Cardiothoracic surgery entails unique, often extreme, conditions in comparison to noncardiac surgery. Profound hemodynamic and physiologic perturbations associated with hypothermic cardiopulmonary bypass (CPB) and circulatory arrest lead to unique dosing considerations for anesthetic drugs, anticoagulants, vasoactives, inotropes, and antifibrinolytics. This chapter will review the pharmacologic considerations of cardiac anesthesia chronologically in stages, from premedication and induction to pre-CPB maintenance, initiation of CPB, separation from CPB, and the post-CPB period. A special discussion will review the unique pharmacologic approach to anticipated deep hypothermic circulatory arrest (DHCA).
Benzodiazepine (eg, intravenous [IV] midazolam 0.02–0.04 mg/kg) may be given prior to arterial line and/or central line placement. Cardiac surgery has among the highest incidence of intraoperative awareness of any elective surgery (1%–2%). Benzodiazepine administration has been shown to decrease the risk of awareness.1 Benzodiazepines also have a relatively slow offset time with respect to their hypnotic effects, particularly in elderly patients. Lorazepam (0.02 mg/kg IV) has a half-life of 12 to 14 hours in healthy young patients, with evidence of residual sedation and electroencephalographic (EEG) changes 8 hours after a single dose.2 In comparison, the half-life of midazolam is 2.8 hours, with EEG returning to near baseline by 3 hours.3 In the setting of cardiac surgery with CPB, delayed awakening attributed to the sedative effects of lorazepam may be more than 9 hours.4 Residual postoperative sedation is of potential concern in patients where early extubation is desired (eg, “fast-track” anesthesia). Midazolam in anxiolytic doses administered preoperatively and pre-CPB is not typically associated with delayed awakening. However, caution should be exercised when considering even a single preoperative dose of lorazepam, or a post-CPB dose of any benzodiazepine, if early extubation is desired.
Beginning slow titration of IV fentanyl 0.5 to 1.0 mcg/kg or sufentanil 0.05 to 0.1 mcg/kg during placement of invasive lines may be considered.
Similar blunting of the autonomic response to intubation and sternotomy has been demonstrated for fentanyl, sufentanil, alfentanil, and morphine at equianalgesic doses. Ideally, laryngoscopy should be performed adequate analgesia is in place to avoid or blunt an adverse hemodynamic effect. Although all these opioids are similar in pharmacologic action (ie, μ-receptor agonists), they each have quite different pharmacokinetics. One major difference is the time to peak effect. This difference is important to consider when dosing an opioid just prior to laryngoscopy. As is described in Chapter 5, Figure 5–1, the time to peak effect varies for each opioid: alfentanil, less than 2 minutes; fentanyl, 4 minutes; sufentanil, 6 minutes; and morphine, more than 80 minutes.
Induction drugs include propofol, etomidate, ketamine, high-dose midazolam, and the combination of ketamine and midazolam. Among the choices of induction agents, propofol leads to the greatest decrease in systemic vascular resistance (SVR) and may be associated with hypotension, even in modest doses. In comparison to ketamine (2 mg/kg), a propofol-based induction (0.5 mg/kg) produced a transient decrease in SVR, pulmonary vascular resistance (PVR), and mean arterial pressure (MAP). Although the cardiac index is decreased slightly (< 10%) with both induction drugs, no significant difference in cardiac index or heart rate was shown between the 2 strategies.5 The decrease in SVR with propofol in this study was transient, with return to baseline after endotracheal intubation.
