Fig. 5.1
Anesthesia workstation
The anesthesia machine is designed to supply medical gases from a gas supply, then mix the gases with inhalational agents at desired concentrations, and deliver the final mixture at a desired and safe/reduced pressure to the breathing circuit that is connected to the patient’s airway. Newer machines are being manufactured, which are smaller and lighter, provide enhanced patient safety features and advanced ventilation modes, and allow automated record keeping and new monitoring capabilities.
Components of the Anesthesia Machine
The anesthesia machine functions with pneumatic as well as electrical components (Fig. 5.2a, b).


Fig. 5.2
Anesthesia mechanic circuit diagram, (a) and (b)
Pneumatic Components
The pressures in the machine can be used to classify the system into three parts:
High-pressure system: This starts from the cylinders and ends at the primary pressure regulator and receives gases at cylinder pressure. This system includes the hanger yoke (including filter and unidirectional valve), yoke block, cylinder pressure gauge, and cylinder pressure regulator.
Intermediate-pressure system: This starts from the pipeline inlet or downstream of cylinder pressure regulator (above) to the flow control valve and includes components that receive gases at reduced and constant pressures (37–55 psi), which is the pipeline pressure. This system includes the pipeline inlets and pressure gauges, ventilator power inlet, oxygen pressure-failure device (fail-safe) and alarm, flowmeter valves, oxygen and nitrous oxide second-stage regulators, and the oxygen flush valve.
Low-pressure system: This starts from the flow control valve (above) to the common gas outlet and receives gases slightly above atmospheric pressure (but less pressure than the intermediate-pressure system). This system includes flowmeter tubes, vaporizers, check valves, and the common gas outlet.
Electrical Components
Master switch: In most machines both electrical and pneumatic functions are activated by the master switch.
Power failure indicator: It warns the administrator of the failure of main power. Alarms may be visual and/or audible.
Reserve power: This is “backup” power, which is available (for at least 30 min) in case of loss of main power and needs to be checked regularly. Individual monitors may have their own reserve batteries or may draw from the reserves of the machine.
Automated machine checkout: if available, should be done before the cases are started in the morning. A manual check should be done before starting every case and a full logout and recheck should be done at least every 24 h. Bypass for automated checkout is available; however, bypassing the automated checkout should be avoided.
Electrical outlets and circuit breakers on the machine: The electrical outlets should be used for anesthesia monitors only. When the circuit breakers are activated, the electrical load should be reduced and the breakers should be reset
Data communication ports: provide information between the machine, monitors, and data management system.
Medical Gases
“Medical gases” are undoubtedly the most commonly used drugs throughout the world. On an average a 300 bedded hospital in USA consumes at least around 450 gallons of oxygen daily. To deliver medical gases to “point of care,” the supply systems use cylinders or pipeline system.
Physics Governing Gas Storage
The primary aim of medical gas storage systems is to store maximal amount of usable gas in minimal volume. For this the gases need compression or even conversion to liquid form by alterations in storage pressure, temperature, or both. The expansion ratio (volume of gas generated per mL of cryogenic liquefied gas) for medical gases is around 800 mL of gas/mL of liquid. The following principles/gas laws affect medical gas storage:
Critical temperature: It is the temperature of a gas above which it cannot be liquefied, irrespective of the amount compression pressure applied on it. Thus for any gas whose critical temperature exceeds that of operating room (OR) temperature (around 20 °C), it cannot be stored in liquid form in the OR. Alternatively these gases are stored in pressurized cylinders.
Boyle’s Law: The absolute pressure exerted by a given mass of an ideal gas is inversely proportional to the volume it occupies, if the temperature and amount of gas remain unchanged within a closed system.
In simple terms it means the higher the pressure applied on the gas, the lower the volume it occupies. For medical cylinders the highest applicable pressure is limited by the tensile strength of medical cylinders. The safety of peak pressures, once gases are compressed in cylinders, is dependent upon their ambient temperature.
Gay-Lucca’s Law: In a fixed volume (cylinder), an increase in gas temperature increases its pressure. Thus if cylinder is in a hotter climate, its pressure can increase significantly crossing the safety limit.
