Anesthesia Machine Ventilators
Eric Schnell
Valdez Bravo
▪ INTRODUCTION
A mechanical ventilator is a fundamental component of modern anesthetic practice. The ventilator performs two critical functions for an anesthetized patient: oxygenation and ventilation. Oxygenation supplies the lungs (and hence, the rest of the body) with oxygen. Ventilation delivers oxygen to the lungs and removes waste gases such as carbon dioxide from the lungs, allowing the body to maintain a physiologic acid-base balance. Additionally, ventilation can be used to administer and remove inhaled anesthetics.
While awake, patients maintain spontaneous ventilation (breathing) by generating negative intrathoracic pressures with contractions of the diaphragm, causing air to flow into the lungs (see Chapter 16). After induction of general anesthesia, respirations are often depressed or absent, depending on the choice of anesthetics and muscle relaxants (i.e., neuromuscular junction-blocking agents). At this point, the airway is usually secured with an endotracheal tube (see Chapter 18). Ventilation and oxygenation are then accomplished by administering positive pressure to the patient’s airway, leading to the flow of oxygen and anesthetic gases into and out of the lungs.
When the mechanical ventilator is not engaged, positive pressure can be manually delivered to the breathing circuit by squeezing the breathing reservoir bag with the adjustable pressure-limiting (APL or “pop-off”) valve partially closed (see Chapter 29). Alternatively, the breathing circuit can be placed in continuity with the ventilator, which delivers positive pressure breaths with a desired waveform and frequency. When the positive pressure is released, the patient passively exhales gases, including waste carbon dioxide, back into the breathing circuit.
Although both manual and mechanical ventilation are capable of providing oxygen, delivering anesthetic gases, and removing carbon dioxide, mechanical ventilation has several distinct advantages. First, it frees the anesthesia provider from having to perform continuous manual ventilation throughout the case. Second, it allows the anesthesia provider to set specific pressures and/or volumes for each breath, allowing for more precise control of ventilation than is possible manually. This fine control is critical when small changes in ventilatory parameters are required to optimize a complex patient’s respiratory function. Third, modern ventilators can deliver positive pressure waveforms that are difficult to achieve with manual ventilation, such as pressure control ventilation or end-inspiratory pauses (“holds”). Fourth, modern ventilators are linked to pressure monitoring systems that give important information about lung compliance. Thus, they are able to instantly release positive pressure if a threshold (maximum) pressure is reached, providing an additional measure of safety (for instance, when a patient coughs or strains against the ventilator). Finally, in certain modes, mechanical ventilators can even assist a patient’s spontaneous breathing by detecting a patient’s respiratory efforts and synchronously delivering positive pressure at the appropriate times.
▪ VENTILATOR MODES
Modern ventilators operate in specific modes that define the overall pattern of positive pressure delivery. The anesthesia provider independently programs parameters specific to each patient/procedure. Basic ventilator modes are distinguished based on two important characteristics: whether the ventilator delivers a fixed tidal
volume or a specific inspiratory pressure and whether the ventilator is triggered by an internal timer or by the patient’s own respiratory efforts.
volume or a specific inspiratory pressure and whether the ventilator is triggered by an internal timer or by the patient’s own respiratory efforts.
Volume control ventilation delivers a set volume (also referred to as the tidal volume) with each positive pressure breath. These breaths are delivered at a provider-determined frequency, and thus provide a predictable minute ventilation (Minute Ventilation = Ventilator Rate × Tidal Volume). One major advantage of the volume control mode is the consistency of minute ventilation over prolonged periods of time, even during changes in lung expandability (known as lung compliance—see Chapter 11). For example, if a patient suddenly bears down and stiffens the chest muscles (making the lungs less compliant), the ventilator needs more pressure to expand the lungs than when the patient was completely relaxed. The ventilator will increase the pressure accordingly, and still deliver the same tidal volume as long as the inspiratory pressure limit (Pmax, often set to 40 cm H2O in adults) is not reached. However, once the pressure limit is reached, the ventilator will stop delivering positive pressure and an alarm will sound, warning the anesthesia provider of the high airway pressure. One disadvantage of this mode is that it often requires slightly higher absolute peak pressures than other modes to achieve the same minute ventilation (Fig. 30.1A). Also, the lungs spend less time at larger lung volumes compared to pressure control ventilation, which may decrease the time available for gas exchange in some portions of the lung.
