As a monitoring modality, EPs are routinely used during surgical procedures that might compromise part of the brain or the spinal cord.
2. Various modalities of EP monitoring are useful for assessing the integrity of neural tracts, particularly during spine distraction and scoliosis surgery.
a. SSEPs are elicited by electrically stimulating a mixed peripheral nerve (usually the posterior tibial, peroneal, or sural nerves) and recording the response from electrodes at distant sites cephalad to the site of surgery. Responses are recorded repeatedly.
b. The functional integrity of the somatosensory pathways is determined by comparing the amplitude and latency change intraoperatively with baseline values.
c. A reduction in the amplitude of the response by 50% and an increase in the latency by 10% are typically considered significant. It is assumed that when using SSEPs, any damage to the motor tracts will be signaled by a change in SSEPs. This, however, cannot be guaranteed, and for this reason, motor EP (MEP) monitoring has been developed.
d. MEP monitoring techniques are subdivided according to the site of stimulation (motor cortex, spinal cord), the method of stimulation (electrical potential, magnetic field), and the site of recording (spinal cord, peripheral mixed nerve, muscle). MEP monitoring is less reliable in patients with a preexisting neurological deficit. Furthermore, early hopes of greater sensitivity of MEPs over other monitoring techniques may have been premature.
e. SSEP monitoring is less affected by the technical difficulties associated with MEP monitoring. MEP monitoring is, however, becoming more widely used, and the two methods are complementary.
CLINICAL PEARL The functional integrity of the somatosensory pathways is determined by comparing the amplitude and latency change intraoperatively with baseline values. A reduction in the amplitude of the response by 50% and an increase in the latency by 10% are typically considered significant.
OXYGEN MONITORING
Near-infrared light with a wavelength between 650 and 950 nm penetrates biologic tissue without harmful ionization and is absorbed by a limited number of chromophores found in human tissue—notably hemoglobin (Hb), oxyhemoglobin (Hb-O2), and cytochrome aa3. Further, these chromophore species absorb light differentially across the near-infrared bandwidth; so the use of multiple wavelengths of light permits quantitation of the relative concentrations of Hb and Hb-O2 separately (54).
Pulse Oximetry
CLINICAL PEARL Delay of an anesthetic induction while waiting for a “baseline” may make the child more anxious, as attention is often focused more on the monitor than on the patient. These monitors should often be placed during or following anesthetic induction in extremely anxious and upset preschool children, once they have become motionless.
1. Pulse oximetry has become a standard of practice and is arguably the most important safety monitor (55,56). Pulse oximeters rarely function well in the active, nonanesthetized small child because motion artifacts prevent the accurate determination of pulsatile blood flow.
2. Pulse oximeters in clinical use in the OR measure functional hemoglobin saturation (the percentage of oxyhemoglobin in relation to the sum of oxyhemoglobin and deoxyhemoglobin), but they do not measure dysfunctional hemoglobins such as carboxyhemoglobin or methemoglobin.
a. Fetal hemoglobin, skin color, and bilirubin or tissue thickness do not usually affect pulse oximeters.
b. Intravenously administered dyes such as methylene blue and indocyanine green can cause errors in pulse oximeter readings (57–59).
CLINICAL PEARL In infants, if the measured oxyhemoglobin saturation is intended to approximate cerebral oxygenation, then preductal placement (i.e., right hand or ears) is desirable. Postductal placement of the oximeter probe in a neonate will reflect oxygen saturation delivered to tissues distal to the ductus arteriosus, and may be extremely helpful in patients with persistent pulmonary hypertension of the newborn.