Etomidate, in comparison to propofol, is associated with significantly less decrease in SVR and MAP, without any difference in cardiac index in patients with coronary artery disease (CAD) or aortic valve disease.6 This was also shown in patients with severe aortic stenosis, where propofol induction was associated with a greater incidence of hypotension than etomidate. However, etomidate is associated with transient adrenal suppression.7 Even a routine induction dose can suppress adrenal function for more than 24 hours.8 Etomidate may be dangerous in septic and critically ill patients who require steroids to maintain their immune function and metabolic homeostasis. Some authors have recommended abandoning etomidate in septic patients or providing supplemental corticosteroid therapy following etomidate administration.9
Midazolam as a sole sedative–hypnotic induction agent has been described, typically in higher doses (0.1–0.2 mg/kg) than used for anxiolysis. The time to peak effect of an induction dose of midazolam is significantly longer (6–8 minutes) than propofol, etomidate, or ketamine. Thus, it should be given earlier in the induction sequence. In patients with normal and decreased left ventricular function, midazolam has been shown to have minimal effect on heart rate, SVR, and cardiac index.10
The nondepolarizing muscle relaxants (NDMRs) such as rocuronium (0.6 mg/kg), vecuronium (0.05 mg/kg), or cisatracurium (0.1 mg/kg) will provide optimal conditions for direct laryngoscopy and endotracheal intubation without significant hemodynamic changes. Succinylcholine 1 mg/kg is used in the setting of a rapid-sequence induction or suspected difficult airway. Succinylcholine, although more rapid in onset than the NDMRs, has been associated with tachycardia and rarely dysrhythmias in certain patients.11 One study in patients with CAD comparing succinylcholine and vecuronium demonstrated a transient increase in heart rate with succinylcholine (11% increase from baseline) and MAP (25% increase from baseline). These hemodynamic effects are short-lived and return to near baseline within 4 minutes of administration.12 The benefits of succinylcholine should be balanced with its adverse effects in patients with ischemic coronary disease and valvular disease.
The time required before peak plasma concentration of any IV medication is dependent upon both the diffusivity of the drug and the cardiac output. In patients with decreased cardiac output, the time of peak plasma concentration (and hence effect-site concentration) may be delayed by up to 60 seconds compared to individuals with normal cardiac output.13 This applies to premedications, opioid and sedative induction agents, and muscle relaxants. Consideration should be given to this potential delay in peak effect to avoid “stacking” doses of medications prior to the peak of the initial dose.
Of the 3 commonly used volatile anesthetics, isoflurane is the most studied in cardiac anesthesia and has been shown to play a role in ischemic preconditioning, helping to reduce infarct size and possibly improve morbidity and mortality in cardiac surgery. More recently, both desflurane and sevoflurane have been associated with decreased troponin release and shorter intensive care unit stay when compared to total IV anesthesia (TIVA).14 The mechanisms of myocardial protection are multifactorial but appear to be unique to volatile anesthetics. The issue remains controversial, and a large retrospective analysis recently showed no difference in markers of ischemia or outcomes between sevoflurane-based and TIVA anesthetics. All volatile anesthetics decrease myocardial function to a modest degree. Isoflurane may preserve myocardial function better than sevoflurane at concentrations equivalent to 1 minimum alveolar concentration (MAC).15
It is well established that concomitant administration of opioids has a “MAC-reducing” effect, decreasing the concentration of volatile anesthetic required to maintain unresponsiveness and to blunt the adrenergic response to surgical stimulation.16 When administering isoflurane to patients with substantial cardiac disease, dosing is reduced to avoid the cardiodepressant effects of isoflurane and opioids are used to make up unmet anesthetic requirements. One advantage of opioids is their minimal impact on cardiovascular function, even at high doses. This feature, although well characterized when administered alone, is not as distinct in the presence of other anesthetics, such as potent inhaled agents. Patients may have pronounced hemodynamic responses to opioids in the presence of other anesthetics.
The interaction between opioids and inhaled agents is often difficult to conceptualize in a clinical context. In general, the interactions are synergistic. As was described in Chapter 3, the extent of synergism varies for different effects. For example, the interactions are more pronounced for analgesia than for loss of responsiveness. Consider the simulations presented in Figure 31–1, which illustrate the synergy between isoflurane and intermittent boluses of fentanyl. This simulation assumes that the isoflurane vaporizer is set to 0.9% in a patient with normal cardiac function and normal ventilator settings. It also assumes that the isoflurane has been on for 20 minutes and has not yet reached near steady state. Three 1-mcg/kg fentanyl boluses were administered intermittently over a 1-hour period, each bolus separated by 15 minutes. Figure 31–1 presents the time course of predicted effect-site concentrations for each drug and 2 predicted effects: analgesia and loss of responsiveness. As with all simulations, this simulation makes several assumptions, which are described in Table 31–1.
