An increasing number of pulmonary disorders are amenable to flexible or rigid bronchoscopic diagnostic or therapeutic intervention. New techniques such as endobronchial ultrasound, electromagnetic navigational bronchoscopy, and treatment of central airway obstruction, including tumor debulking and placement of airway stents, require specialized equipment and the physical space to store and use it. The increase in variety and volume of procedures led to the development of dedicated procedural space in many hospitals—interventional pulmonology or bronchoscopy suites.
Many bronchoscopic procedures can be performed with minimal sedation. Thus the continual presence of a trained anesthesia provider is not always necessary. However, because of procedural or patient complexity, anesthesia providers are being increasingly consulted to provide care in these settings. For several reasons, the pulmonology suite can be an intimidating location for the anesthesiologist or nurse anesthetist. Familiarity with the physical space and ancillary staff may be limited as a result of the intermittent request for anesthesia services. Patients often have multiple baseline comorbidities with tenuous respiratory or cardiac status. Procedures are typically short, with rapid patient turnover and limited time for preprocedural evaluation. The airway must be shared with the bronchoscopist, and the patient’s head is often turned away from the anesthesiologist. Anesthesia providers who do not frequently perform in these cases may be somewhat unfamiliar with the specialized ventilatory and intravenous anesthetic techniques used for these procedures. Finally, the procedure suite may be located distant from the main operating rooms, limiting the availability of assistance in an emergency.
The goal of this chapter is to provide an overview of the procedures and accompanying airway and ventilation choices, anesthetic management issues, and complications unique to the adult interventional bronchoscopy suite.
Interventional Bronchoscopic Techniques
Modern interventional bronchoscopy encompasses a variety of diagnostic and therapeutic procedures. An in-depth discussion of all techniques exceeds the scope of this chapter; therefore we have chosen to briefly examine a few important procedures that are either becoming widespread or show great potential for transforming interventional bronchoscopic practice over the next decade. Common anesthetic techniques and airway choices for each procedure are detailed in Table 16-1 .
Bronchoscopic Technique | Recommended Anesthetic Technique | Recommended Airway |
---|---|---|
DIAGNOSTIC | ||
Endobronchial ultrasound with transbronchial needle aspiration (EBUS-TBNA) | Conscious sedation versus less commonly general anesthesia | None versus SGA |
Electromagnetic navigational bronchoscopy (ENB) | Conscious sedation versus less commonly general anesthesia | None versus SGA |
THERAPEUTIC | ||
Treatment of central airway obstruction | TIVA with rigid bronchoscopy versus awake fiberoptic intubation; reduced Fi o 2 ; consider Heliox and maintenance of spontaneous ventilation even with rigid bronchoscopy | ETT versus rigid bronchoscopy |
Airway stents | General anesthesia, often TIVA with rigid bronchoscopy | ETT versus rigid bronchoscopy |
Bronchoscopic balloon dilation | TIVA with paralysis; jet ventilation versus bag-mask ventilation between balloon inflations | Rigid bronchoscopy |
Bronchoscopic lung volume reduction (BLVR) | Conscious sedation versus general anesthesia | None versus SGA |
Radiofrequency ablation | Conscious sedation versus general anesthesia if lung isolation is necessary; reduced Fi o 2 | None versus double-lumen endotracheal tube if lung isolation is necessary |
Bronchial thermoplasty | Conscious sedation | None versus SGA |
Endobronchial Ultrasound with Transbronchial Needle Aspiration
Endobronchial ultrasound with transbronchial needle aspiration (EBUS-TBNA) is a less invasive procedure than traditional mediastinoscopy; both are intended to yield tissue for diagnostic purposes. For EBUS, an ultrasound transducer integrated into a dedicated bronchoscope is placed either directly in contact with the tracheal or bronchial wall or indirectly via a saline-inflated balloon, displaying real-time ultrasound images. Fine needle aspiration of lymph nodes can then be performed under ultrasound visualization. Procedural indications include staging of non–small cell lung cancer, diagnosis of suspected lung cancer without endobronchial lesions, and evaluation of unexplained mediastinal lymphadenopathy. EBUS-TBNA may be diagnostically superior to computed tomography (CT) and positron emission tomography (PET) and has overall accuracy similar to that of mediastinoscopy but with less invasiveness and the ability to sample hilar lymph nodes. As the required technology is maturing and becoming more widely available, EBUS-TBNA is quickly becoming the standard of care.
