Nitrous oxide equilibration with the vessel-rich group occurs within 5 to 15 minutes from the beginning of induction. The muscle group equilibrates within approximately 1 hour, and the vessel-poor group and fat group equilibrate within 2 to 3 hours.
Factors that govern the elimination of the agent from the body are the same as those that govern the uptake and distribution at the beginning. Hypoventilation, increased cardiac output, highly soluble agents, increased alveolar-to-venous anesthetic concentration gradient, all lengthens the period of emergence.
Diffusion Hypoxia
At the end of an anesthetic, large quantities of nitrous oxide diffuse into the alveoli and dilute the oxygen that is present. This lasts for approximately 10 minutes and, if during this time the patient is allowed to breathe room air, hypoxia may ensue.
Changes in Ventilation
For each 1 mmHg decrease in the PaCO2 caused by an increase in V̇A, an approximate 3% to 4% decrease in cerebral blood flow (CBF) occurs. A change in the length of time of anesthetic induction results from three factors: increased V̇A, decreased CBF, and solubility of the inhaled agents. For a moderately soluble agent like halothane the increased V̇A produces a more rapid rise in end-tidal halothane partial pressure that offsets the decrease in CBF. For a relatively insoluble agent such as nitrous oxide, induction time is increased as the modest increase in end-tidal nitrous oxide partial pressure obtained by hyperventilation is more than offset by the decrease in CBF.
Eventually, no matter what agent is used, the increased end-tidal partial pressure resulting from the decrease in cardiac output and increase in V̇A is enough to overcome the decrease in CBF.
INHALATION AGENTS AND ORGAN SYSTEM FUNCTION
The minimum alveolar concentration (MAC) is the amount of an inhalational agent that prevents movement in 50% of patients in response to surgical incision. After approximately 31 years of age, the MAC value begins to decrease; theoretically, the value for a patient 100 years of age is only 25% to 50% that of a young adult.
Circulatory Effects
Blood pressure is decreased with all inhalational agents and can be due to decreased contractility (e.g., halothane) or decreased systemic vascular resistance (e.g., isoflurane or desflurane) (6). Isoflurane and desflurane lead to coronary vasodilatation and may cause ischemia. The effect of both isoflurane and desflurane on contractility is less than halothane and enflurane (7).
Cardiac Rhythm
Halothane is the most arrhythmogenic agent while enflurane, desflurane, and sevoflurane are the least. Desflurane at a concentration of 6% led to a significant increase in QTc in children, whereas 2% sevoflurane did not (8).
Hypoxic Pulmonary Vasoconstriction
Hypoxic pulmonary vasoconstriction (HPV) is inhibited in a concentration-dependent fashion (9). However, the clinical significance of the difference between the anesthetic agents in this regard is not clear. When looking at a porcine model of one-lung anesthesia, neither isoflurane nor desflurane was found to have a deleterious effect on oxygenation (10).
Respiratory Effects
All inhalational agents are respiratory depressants. Compensatory responses to both hypoxia and hypercarbia are blunted (11).
Hepatic Effects
Up to 20% of patients may demonstrate mild disturbances in liver function following anesthesia with halothane (6).
Neither desflurane nor sevoflurane seems to have hepatotoxic properties (12).
Renal Effects
All potent inhalation agents result in a dose-dependent decrease in renal blood flow. In patients with preoperative renal impairment, anesthesia with either desflurane or isoflurane did not lead to worsened renal function (13).
INTRAVENOUS AGENTS
Narcotics
General Properties
Morphine sulfate was first isolated from opium in 1803. The use of high-dose narcotics for anesthesia was popularized in the 1970s.
The opiate receptor complex has three major receptor groups: μ, δ, and κ. Pain relief is mediated by the μ-receptor and also affect the respiratory, cardiovascular, gastrointestinal, and neuroendocrine systems (14).
Modern anesthesia practice includes morphine, meperidine, methadone, fentanyl, alfentanil, sufentanil, and, more recently, remifentanil. Morphine is associated with recall, histamine release, respiratory depression, hypertension, and vasodilation. Synthetic drugs related to the phenylpiperidines, such as fentanyl, sufentanil, alfentanil, and remifentanil, do not induce histamine release or vasodilation and thus hemodynamically stable (14). Remifentanil, has a rapid onset, metabolized by plasma esterases, and has a half-life of 8 to 20 minutes independent of liver or renal function thus making it ideal in patients following neurosurgical procedures or head injury who need frequent neurologic assessments. However, narcotic drugs cannot be depended upon to provide complete anesthesia alone.