Medical Gas Supply Source
Medical gases are delivered to the anesthesia machine either by pipeline or via cylinders.
Pipeline Supply
This is the primary source of gas supply in the hospital. A central piping system is used to deliver oxygen, nitrous oxide, and air, usually at pressures of about 50 psi. Both oxygen and nitrous oxide are stored as liquids in large tanks. The pipelines are gas specific and coded with the gas name and specific color. In addition, for correct connections, the diameter index safety system (DISS) at the machine end and noninterchangeable quick coupler’s (NIST) or Schrader’s probe at the terminal wall units are incorporated to prevent accidental crossing of gases.
A check valve distal to the pipeline inlet prevents backflow of gases (reverse flow from the machine to the pipeline) or leaks to the atmosphere. The pipeline pressure indicator indicates the gas inlet pressures. To minimize pressure fluctuations when the oxygen flush valve or the ventilator is in use, two-stage pressure regulators further reduce the pressures (both pipeline and cylinder pressures) to 20 psi for oxygen and 38 psi for nitrous oxide.
Gas Distribution and the Pipeline System
Maintaining large cylinders, gas reservoirs, and cryogenic liquid gases at the point/site where these gases are used (operating room) is neither safe nor practical. Components of the gas distribution system that deliver these gases at “point of care” are:
Gas source: Cylinder manifold, cryogenic liquid gas reservoirs, as per National Fire Protection Agency (NFPA) standards, must be located in open remote areas with bulk (liquid) oxygen reservoir having at least a 2-day hospital supply and a backup high-pressure H-cylinder manifold supply of at least one day. Each H-cylinder holds up to 6,000 L of oxygen or 16,000 L of nitrous oxide.
Connecting pipeline system: Copper-based piping system receives gases at a pressure of 50–55 psi and should be capable of withstanding at least 150 psi for safety purposes. Recommended outer diameter of oxygen pipeline must be ½ an inch, whereas for all other gases, it should be 3/8 of an inch. Additional safety features in this system include:
Pressure relief valves: If the pressure exceeds by 50 % of the working pressure, the valve allows a deliberate leak to prevent buildup of pressure in the system.
Shutoff valves: These prevent pressure transmission downstream, thus allowing for pipeline maintenance/cleaning or preventing gas-related hazards by shutting off gas supplies.
Terminal outlet units: These are units to which the user connects the medical devices that use the gas supplies. Common terminal outlets include wall-mounted outlets, ceiling-mounted pendants (as in intensive care units), or ceiling-mounted hoses (in OR). The safety features of these units include:
Automatic shutoff valves: These are self-sealing valves that shut off gas flow when no device is connected to them. Inserting the connecting male probe of concerned device into the terminal socket allows gas flow automatically. They prevent any gas wastage/leakage when the system is not in use.
Gas-specific connectors: The terminal unit of specific gas outlet has a unique configuration (female connector) that only allows connection to the corresponding male inlet connector from the medical device. Thus the possibility of wrong gas inflow to the patient is prevented. The two systems of specific connectors (socket assembly) used in most hospitals are:
Diameter index safety system (DISS) (Fig. 5.3a): was developed as a standard to provide noninterchangeable connections, which are removable, exposed, and threaded connections. The DISS can be used in conjunction with individual gas lines delivering gas at pressures of up to 200 psi. Each DISS connection consists of a body adaptor, nipple, and nut. As the inner diameter of the body adaptor increases or decreases, the diameter of its mating nipple increases or decreases proportionally. In this way, only the properly mated and intended parts fit together (because of a unique thread engagement).
Fig. 5.3
(a) Diameter index safety system, (b) quick connectors
Quick connectors (Fig. 5.3b)—Like the DISS system, they also allow a unique male probe from the equipment to fit into the specific female socket of the gas outlet. The advantages of these connections are that they are easier to engage and disengage, requiring minimum force, and can be done by a single hand. These connectors are, however, associated with a higher incidence of gas leaks when compared to DISS. The mechanism preventing a wrong connection is quite simple; the male probe either has a gas-specific shape or has two different mating portions with specific distance or orientation for each gas. The corresponding female socket has a configuration that allows only one specific complimentary male probe to be inserted.