Figure 31–1
Simulations of isoflurane and fentanyl effect-site concentration (Ce) levels and predictions of drug effects (analgesia and loss of responsiveness) over time. Simulations used published pharmacokinetic parameters17, 18, 19, 20, 21, 22, 23, and 24 for a 30-year-old, 100-kg, 183-cm male with normal cardiopulmonary physiology. Simulations assumed a mechanically ventilated patient with settings of 10 breaths per minute, tidal volume of 600 mL, and isoflurane vaporizer set to 0.9%. Interactions between fentanyl and isoflurane (analgesia and loss of responsiveness) were based on published interactions models for sevoflurane and remifentanil.25, 26, and 27 Isoflurane and fentanyl concentrations were modeled as sevoflurane and remifentanil equivalents.28, 29, and 30 The top plot presents the predicted Ce levels for isoflurane and fentanyl over an 80-minute time window. The fentanyl boluses are 1 mcg/kg and separated by 15 minutes. The letters represent a time point just prior to fentanyl administration (A) and at the peak fentanyl Ce levels for each fentanyl bolus (B–D). The middle plots present topographic representations of the interaction models for loss of responsiveness (left) and analgesia (right). The blue and green lines represent the isoeffect (isobole) lines. The dash-dot, solid, and dashed lines represent the 5%, 50%, and 95% probability of effect. The letters A through D mark the fentanyl–isoflurane concentration pairs at the time points described above.
The bottom two plots present the probability of effects over time. The probability of unresponsiveness is a blue area and the probability of analgesia is a green area for fentanyl combined with isoflurane. The probability of analgesia for fentanyl alone is a gray area, and the probability of loss of responsiveness for isoflurane alone is a gray area.


Assumptions |
Limitations
|
A few points that are well visualized in this simulation merit discussion. First, under these conditions, predictions of isoflurane effect-site concentrations continue to climb despite no change in dosing. Isoflurane requires a long time to reach near steady state (Chapter 6). So in the context of a typical cardiac case, it is likely, if isoflurane dosing remains constant, that isoflurane effect-site concentrations will continue to slowly increase. Second, although the isoflurane vaporizer is set to 0.9%, the effect-site concentration is lower (over the 80-minute window, the isoflurane effect-site concentration starts at 0.5% and ends at 0.7%). Third, the 3 fentanyl doses, separated by 15 minutes, yield effect-site concentrations that continue stack up upon one another such that the peak fentanyl effect-site concentration of the third bolus is substantially higher than the first (ie, greater than a 2-fold increase). Fourth, the synergy between fentanyl and isoflurane is more pronounced for analgesia than it is for loss of responsiveness.
Four time points are labeled with letters to illustrate the synergistic interactions. They represent the time just prior to the first fentanyl bolus (A) and the peak fentanyl effect-site concentrations after each bolus (B–D) as presented in the top plot. The letters map out the fentanyl and isoflurane effect-site concentration pairs on a topographic rendering of fentanyl–isoflurane response surfaces for loss of responsiveness and analgesia (middle plots). The 5%, 50%, and 95% isoeffect lines (also known as isoboles) are superimposed on each plot.
Without fentanyl (point A), low dose isoflurane provides a moderate to high probability of loss of responsiveness (between the 50% and 95% isoboles) and moderate probability of analgesia (near the 50% isobole). However, with the first fentanyl bolus (point B), both probabilities of effect exceed the 95% isobole. The additional fentanyl boluses only drive the probabilities of effect closer to 100% (points C and D) and are likely unnecessary.
The bottom two plots in Figure 31–1 present predictions of drug effect over time. The probability of loss of responsiveness over time is presented for isoflurane alone (gray area) and in combination with fentanyl (blue area). Fentanyl somewhat increases the sedative–hypnotic effect of isoflurane. The probability of analgesia over time is presented for fentanyl alone (gray area) and in combination with isoflurane (green area). Isoflurane substantially increases the analgesic effect of fentanyl.