TABLE 54.2 Selected Pharmacokinetic Data for Four Opioids |
![]() |
Pharmacokinetics/Pharmacodynamics
Selected pharmacokinetic data for four commonly used opioids are summarized in Table 54.2. Similarities between the redistribution and elimination half-lives and the clearance and steady-state volume of distribution are noteworthy. The major difference is in lipid solubility, which correlates with potency. The depressant effect from morphine and fentanyl can be seen even after the analgesic effect of that drug has dissipated. Remifentanil has a rapid onset and offset of effect, even after a prolonged infusion.
Hemodynamic Effects
Hypotension is seen with morphine dose of 1 to 4 mg/kg secondary to vagal-induced bradycardia, vasodilation, histamine release, and splanchnic blood sequestration. Hypotension seldom occurs at a rate of 5 mg/min or less.
Treatment with H1 (diphenhydramine) and H2 (cimetidine) blockers attenuates the cardiovascular response to histamine. Fentanyl, 30 to 100 μg/kg, rarely causes hypotension, changes in contractility, heart rate, cardiac output, or systemic or pulmonary artery occlusion pressure even in patients with poor left ventricular function, perhaps because it does not cause histamine release. Remifentanil has a beneficial hemodynamic profile, similar to fentanyl (15).
Respiratory Effects
Dose-dependent respiratory depression is seen. Hypoxic and hypercarbic ventilatory drives are decreased. The pontine and medullary centers for respiratory rhythmicity are impaired, resulting in increased irregular and periodic breathing.
With morphine triggering or worsening of bronchospasm due to histamine release is possible but not seen with fentanyl, sufentanil, or remifentanil. Fentanyl and its derivatives can cause chest wall rigidity. The mechanism is not well understood, but may be a result of γ-aminobutyric acid (GABA) receptor stimulation located on interneurons. Treatment is commonly with a muscle relaxant.
Neurologic Effects
Morphine has no effect on CBF, cerebral metabolic rate for oxygen (CMRO2), or cerebral metabolic rate for glucose (CMRG) in CBF, but autoregulation was better maintained than in the control group (16). Fentanyl, in a model of traumatic brain injury, did not lead to a reduction in CBF despite a decrease in arterial blood pressure (17).
Gastrointestinal Effects
Opioids can cause emesis by stimulation of the chemoreceptor trigger zone in the area postrema of the medulla; increased gastrointestinal secretions; decreased motility that also may affect emetic action; and increased smooth muscle tone of the gastrointestinal tract and the sphincter of Oddi. The effects of opiates on the GIT can be ameliorated by methylnaltrexone which does not cross the blood–brain barrier.
Immune Function
Effects on the immune system are variable but opiates may be associated with suppression of natural killer cells and the immune response in animal studies (18–21).
Barbiturates
Thiobarbiturates (e.g., sodium thiopental and methohexital) are frequently used due to their ultrashort onset and offset action compared with other barbiturates. Methohexital is two to three times more potent than thiopental.
Pharmacokinetics/Pharmacodynamics
Thiopental is highly lipophilic. A dose of 3 to 5 mg/kg induces loss of consciousness within one arm–brain circulation time (10 to 15 seconds). The short duration of action of this drug (5 to 10 minutes) is due to its redistribution from the brain to muscle, skin, and, to a lesser extent, fat. The elimination half-life of the drug is long, making thiopental into a long-acting drug with larger doses.
Methohexital is only slightly less lipid-soluble and the onset and duration of loss of consciousness are approximately the same as with thiopental because of its rapid redistribution, but it is more dependent on hepatic blood flow for clearance.
Neurologic Effects
Barbiturates facilitate inhibitory—GABA—neural transmitters and inhibition of excitatory neural transmitter action and lead an increase in the duration that chloride (Cl−) ion channels remain open. There is a decrease in CMRO2, CBF, and ICP and hence used for brain protection.