EBUS with or without biopsies is usually only minimally stimulating, and most procedures can be completed under conscious sedation; however, general anesthesia may be requested in several instances. First, sampling adequacy is improved with sampling a higher number of lymph node stations. When extensive staging is performed, particularly when lymph nodes are less than 1 cm in their largest dimension, procedures can become quite prolonged. Patient intolerance may necessitate general anesthesia. Further, some pulmonologists prefer general anesthesia when a large number of stations are sampled, believing it improves diagnostic yield. Finally, the saline-filled balloon can occasionally completely obstruct the airway; cases have been reported in which coughing against the obstruction led to negative-pressure pulmonary edema, a problem obviated by general anesthesia with muscle relaxation.
Electromagnetic Navigational Bronchoscopy
Electromagnetic navigational bronchoscopy (ENB) was developed as a guidance system to assist the bronchoscopist in performing a biopsy of difficult-to-access peripheral, pulmonary nodules. The technology combines multidirectional CT (MDCT) imaging, magnetic field localization, and a steerable probe with a magnetized tip. During the procedure, the bronchoscope is guided into the area of interest and the tip location is displayed on the MDCT image. Diagnostic yield of biopsies for small peripheral lung nodules is much improved over previous techniques. Other potential uses include placement of fiducial markers for stereotactic radiotherapy and marking of nodules for surgical wedge resection. Sedation and anesthetic needs are similar to those of EBUS and dictated by patient comorbidities, needs of the bronchoscopist, and anticipated procedure duration.
Treatment of Central Airway Obstruction
In modern practice, relief of obstruction of the central airways (trachea, mainstem bronchi, and bronchus intermedius) remains the main indication for therapeutic bronchoscopy. Obstruction can result from intraluminal malignancy, external compression by mediastinal mass, massive hemoptysis with clot, or benign causes such as foreign body, postintubation tracheal stenosis, or tracheobronchomalacia. A variety of therapeutic approaches exist, with selection depending on cause, urgency, and availability. Laser ablation, electrocautery, argon plasma coagulation (APC), mechanical debridement, and airway stents can provide immediate relief of obstruction. In contrast, cryotherapy, brachytherapy, and photodynamic therapy produce delayed effects. If intervention is urgent because of severe or progressive obstruction, effort should be made to stabilize the patient to enable completion of diagnostic studies (such as CT imaging) to aid in developing an optimal treatment strategy. In this situation, heliox, given its lower density than air or 100% oxygen, can improve gas flow across highly narrowed areas of the airway and reduce turbulent flow conditions. If heliox is ineffective or not available, consideration must be given for intubation distal to the obstruction, typically accomplished with fiberoptic bronchoscopy. Alternatively, rigid bronchoscopy in expert hands can be lifesaving.
The neodymium-doped yttrium aluminum garnet (Nd:YAG) laser and other “hot” therapies, such as electrocautery and APC, are designed to vaporize tissue and achieve superior coagulation and thus are often chosen to treat obstructing central airway lesions. Unfortunately, these thermal techniques also carry an increased risk for airway and procedure room fires. Microdebriders, which mechanically debulk airway tumors, were developed as “cold” therapies that still can rapidly open an airway. Although inferior to thermal techniques in controlling bleeding, microdebriders also avoid some of their potential complications.
APC is based on gas flow, and many lasers have a gas-cooled fiber that is used to apply the energy. Case reports have documented intravascular gas during use of the Nd:YAG laser and even cardiac arrest secondary to gas embolism during bronchoscopic APC. In animal studies, gas emboli were more frequent with higher laser coolant flow and longer laser pulse generation, a finding the authors speculatively ascribed to positive-pressure ventilation forcing air through small bronchovascular defects. Therefore current procedural recommendations include using the lowest possible setting for laser coolant flow, using noncontact mode, and applying thermal ablation only during apnea or spontaneous breathing.