Cylinder Supply
Gas cylinders are available for oxygen, nitrous oxide, and air (Table 5.1). These cylinders are color coded with cylinder labels and the Pin Index Safety System (PISS) to prevent gas delivery errors. Additionally, a safety relief valve opens in case of extreme pressures within the cylinder. A check valve prevents gas transfer between empty cylinders and minimizes leakage of gas to the atmosphere. The hanger yoke assembly orients the cylinders and maintains a unidirectional gas flow.
Table 5.1
Medical gas cylinders
Medical gas | Form in cylinder | E cylinder capacity | Color | Maximum pressure (at 20 °C) |
---|---|---|---|---|
Oxygen | Gas | 600–700 | Green | 1,800–2,200 |
Air | Gas | 600–700 | Yellow | 1,800–2,200 |
Nitrous oxide | Liquid | 1,600 | Blue | 745 |
The cylinder contains gases at high and variable pressures which are inappropriate for direct use. Therefore, the pressure is reduced to a lower and constant pressure by the primary pressure regulator. Bourdon’s pressure gauge is used to measure the pressure of gas inside the cylinder. This has a flexible curved tube that proportionately straightens out when exposed to gas pressure.
A full oxygen cylinder has a pressure of 2,200 psi, while a full nitrous oxide cylinder has a pressure of 745 psi. The pressures reflected are true indicators of residual gas pressure in oxygen cylinders but not in nitrous oxide cylinders, since nitrous oxide is in the liquid form. The pressure in a nitrous oxide cylinder will read 745 psi until it is 1/4 (400 mL) full. If both the cylinders and the pipeline supplies are kept open, the slightly lower pressure in the cylinder pipeline (45 psi) facilitates the preferential use of main pipeline supply. However, the cylinders should be kept closed after daily checks to prevent their unnoticed use in the event of pipeline gas supply failure, since cylinders are mainly a backup source.
Safe Practices for Handling Cylinders
Regular checkup of cylinders for leaks, erosions, or any physical damage.
Store cylinders in cool, dry places away from any possible inflammable source.
When using the anesthesia workstation, check “pin index” match and also use “Bodok seal” (a washer preventing leaks at contact cite between yoke and cylinder nipple).
Cylinder gas must be free from any moisture; otherwise the escaping gas can lead to icing and occlusion of the nipple (especially nitrous oxide).
Avoid damage to outlet valve—cylinders with “bull nose” (output valve with side “L” angulation, cylinder type F, G, H) should be stored in vertical position, whereas cylinders with “pin index” valves (without any angulation) can be stored in horizontal position.
Quality assurance tests must be performed as per manufacturer’s recommendations (usually at 5-year intervals).
Types of Medical Gases
Oxygen
Commercially available oxygen is produced either by fractional distillation of liquefied air or by using oxygen concentrators. Modern zeolite-based oxygen concentrators are capable of producing up to 10 L/min of oxygen with 99 % purity. Oxygen can be stored in high-pressure cylinders as a gas for mobile use or in the liquid form for hospital use. The “E”-type manifold cylinder is the commonly used mobile/rescue oxygen source. Estimation of residual time for oxygen supply in an “E” cylinder can be calculated as:


A full oxygen cylinder (600–700 L) at 18–2,200 psi will run for approximately 1 h at a 10 L/min flow. So if flow is halved, the time doubles, or if pressure is halved (half-full cylinder), the time will be halved. Recently, high-pressure oxygen cylinders (pressures up to 3,000 psi) have been made available, especially for remote locations. A pressure-reducing valve reduces cylinder pressure to a standard working pressure of about 45–50 psi.
For hospital pipeline supply, oxygen is stored in the liquid form and used via the “vacuum insulated evaporator system.” This is considered as the most efficient and cost-effective method of storing oxygen. Using cryogenic, high-pressure principle (−160 °C, 5–10 atmospheres), oxygen is stored in the liquid form and capable of generating 842 mL of gas/mL of liquid. In contrast, a regular cylinder delivers only 137 mL of gas/mL of cylinder volume. Thermal insulation is a prime requirement, which is maintained by creating a vacuum between the inner steel and outer carbonated steel vessel.