Pressure control ventilation delivers a set plateau level of positive pressure with each inspiration (Fig. 30.1B). The actual tidal volume delivered depends on the patient’s lung compliance: A more compliant set of lungs will expand more easily and thus receive a higher tidal volume at the same pressure control settings than a less compliant (stiffer) set of lungs. One advantage
of this mode is that for any given peak pressure, the patient will spend a larger amount of time at larger lung volumes, and have a larger tidal volume, than that reached during volume control ventilation. It may be particularly useful in patients with very stiff lungs, or those in whom it is otherwise difficult to sustain adequate ventilation without resorting to very high inspiratory pressures. A disadvantage of this ventilator mode is that minute ventilation can change substantially if the patient’s pulmonary compliance changes. However, this can be easily countered by programming the alarms on the ventilator to notify the provider when tidal volumes or minute ventilation fall outside of a predetermined range.
of this mode is that for any given peak pressure, the patient will spend a larger amount of time at larger lung volumes, and have a larger tidal volume, than that reached during volume control ventilation. It may be particularly useful in patients with very stiff lungs, or those in whom it is otherwise difficult to sustain adequate ventilation without resorting to very high inspiratory pressures. A disadvantage of this ventilator mode is that minute ventilation can change substantially if the patient’s pulmonary compliance changes. However, this can be easily countered by programming the alarms on the ventilator to notify the provider when tidal volumes or minute ventilation fall outside of a predetermined range.
![]() ▪ FIGURE 30.1 Pressure-time waveforms of different ventilation modes. A: In volume control ventilation, pressure steadily increases during inspiration, as gas (typically at a constant flow rate) fills the lungs. During expiration, pressure drops quickly as the chest and diaphragm passively recoil. Breaths are delivered at regular time intervals. B: In pressure control ventilation, the ventilator quickly reaches the plateau (control) pressure and maintains this throughout the breath. This requires an increased respiratory flow at the outset of the breath and a small delay for the ventilator to reach the target pressure. Expiratory flows are passive and follow the same waveform as in volume control. C: In pressure support ventilation, the patient initiates each pressure-controlled breath by generating negative pressure (note the downward deflections prior to the pressure support), and thus inspiratory timing is dependent on patient effort. D: In SIMV, a volume-controlled breath is delivered with each inspiratory effort. If the patient fails to initiate a breath after a set interval, the ventilator will deliver a volume-controlled breath regardless. |
Both of the aforementioned ventilator modes are designed to deliver a set number of breaths per minute (the respiratory rate) on a regularly timed basis. This is absolutely necessary when an anesthetized patient is paralyzed and unable to breathe spontaneously. However, many anesthetics do not require complete muscle paralysis, and these patients may continue to make respiratory efforts while fully anesthetized. Modern ventilators are able to assist these efforts by synchronizing positive pressure delivery with a patient’s own respiratory rate. This assistance helps to counter the respiratory depressant effects of many anesthetics and the increased work of breathing through the resistance of an endotracheal tube.
In pressure support ventilation (sometimes referred to as PSV-Pro ventilation), the ventilator senses a patient’s inspiratory effort as a drop in breathing circuit pressure and delivers a set level of positive pressure to augment the patient’s tidal volume (see Fig. 30.1C). In this mode, the patient is less likely to breathe in opposition to the ventilator, and thus less likely to cough or strain. As this mode depends on the patient’s effort to initiate an inspiratory cycle, if the patient stops making ventilatory efforts (or these efforts are too weak to trigger the ventilator), he or she receives no ventilation. For this reason, pressure support modes often have a backup mode that automatically begins timed positive pressure ventilation after a specified interval if no breaths are detected (often 30 seconds).
One last commonly employed ventilator mode, synchronized intermittent mandatory ventilation (SIMV), delivers a specified volume synchronously with a patient’s respiratory efforts (Fig. 30.1D). However, it is also programmed to deliver the same volume after a specified time even if the patient has not made any inspiratory breaths during this interval.
More advanced modern ventilators function using combinations of several of the above characteristics. Additionally, more detailed settings such as the ratio of time the ventilator spends in inspiration versus expiration during each respiratory cycle (the I:E ratio) can be changed, which may be useful in managing clinical situations such as bronchospasm.