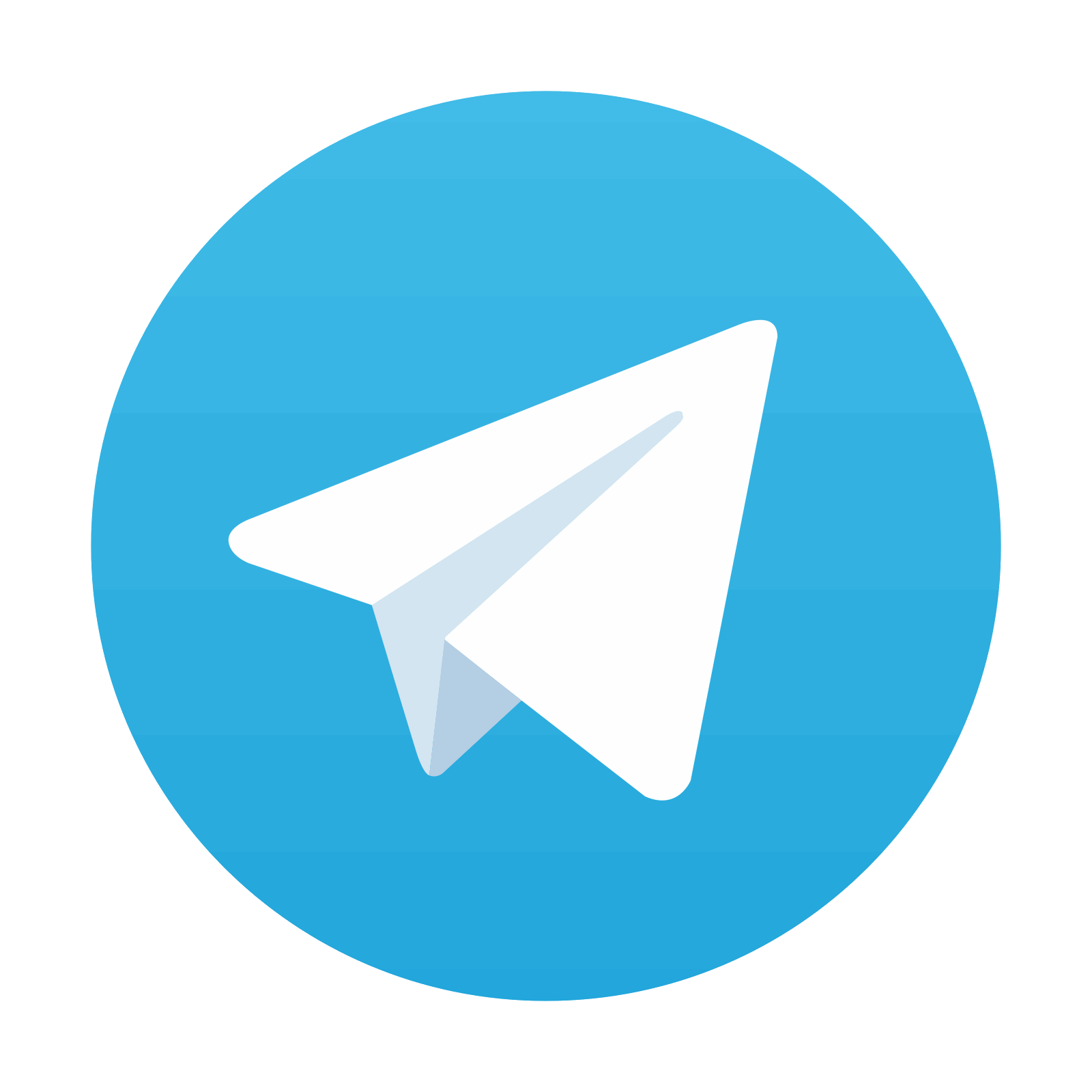
Stay updated, free articles. Join our Telegram channel

Full access? Get Clinical Tree
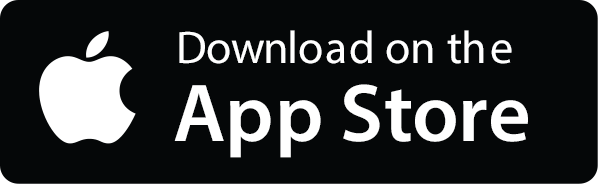
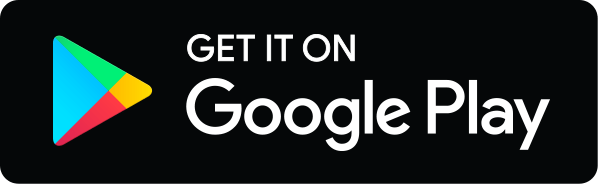