Nitrous Oxide
Nitrous oxide (N2O) is produced by controlled heating of ammonium nitrate to a temperature of 250 °C. Hospitals store N2O in high-pressure and high-capacity (16,000 L each) H-cylinders, which are connected by a manifold. Owing to its high critical temperature (36.4 °C), which is above OR room temperature, N2O is stored in cylinders as a liquid at OR temperature. Thus, the pressure in a nitrous oxide cylinder is not proportional to the volume of gas and will always read 745 psi until it is 1/4 (400 mL) full. The estimation of residual amount of N2O can only be done by weighing the cylinder and subtracting it from the tare weight (weight of empty cylinder) stamped on the cylinder.
As a safety feature, nitrous oxide cylinders are not fully filled with the liquid, as any accidental increase in temperature can lead to vaporization of liquid, increasing pressures tremendously to a dangerous level. However, all cylinders are equipped with a pressure relief valve, which is designed to open at 3,300 psi, well below the “E” cylinder’s maximum pressure threshold of 5,000 psi.
Medical Air
Air is being more commonly used during anesthesia to offset the side effects of N2O or developing oxygen toxicity. Atmospheric air on compression, after passing through a series of driers and filters to remove impurities, is labeled as medical grade air. As per US pharmacopeia, it must contain 19.5–23.5 % oxygen and less than 0.001 % carbon monoxide. Special considerations are given to remove moisture, particulate matter, bacteria, and oil (a contaminant from the compressor system). Recently, synthetic medical air has been developed by using a mixture of liquid oxygen and nitrogen. Synthetic medical air has the advantage of being free from impurities, with the manufacturing process being easy without the need of using special compressors.
Heliox
Heliox is a mixture of oxygen and helium in varying proportions. Because of its low density, it is useful in airway obstruction as it provides laminar flow. The mixture is named on the basis of its oxygen concentration. For example, a 20 % oxygen and 80 % helium mixture is labeled as heliox-20. Approved mixtures are the heliox-20 and heliox-30, which have a density of almost 1/3 of air. Heliox is stored as a compressed gas, and oxygen flowmeters are used to measure its flow/output.
Xenon
Xenon is a recent addition into the list of medical gases, but it is yet to be licensed for its use for anesthesia maintenance. It is almost five times denser than air and is supplied in a low-pressure compressed gas cylinder.
Fail-Safe Safety Devices: Oxygen Supply Pressure Failure
These fail-safe safety devices are linked either mechanically, pneumatically, or electronically and proportionately reduce or completely shut off supply of all other gases, except air, when the oxygen pipeline pressure falls to below 50 % of “normal” supply or usually less than 30 psi, in order to provide a minimum oxygen concentration of 23–25 % at the common gas outlet. These safety devices are present in gas line supplying all flowmeters, except the one for oxygen. Gases such as air and helium may not be linked with these systems.
Fail-safe safety devices can prevent the delivery of a hypoxic gas mixture to the patient, only if it is confirmed that the correct gas is flowing through the pipeline, as they only sense the loss of pipeline pressure. The administration of a hypoxic mixture can occur in spite of these safety devices in the following instances: if there is supply of wrong gas, use of inert gases, leak downstream of flowmeters, defective mechanics, or addition of low-potent gases in high concentration:
1.
The fail-safe valve (Fig. 5.4) is located downstream of nitrous oxide supply source and is controlled by the oxygen supply pressure. In Datex-Ohmeda machines, the fail-safe valve is also called pressure sensor shutoff valve and has a threshold of 20 psi to shut off other gases. North American Dräger has an oxygen failure protection device (OFPD), which is based on a variable flow-type proportionating principle, to interface the oxygen pressure with that of other gases.


Fig. 5.4
Fail-safe valve
2.
Newer Datex-Ohmeda machines have a Link 25 proportion-limiting control system (Fig. 5.5), which maintains a minimum 1:3 O2:N2O concentration or prevents delivery of less than 25 % of oxygen. Also, a pressure sensor shutoff valve is present with a threshold of 26 psi for oxygen, at which it completely shuts off N2O flow.