One commonly used, and clinically important, ventilator function is the ability to maintain positive pressure between breaths, called positive end expiratory pressure (PEEP). Without PEEP, the patient exhales gas back into the circuit until the airway pressure reaches atmospheric pressure. With PEEP engaged, expiratory flow is mechanically stopped when the desired expiratory pressure is reached, and thus positive pressure is maintained until the next inspiratory cycle. PEEP
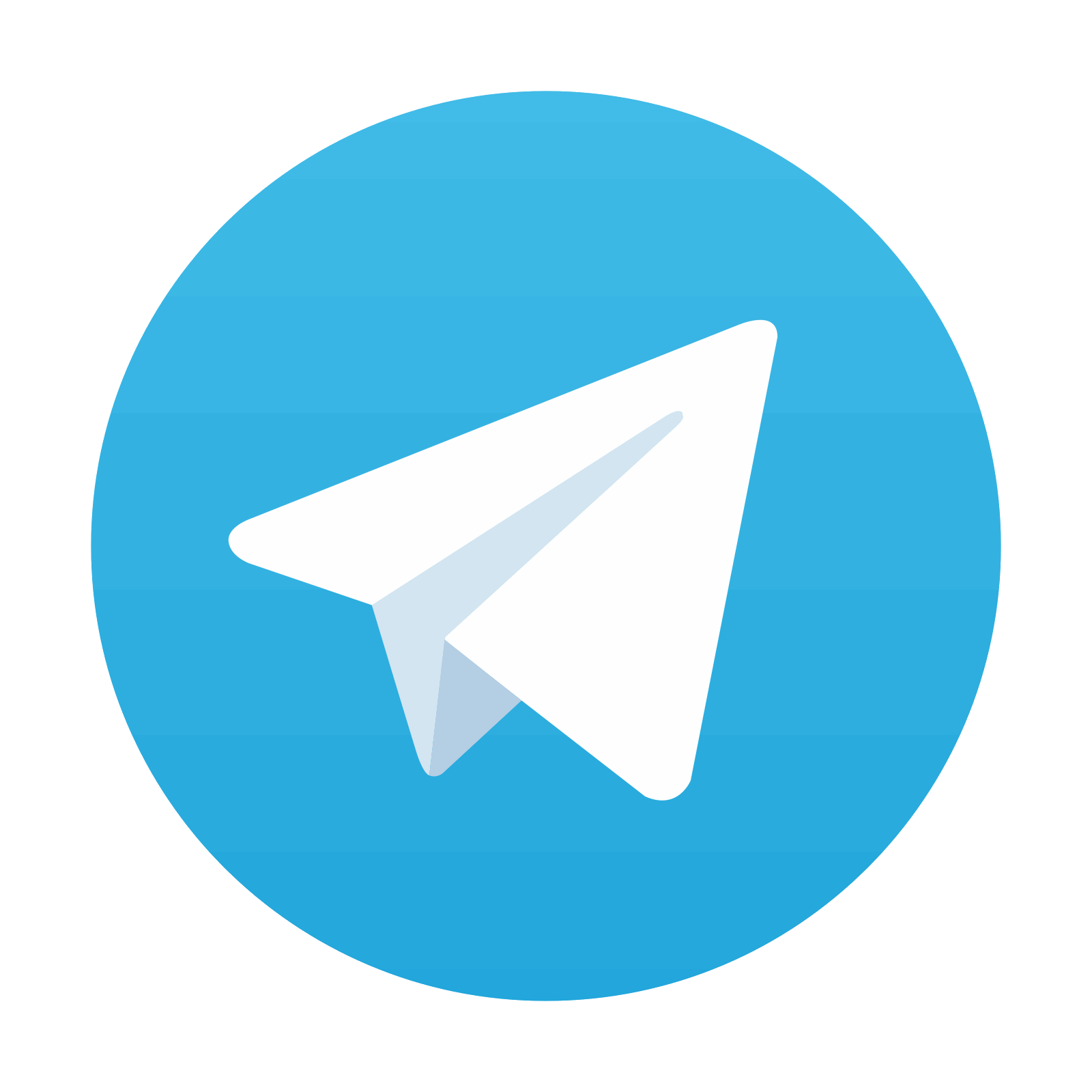
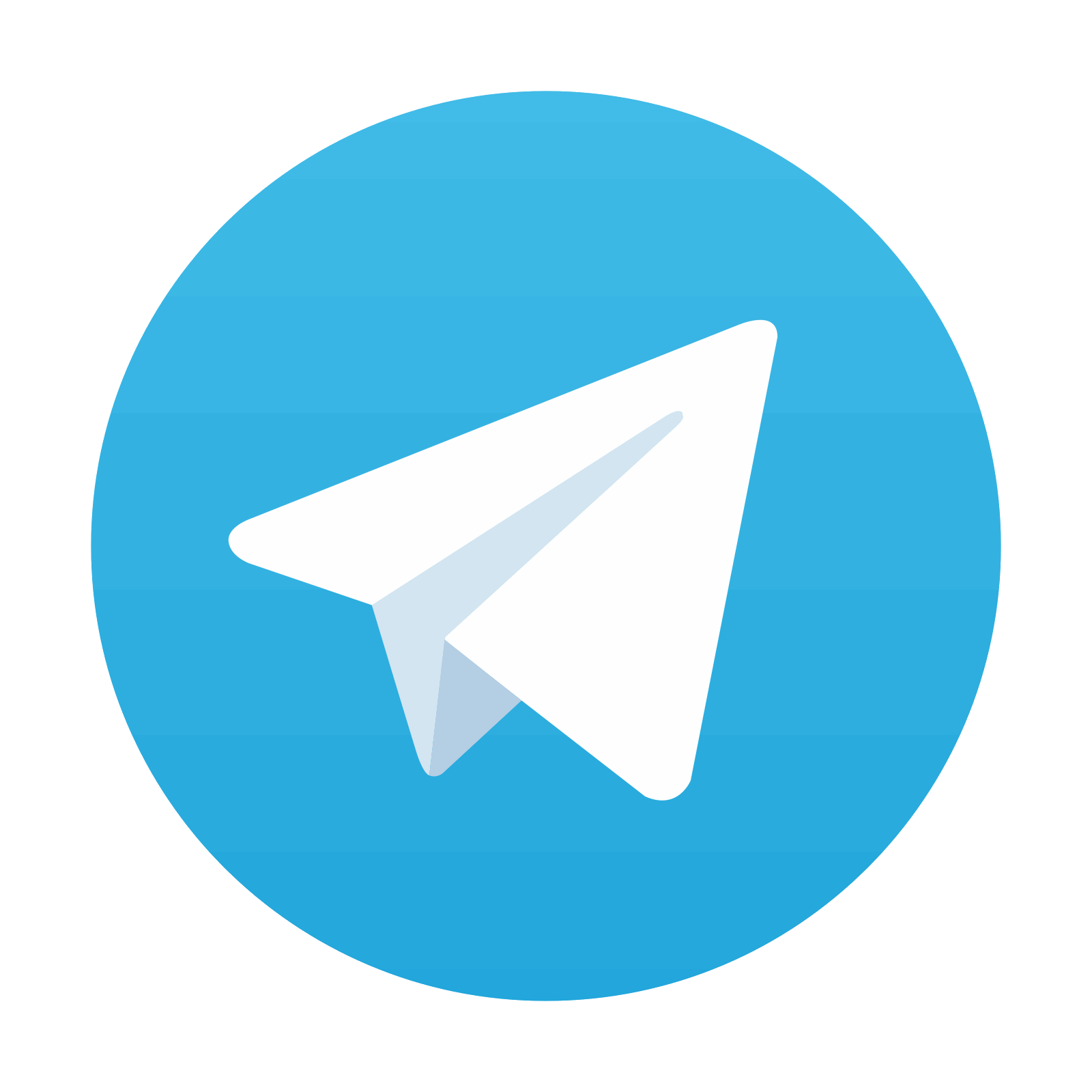
Stay updated, free articles. Join our Telegram channel

Full access? Get Clinical Tree
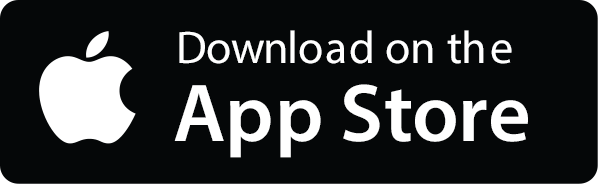
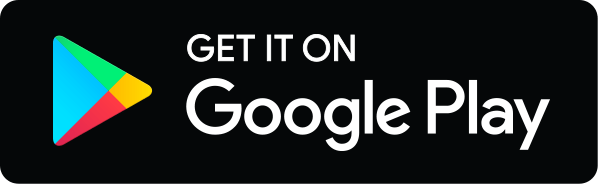