Cardiorespiratory Effects
Contractility is decreased with a 10% to 25% decrease in cardiac output, blood pressure, and stroke volume at clinically relevant doses. A decrease in venous tone decreases preload. The responses to carbon dioxide elevation and hypoxia are impaired.
A continuous barbiturate infusion, affects immune function increasing chances of developing infections (22).
Propofol
Propofol is a sedative–hypnotic agent. The agent is not antianalgesic—as are the thiobarbiturates but has minimal amnestic effects (23). It is insoluble in aqueous media and hence available in a 1% weight/volume (Intralipid) emulsion composed of soybean oil, glycerol, and purified egg phosphatide. No histamine release is seen.
Pharmacokinetics/Pharmacodynamics
Propofol is extensively distributed into vessel-rich tissues, and ultimately redistributed to lean muscle and fat. It has high clearance and the short elimination half-life but affected by age (decreased clearance and dose requirement), obesity (increased clearance and volume of distribution), and type of procedure.
A dose of 2 to 2.5 mg/kg results in loss of consciousness in less than 60 seconds; rapid intravenous injection of 1 to 1.5 mg/kg in the elderly or a patient who has been given narcotic or benzodiazepine premedication is often sufficient for induction. The effective dose in 50% of patients studied (ED50), which is analogous to MAC for potent inhalation agents, is 53.5 μg/kg/min (95% confidence limits; 39.9 to 63 μg/kg/min) (24).
Neurologic Effects
The mechanisms of action of propofol are unclear. At a dosage of more than 150 μg/kg/min results in EEG burst suppression lasting 15 seconds or longer returning to the awake state within 11 minutes of drug discontinuation.
Propofol leads to a dose-related decrease in CBF and CMRO2, and leads to progressive EEG suppression with increasing dose of propofol (25) thus beneficial in patients with intracranial disease and for long-term sedation in the ICU.
Cardiovascular Effects
Propofol is a negative inotrope and produces a dose-dependent decrease in systolic, diastolic, and mean arterial blood pressure; an effect enhanced by narcotic premedication. Profound cardiovascular depression may be seen when propofol is used in elderly or hypovolemic patients and those with impaired ventricular function.
Respiratory Effects
Apnea is seen on induction with propofol and the response to hypoxia is also significantly blunted (26). Adjuvant use of narcotics further depresses respiratory drive.
Propofol Related Infusion Syndrome
First described by Parke et al. (27) propofol related infusion syndrome (PRIS) has been described in adults also (28,29). It is associated usually with high dose (>4 mg/kg/hr) and prolonged infusions (>48 hours) but has also been described after short-term infusions (30). A syndrome characterized by unexplained metabolic acidosis, bradycardia, hypotension, arrhythmias, EKG changes consistent with a brugada-like picture, rhabdomyolysis and renal failure (31). Fatty infiltration of the liver is very common early in the syndrome (Table 54.3).
Propofol increases the activity of malonyl CoA, inhibiting the entry of long chain fatty acids into the mitochondria and uncoupling beta oxidation. This leads to accumulation of fatty acids in muscle and other organ systems. This is more pronounced in a high catecholamine, low carbohydrate state as seen in critically ill malnourished populations such as the extremes of age who are at higher risk of the syndrome (32,33).
TABLE 54.3 Clinical Manifestations of Propofol Related Infusion Syndrome (PRIS) |
![]() |
Treatment is largely supportive. Triglycerides levels should be monitored when patients are on propofol.
Benzodiazepines
The benzodiazepines most commonly employed are diazepam, lorazepam, and midazolam. Diazepam and lorazepam are insoluble in water. Lorazepam is less lipid-soluble than diazepam, and its slow entry into the CNS may be significant to its slower onset of action.
Pharmacokinetics/Pharmacodynamics
Diazepam. Intramuscular injection is painful, and absorption is erratic. Clearance involves oxidation to active metabolites. With hepatic disease, the volume of distribution increases and metabolism decreases, resulting in an increase in the half-life from 40 to 80 hours.
Lorazepam. Lorazepam is unaltered by age or renal disease, but hepatic disease increases the half-life.
Midazolam. Midazolam also can be administered by intramuscular, intravenous, or oral routes. The drug undergoes extensive metabolism to active and inactive metabolites.
Neurologic Effects
Benzodiazepines have dose-dependent effects on the CNS and potentiate inhibitory GABA neurotransmission. They increase the frequency but not the duration of chloride channel opening.