Fig. 5.5
Ohmeda Link-25 proportion-limiting control system
3.
Newer Dräger machines have an oxygen ratio monitor controller (ORMC), which shuts off nitrous oxide when oxygen pressure falls below 10 psi. Other Dräger machines have a sensitive oxygen ratio controller (S-ORC), which shuts off nitrous oxide when oxygen flow drops below 200 mL/min.
4.
The Penlon machines have a paramagnetic oxygen analyzer which gives off an audible alarm when the oxygen concentration falls below 25 % and also simultaneously cuts off nitrous oxide supply.
5.
Some machines are equipped with the minimum mandatory oxygen flow sensor of 50–250 mL/min.
6.
Oxygen supply failure alarms are medium priority alarms, which can be audible, visual, or both, and activated within 5 s of oxygen supply pressure failure and cannot be disabled. Some machines have a Ritchie whistle, which is an audible alarm that gets activated when the pressure drops below 38 psi and sounds till the pressure falls to 6 psi.
7.
A gas selector switch installed in some machines prevents simultaneous use of air and nitrous oxide.
8.
The oxygen flush valve receives gases from the cylinders or pipeline at 45–55 psi and is directly connected to the common gas outlet, bypassing the flowmeter and vaporizers. It is a self-closing device, can be operated with single hand, used for rapid refill or flushing of the breathing circuit, and provides 100 % oxygen at flows of 35–55 L/min. If the oxygen flush valve is faulty, it can cause barotrauma or dilution of inhaled anesthetic gases, potentially leading to intraoperative awareness.
Flowmeters
Flowmeters are deigned to precisely control and deliver gases to the common gas outlet over a range of flows. The flowmeters can be either an electronic type or the constant-pressure variable-orifice type. They are calibrated for specific gases at 20 °C at an ambient pressure of 760 mmHg. The flow rate through the vaporizer can be low and laminar (depends on the viscosity of the gas) or high or turbulent (depends on the density of the gas).
The flowmeter (Fig. 5.6) is composed of the body, stem, seat, and the control knob. Flowmeters consist of either a single- or double-tapered glass tube in series (Thorpe tube), mounted on a panel of fluorescent coating. Flowmeters are color coded, have an interior antistatic coating, and have knobs with a high torque to prevent changes from casual contact.


Fig. 5.6
Flowmeter
The oxygen knob may be larger, fluted, and protrude further and is positioned the last in sequence or farthest to the right (nearest to the outlet) to prevent delivery of a hypoxic mixture in the event of a leak (Fig. 5.7). The flow rate is measured with either a plumb bob-type float (read at the top) or the ball-type float (read at the center), which rotates with the flow of gas. The float is also coated with antistatic material to prevent sticking and has float stop which stops in full on/off position.


Fig. 5.7
Flowmeter arrangement
Near the bottom of the flowmeter tube, the diameter is small, and as the flow of gas is initiated, it creates pressure to lift the bobbin/float up. As the float rises, the tube orifice widens (tapering tube) allowing more gas to pass around the float. The float will rise until the pressure above and under the float equilibrates and supports its weight. If gas flow is increased further, the float will rise again until its weight is supported (Fig. 5.8). Therefore, gas flow in the flowmeter not only depends on the diameter of the tube but also on the weight and cross-sectional area of the float.


Fig. 5.8
Workings of a flowmeter
In electronic flowmeters, gas flows across a needle valve in a fixed volume chamber. As the flow increases, the pressure increases, and the solenoid valve opens to let out the gas when a specific pressure limit is reached. The flow/min is related to the number of times the valve opens.
Air may directly reach the flowmeters to allow its administration in the absence of oxygen. However, for other gases, the flow is permitted only if there is sufficient oxygen pressure. Most anesthesia machines also have an auxiliary O2 flowmeter with its own flow control valve, flow indicator, and outlet, providing a maximum flow of 10 L/min. The auxiliary oxygen port can be used to provide oxygen to the patient, for driving the ventilator, or for jet ventilation, and can be used without turning the anesthesia machine on.