As discussed in Chapter 22, patients with low cardiac output have decreased uptake of volatile anesthetic into the blood and will therefore more rapidly achieve equilibrium between alveolar concentration and effect-site concentration. Titration of volatile anesthetics will also be affected by cardiac output in a similar way. Changes in inspired concentration of volatile anesthetic will be reflected more rapidly in the brain in patients with low cardiac output. The more soluble the anesthetic, the more pronounced the effect of decreased cardiac output on the rate of equilibration. For example, simulations of isoflurane administration reveal that a change in cardiac output from 5 to 3 L/min is associated with a 15% to 20% increase in end-tidal concentration compared to target end-tidal concentration within 1 minute of the change in cardiac output.32 The same change in cardiac output leads to an increase in the end-tidal concentration of desflurane, a less-soluble anesthetic, of less than 10%.
Like a high-cardiac output state, a significant left-to-right shunt (eg, uncorrected congenital defect such as an atrial septal defect, ventricular septal defect, patent ductus arteriosus, or large patent foramen ovale) leads to increased flow through the pulmonary circulation. This will slow the equilibration and titration of volatile anesthetics, with the most pronounced effects occurring in more soluble agents (ie, isoflurane). Predominantly right-to-left shunts do not significantly alter the uptake and distribution of volatile anesthetics.
Propofol may be used in conjunction with opioids (eg, TIVA) and/or volatile anesthetics to provide balanced anesthesia in the pre-CPB period. Although propofol-based anesthesia may be associated with decreased SVR and the potential for more hypotension compared to volatile anesthesia, the TIVA approach has been used to provide hemodynamically stable anesthesia in numerous studies. Using a target-controlled infusion (TCI), the pre-CPB target plasma concentration typically ranges from 1 to -3 mcg/mL. If TCI pumps are unavailable (as in the United States), this can be achieved with propofol infusion rates of 65 to 100 mcg/kg/min.33
An example of a propofol TCI set to 3 mcg/mL is compared to a continuous infusion set at 100 mcg/kg/min for 2 hours in Figure 31–2. In this simulation, both the TCI and the continuous infusion achieve a high probability of unresponsiveness, but the infusion may require a bolus to achieve unresponsiveness in a timely manner. If just using the infusion, it may take up to 20 minutes to reach a reasonable probability of unresponsiveness. Of note, both procedures have a similar recovery time.
Figure 31–2
Simulation of a target-controlled propofol infusion set to 3 mcg/mL and a continuous propofol infusion set to a rate of 100 mcg/kg/min for 2 hours. The top plot presents the dosing regimen as infusion rates for the target-controlled infusion (blue line) and the continuous infusion (black line). The middle plot presents the propofol effect-site concentration (Ce) levels for each dosing regimen. The bottom plot presents the probability of unresponsiveness for each dosing regimen. Simulations used published pharmacokinetic parameters for propofol.34,35

Infusions of synthetic opioids are commonly used in cardiac anesthesia to maintain a steady-state level of analgesia during this period of intense stimulation. Fentanyl and sufentanil are frequent adjuncts to maintenance with volatile anesthesia, and their role in decreasing the volatile anesthetic requirement promotes preservation of myocardial function throughout the pre-CPB period. Prior studies of fentanyl have shown near-maximal analgesic effects at a plasma concentration of 7 ng/mL, whereas plasma concentrations less than 5 ng/mL are associated with an increased requirement for volatile anesthetic to control hemodynamic responses to the surgical stimuli of sternotomy, sternal lift, and sternal spreading.16 Sample bolus and continuous infusion dosing regimens that achieve this range of fentanyl concentrations are presented in Figure 31–3. Underdosing the opioid may require higher concentrations of volatile anesthetic, which in turn can lead to an undesirable decrease in myocardial contractility, underscoring the importance of balanced anesthesia in the pre-CPB period. Where available, TCI may be used to maintain this target plasma concentration. Again, if TCI is unavailable, a sufentanil bolus of 1 mcg/kg followed by an infusion of 0.6 mcg/kg/h will maintain a predicted plasma concentration greater than 0.7 ng/mL, which is associated with adequate analgesia (Figure 31–4).