Antegrade amnesia is seen with all of the benzodiazepines, but more so with lorazepam.
Ketamine
Pharmacokinetics/Pharmacodynamics
Ketamine is structurally related to phencyclidine, known in street vernacular as “angel dust.” After an intravenous dose of 2 mg/kg, consciousness is lost in little more than one arm–brain circulation time and returns 10 to 15 minutes later secondary to rapid drug redistribution into muscle and other tissues. The most important metabolite is norketamine, and has approximately one-third the potency of ketamine.
Neurologic Effects
The exact mechanism of action of ketamine is not well understood but most likely is NMDA receptor antagonism. It can have hallucinogenic effects including “out of body experiences.”
It is a potent analgesic and a dissociative anesthetic, a feeling of indifference to the outside surroundings but preserved muscle tone. Ketamine increases CBF and so must be used with caution in individuals with elevated ICP.
Cardiovascular Effects
Ketamine causes central stimulation of the sympathetic arm of the autonomic nervous system and the cardiovascular effects are primarily related to CNS stimulation with an increase in systemic blood pressure and cerebrovasodilation, resulting in increased ICP. Pulmonary vascular resistance and right ventricular stroke work also are frequently increased.
Respiratory Effects
Ketamine is not a respiratory depressant; the ventilatory response to carbon dioxide is maintained while potentiating the bronchodilatory effects of catecholamines. Ketamine increases oral secretions, necessitating the use of an anticholinergic agent.
Other Effects
The drug has been used safely in patients with malignant hyperthermia (MH). Postanesthetic emergence reactions—nightmares and hallucinations—occur in 5% to 30% of patients and can be attenuated by using a benzodiazepine.
Etomidate
An induction agent that maintains hemodynamic stability, and hence employed for intubation of critically ill patients with hemodynamic instability. It can cause adrenal suppression when used in a prolonged infusion but has also been demonstrated following a single dose (34). However, the benefits of hemodynamic stability may overcome any concerns about adrenal dysfunction and its consequences (35).
Dexmedetomidine
Dexmedetomidine is a highly selective, short-acting central α2-agonist. It is used in the ICU for providing sedation and some degree of analgesia. It is used primarily for sedation in the ICU. It provides a dose-dependent degree of sedation, analgesia, anxiolysis, and sympatholysis (alpha 2a receptor mediated), inhibits shivering (alpha 2b receptors) and associated with decreased delirium (alpha 2c receptor). When used in postoperative patients in the ICU, dexmedetomidine can provide better sedation with fewer narcotics than propofol (36). It does not cause respiratory depression and hence can be used through an extubation protocol.
It is chemically related to clonidine but has eight times the affinity for alpha 2 receptors.
A biphasic response is seen with initial hypertension and bradycardia followed by a decrease in BP. It can be used perioperatively in cardiac surgery (37) and has some protective effect in brain trauma (38) the MENDS study showed a lower incidence of delirium (39).
ANESTHESIA TECHNIQUES AND SPECIFIC CLINICAL STATES
There is no evidence to conclusively support one anesthetic technique over another in critically ill patients. However, in a hemodynamically unstable patient it is best to avoid potent inhalation agents and prefer an intravenous technique involving either ketamine or one of the phenylpiperidine narcotics. In traumatic brain injury, one can use intravenous benzodiazepines, barbiturates, ketamine and narcotics with isoflurane; while potent-inhaled anesthetic agents are avoided.
Total intravenous anesthesia (TIVA) with short-acting narcotics and propofol allow for an easily titratable anesthesia without the disadvantages of inhalational anesthesia. Closed gas spaces such as pneumothorax, pneumocephalus, or bowel obstruction demand that nitrous oxide be withheld.
Acute Respiratory Distress Syndrome
The development of acute respiratory distress syndrome (ARDS) is hypothesized by a two-hit mechanism. A patient at risk for cytokine release due to various mechanisms (e.g., trauma, sepsis, critical illness, pancreatitis, extensive surgery), if exposed to a second hit such as blood transfusions, high tidal volumes, inappropriate antibiotics can develop lung injury.