Anesthesia Breathing Circuits
An “anesthesia circuit” is defined as an assembly of components that connect the patient’s airway to the anesthesia machine, creating an artificial atmosphere from and to which the patient ventilates. They are designed for either spontaneous or positive pressure ventilation, while simultaneously allowing a safe and convenient method to deliver inhaled anesthetic agents. Over the last two centuries, these circuits have evolved from the simple Schimmelbusch’s mask to the modern circle system.
Requirements of a Breathing System
The requirements, both essential and desirable, of an ideal breathing system are described below. The circuit must be capable of:
(a)
Delivering the gases from the machine to the alveoli: to the nearest possible concentration that is set manually. In the process of delivery, it must be capable of rapid changes in the concentration. If the circuit volume is large, alterations made in fresh gas flow rate may take a long time to reach equilibrium with that being delivered, and it may fail to meet the target concentration in an optimal time frame. The factors that add to discrepancy between the set and delivered concentration are rebreathing, air dilution, leaks, anesthetic agent uptake, and agent expired by the lung.
(b)
Eliminating carbon dioxide effectively: from the gases being breathed in. This forms the basis of “efficiency of the circuit.”
(c)
Minimal dead space: Dead space of a circuit is defined as “the volume of the breathing system from the patient-end to the point up to which to and fro movement of expired gas takes place.” Dead space is responsible for not only increasing rebreathing in the circuit but also increasing the work of breathing in a spontaneously breathing patient. In a circle system, the dead space is limited to beyond the point where the inspiratory and expiratory limbs unite (Y piece) and includes the endotracheal tube (Y piece to the ETT). The circuit tubing length does not affect the dead space.
(d)
Minimal possible resistance: Increase in circuit resistance offers resistance to deflation of lungs (expiration), which is a passive process. The overall resistance offered by a circuit can be estimated by Hagen-Poiseuille’s equation, that is,
where k is a constant. The above equation forms the basis of designing an optimal anesthesia circuit with the aim of lowering the work of breathing. The above equation also highlights that the radius of the tubing is the most substantial determinant of overall resistance, and a mere reduction of the radius to half increases the resistance to 16 times. Additional factors that can cause an increase in circuit resistance are valves in the circuit, acute bends, and turbulent gas flows (at high gas inflow rates).

(e)
Fresh gas economy: The anesthesia circuit must use the lowest possible volume of fresh gas inflow to eliminate CO2 and prevent rebreathing.
(f)
Heat and moisture conservation: An ideal breathing circuit must try to conserve the heat and humidity in the expired gas, which helps to maintain physiological function and ciliary motility of respiratory mucosa. The inspired gas is often dry and cold, which can lead to significant heat and water loss.
(g)
Light weight: Lighter circuits add to portability and also prevent drag on the patient’s “airway device” or mask. This property adds significantly to convenience and safety in use of a circuit.
(h)
Universal for age: If the breathing circuit can be used over a wide range of ages, it will add to user acceptability significantly.
(i)
Scavenging: An anesthesia circuit should be free from leaks and allow for collection of exhaled gases effectively by providing a common accessible exit point.
Classification of Breathing Systems
Breathing systems can be classified depending on the amount of rebreathing of gases as open, semi-open, semi-closed, and closed (Table 5.2). In the semi-open system there is no rebreathing of gases, but it requires high fresh gas flows, while in the semi-closed and closed systems, there is rebreathing of exhaled gases after absorption of carbon dioxide. The use of carbon dioxide absorbent prevents the rebreathing of carbon dioxide, while allowing rebreathing of inhaled agents and other gases. In a closed system the inflow of gas exactly matches the take-up or consumption. The semi-closed circle breathing system is the most common type of circuit used (see below).