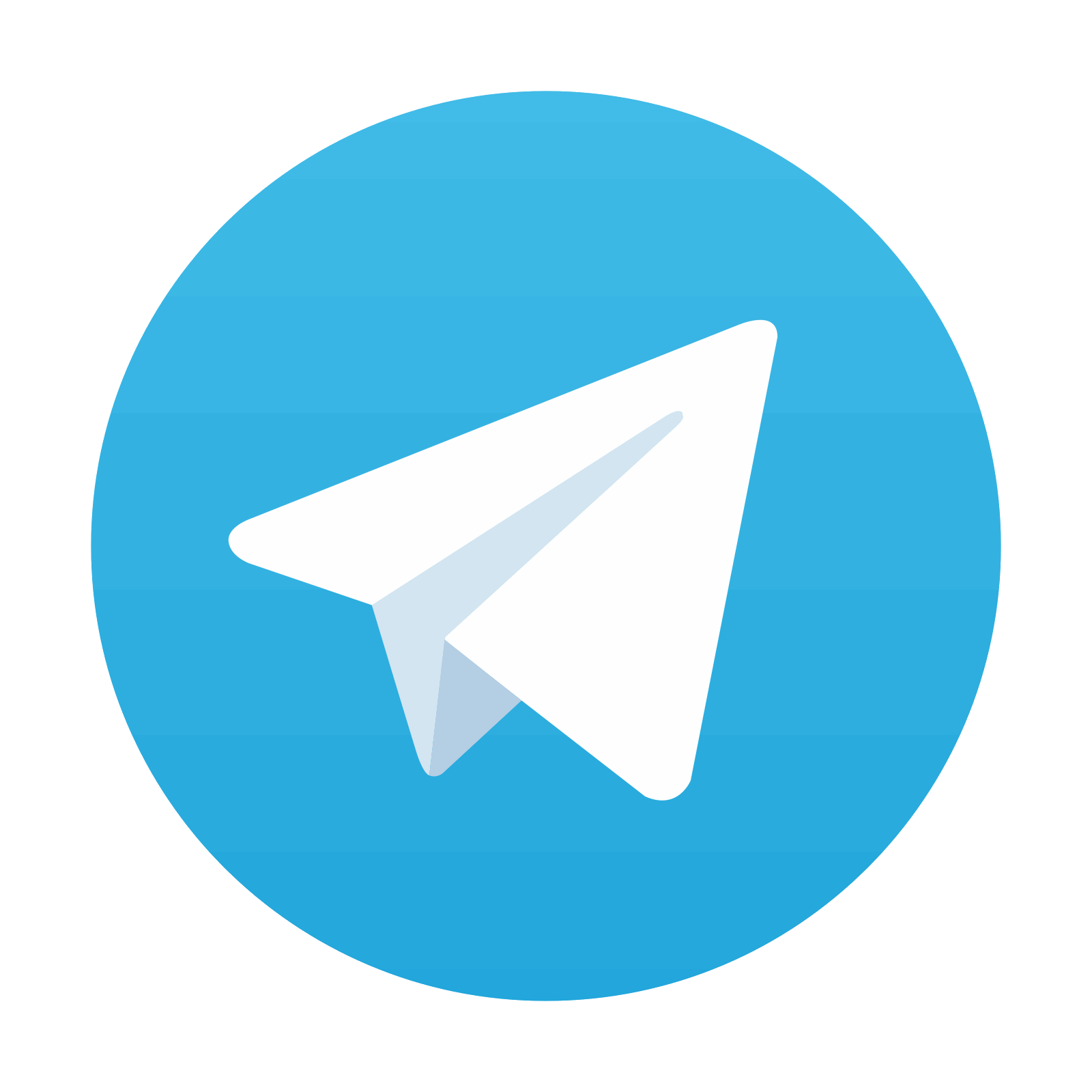
Stay updated, free articles. Join our Telegram channel

Full access? Get Clinical Tree
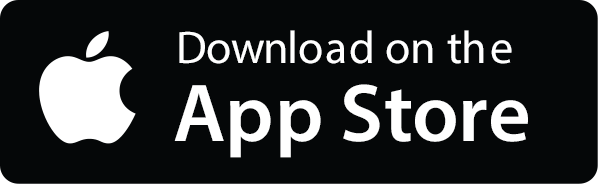
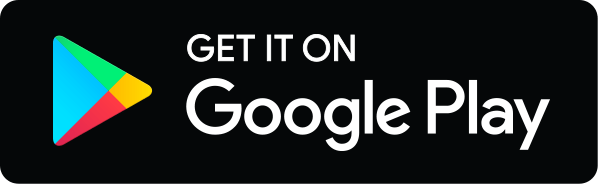