ARDS presents with hypoxemia secondary to atelectasis and shunt, thus presenting challenges to mechanical ventilation. Use of low tidal volumes of 6 to 8 mL/kg ideal body weight is preferred (18,40). A higher than normal PaCO2, “permissive hypercapnia” is tolerated. The approach of lung protective strategy has been shown to improve outcome in patients with ARDS and should probably be maintained in patients undergoing surgery (18,41,42). The use of PEEP to prevent derecruitment is important, but the exact amount is still under debate (43–45).
Identifying patients who are at risk for developing lung injury (lung injury predictive score) and avoiding a second trigger such as blood transfusion, high tidal volumes could prevent progression to ARDS (46).
Management of patients with ARDS should be directed at the maintenance of adequate hemodynamics without fluid overload (47,48).
The use of dynamic markers such as pulse pressure variation (PPV) and systolic pressure variation (SPV) may be challenging in a setting of low compliance and low tidal volumes such as ARDS (49). Other techniques such as a response of stroke volume index, cardiac index or increase in end-tidal CO2 (ETCO2) with passive leg raise (PLR) or an end expiratory occlusion could be employed safely in this setting (50–54).
The Patient with a Head Injury
Optimal cerebral perfusion pressure—generally considered to be “optimized” at 60 to 65 mmHg is the goal. Anesthesia directed at reducing intracerebral pressure, barbiturates, maintenance of normothermia or mild hypothermia, increasing serum osmolarity, and judicious use of diuretics, use of vasopressors and inotropes to improve cardiac output and blood pressure and at times, hyperventilation. The approach is to direct therapy to optimize CBF by improving central perfusion pressure.
The Patient with Shock
In hypovolemic, septic shock patients, it is important to minimize intrathoracic pressures during fluid resuscitation. Drugs that depress contractility and cause vasodilation should be avoided. Sometimes in extreme cases, even drugs that are considered to maintain hemodynamic stability, such as ketamine, can lead to hemodynamic collapse.
In patients with cardiogenic shock, intrathoracic pressure is not detrimental, and may even be beneficial. However, most anesthetics are cardiac depressants and should be used with caution in patients with shock. All patients in shock need invasive hemodynamic monitoring to assess fluid responsiveness (55,56).
POSTOPERATIVE CARE
Postoperative complications have been found to occur in 5% to 30% of patients.
Hypoxemia
Postoperative hypoxemia can result from diverse etiologies (Table 54.4).
Hypoventilation caused by residual anesthetic or muscle relaxant especially in patients who are predisposed such as obesity-hypoventilation syndromes, obstructive sleep apnea (OSA), neuro muscular disorders like myasthenia gravis, amyotrophic lateral sclerosis.
Atelectasis could be secondary to one-lung intubation, upper abdominal and thoracic surgery especially in patients who have pre-existing lung conditions such as COPD, kyphoscoliosis.
Note that closing capacity and FRC alter with age and the older individuals are more predisposed. Early recognition of this condition and application of noninvasive ventilation (NIPPV-CPAP/BiPAP, aggressive incentive spirometry) can prevent the complications such as hypoxia and pneumonia.
Upper airway obstruction due to a decreased level of consciousness is a common reason for hypoxemia and hypercarbia especially in patients with OSA. Pulmonary edema could be cardiogenic or noncardiogenic. Noncardiogenic may be due to aspiration, infection, ARDS, trauma, transfusion-associated lung injury (TRALI) or neurogenic pulmonary edema. Pulmonary vascular permeability index can be measured and can differentiate between cardiogenic and noncardiogenic pulmonary edema. Negative-pressure pulmonary edema can develop after a strenuous inspiratory effort against an obstructed airway and can take up to 10 hours after the episode of airway obstruction. It is most commonly secondary to laryngospasm during anesthetic induction of or emergence from anesthesia.