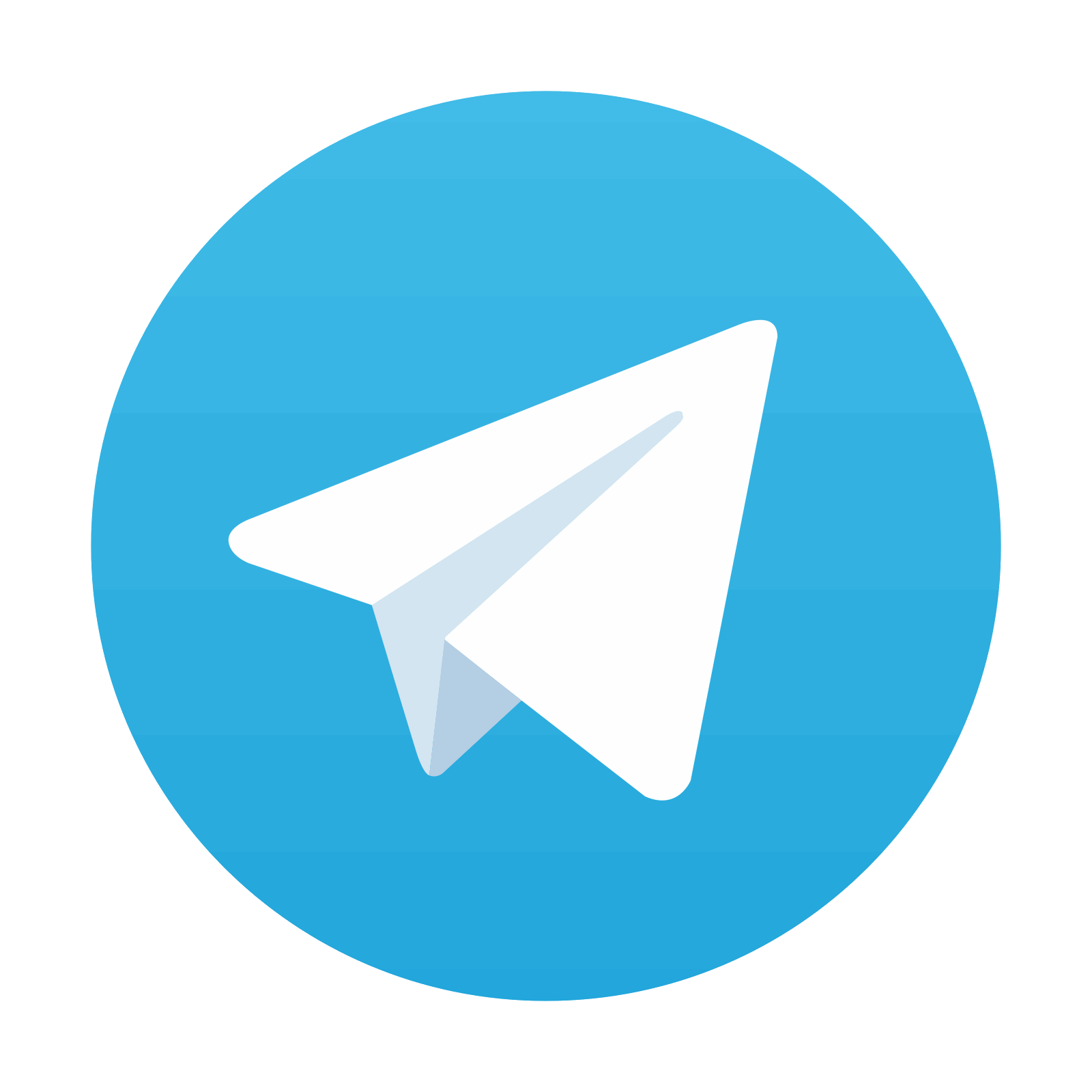
Table 5.2
Classification of breathing systems
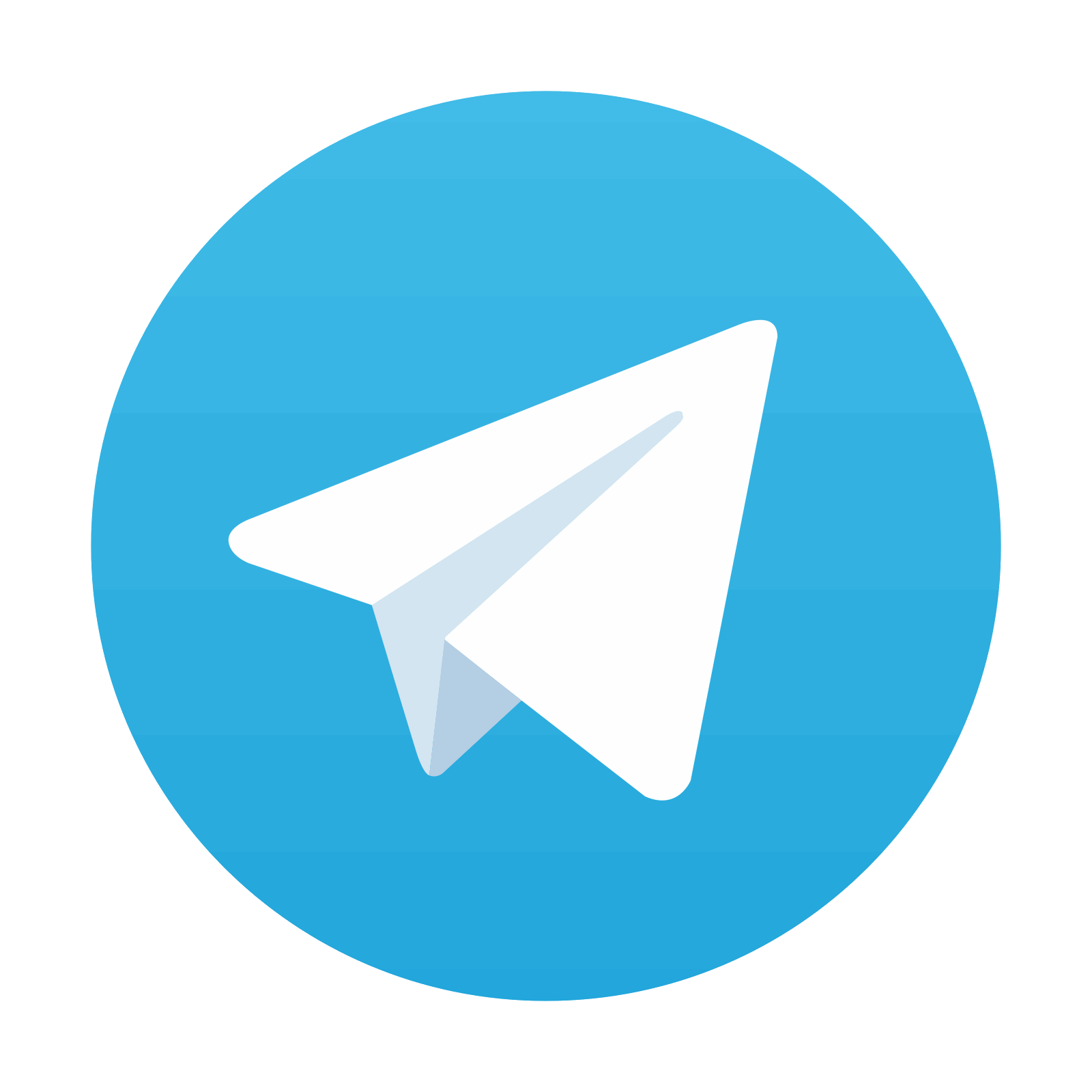
Stay updated, free articles. Join our Telegram channel

Full access? Get Clinical Tree
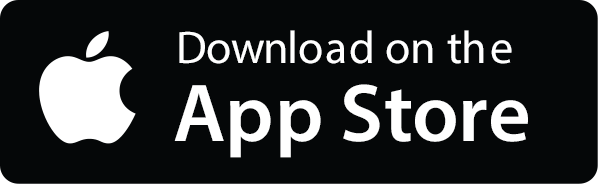
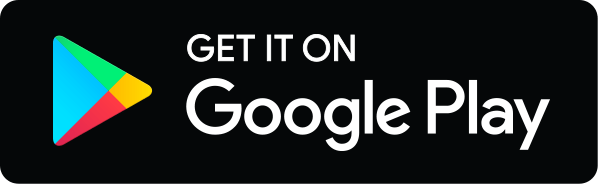