TABLE 54.4 Etiology of Postoperative Hypercapnia |
![]() |
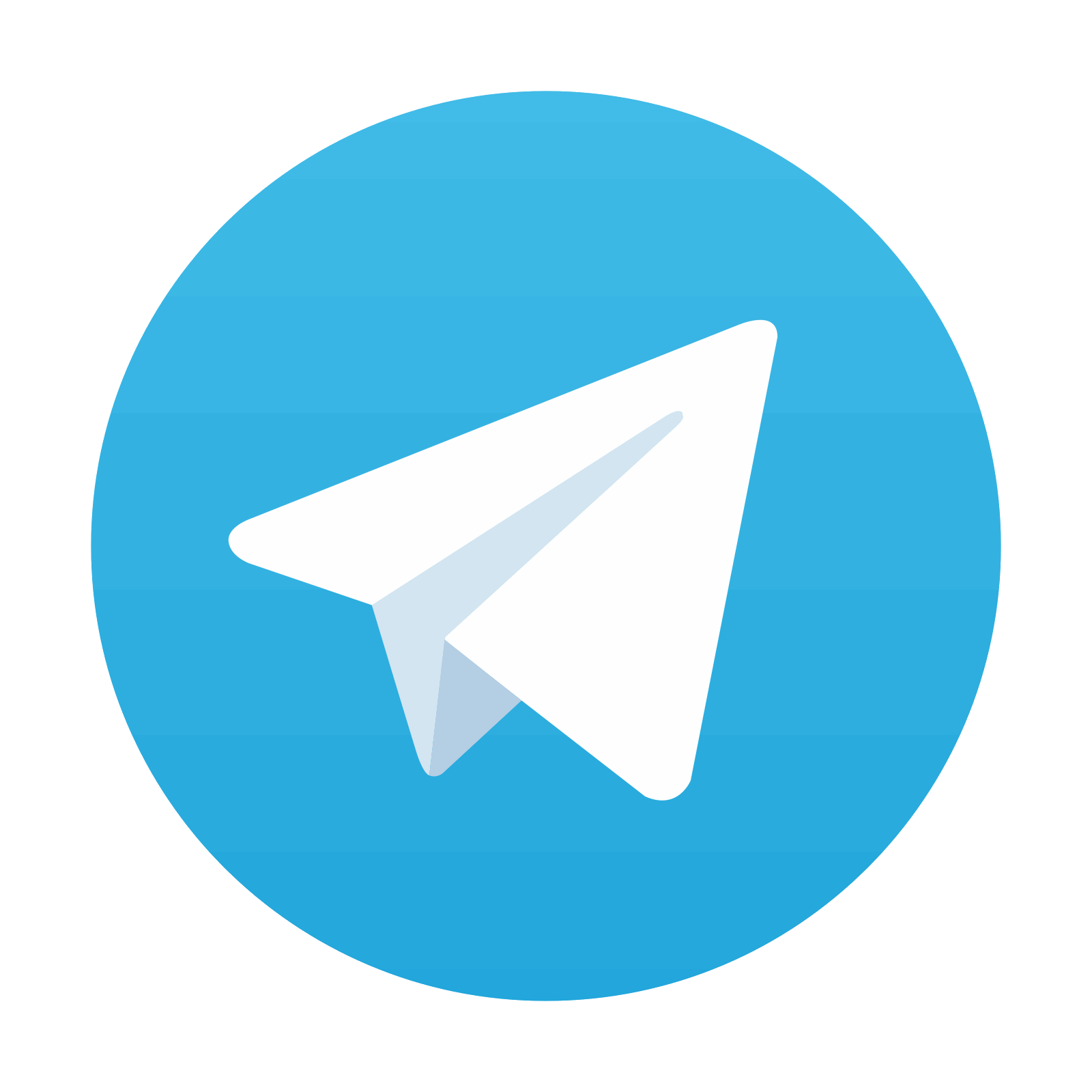
Stay updated, free articles. Join our Telegram channel

Full access? Get Clinical Tree
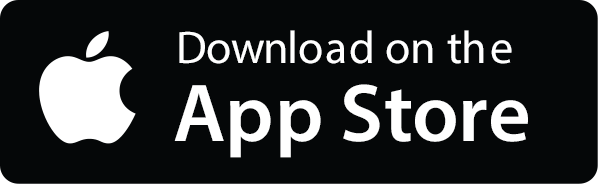
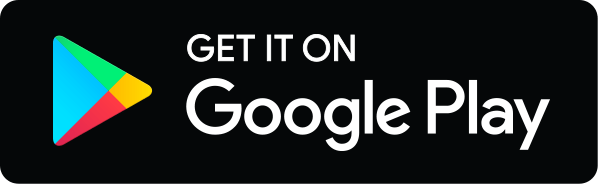