Airway Stents
Indications for airway stenting include extrinsic airway compression, mixed extrinsic and endoluminal lesions, recurrence of airway compromise after treatment of intraluminal lesions, inoperable nonmalignant disease, and treatment of airway fistulas. Stents can be made of silicone, expandable metal, or a combination of both, such as a covered metal stent. Metal stents resist migration because they become embedded in airway tissue and tend to generate a proliferative granulation response. Unfortunately, this also means that metal stents can be difficult and potentially dangerous to remove if in place for more than a few weeks. Expandable metal stents can be placed via flexible or rigid bronchoscope. Fluoroscopy is often used to guide placement. Because silicone stents do not collapse, a rigid bronchoscope must be used for placement.
Complications are common with all stent types. Granulation tissue formed after metal stent placement may occasionally cause airway obstruction and necessitate reintervention, often by Nd:YAG laser ablation. Removal of metal stents is best accomplished with a rigid bronchoscope and jet ventilation. Silicone stents are much easier to remove but are prone to migration, mucous obstruction, and infection.
Bronchoscopic Balloon Dilatation
Bronchoscopic balloon dilatation is commonly used to treat nontraumatic subglottic stenosis, often in conjunction with injection of corticosteroids or the anti-neoplastic drug mitomycin-C in an attempt to reduce the rate of restenosis. Total intravenous anesthesia (TIVA) with paralysis is often used in combination with intermittent bag-mask ventilation or jet ventilation before and after balloon dilatation, which typically lasts 1 to 3 minutes and can be performed repeatedly during a single procedure. During balloon dilatation, it is important to maintain adequate muscle relaxation and monitor for diaphragmatic firing to prevent respiratory effort against the occluded airway and the subsequent development of negative-pressure pulmonary edema. Further, improvement in stenosis is often delayed, so the anesthesia provider must be particularly vigilant in monitoring for airway obstruction and adequate ventilation both immediately after the procedure and in the postanesthesia care unit (PACU).
Radiofrequency Ablation
Radiofrequency ablation (RFA) is a minimally invasive treatment modality that has previously been used to treat patients with hepatic, renal, and breast tumors. After the RFA electrode is inserted into the target lesion, alternating radiofrequency currents are generated, leading to frictional heating and ultimately coagulative necrosis and cell death. The procedure tends to be well tolerated and is often done under conscious sedation. However, achieving effective targeting of pulmonary neoplastic lesions and protecting nontargeted tissues can be challenging. Deflating the target lung can be helpful but may move the lesion too close to central structures. A technique to obtain improved tumor positioning has been described using lung isolation with a double-lumen endotracheal tube (DLT) with static inflation of the target lung by either clamping the DLT lumen supplying the target lung or applying CPAP to maintain target lung inflation. Although not reported with RFA of pulmonary lesions, the use of high oxygen concentrations may pose a fire risk.
Bronchial Thermoplasty
In poorly controlled chronic asthma, airway smooth muscle hypertrophy is a component of airway remodeling, which contributes to ongoing symptoms. Bronchial thermoplasty, which uses heat generated by radiofrequency energy to destroy bronchial smooth muscle, has been shown in at least two trials to (1) improve prebronchodilator forced expiratory volume in 1 second (FEV 1 ), (2) reduce severe exacerbations, (3) decrease rescue medication use, and (4) improve reported quality of life for patients with severe asthma. Current guidelines, however, limit bronchial thermoplasty as a treatment option to selected patients with severe persistent asthma already on maximal medical therapy. Bronchial thermoplasty is generally well tolerated and can usually be accomplished under conscious sedation. However, if the anesthesiologist is involved, he or she must be aware of the increased risk for respiratory complications in the first 24 hours after the procedure, primarily acute bronchospasm or increased mucous plugging, occasionally resulting in lobar collapse.
Bronchoscopic Lung Volume Reduction
The National Emphysema Treatment Trial was published in 2003, demonstrating that in a carefully selected patient population with severe heterogeneous emphysema, lung volume reduction surgery (LVRS) reduced mortality, improved exercise tolerance, and improved quality of life. However, LVRS was associated with considerable morbidity and mortality, causing investigators to speculate that less invasive bronchoscopic interventions might achieve similar benefits while minimizing perioperative risk. Multiple techniques are currently under study, including intrabronchial valves, lung volume reduction coils, lung sealant, and bronchoscopic thermal vapor ablation. The goal for any of these procedures is to achieve segmental or lobar collapse to effectively reduce lung volume, thus reducing air trapping and improving elastic recoil and expiratory flows. Although results for many of these modalities are somewhat promising, none are ready for widespread adoption, and optimal anesthetic techniques have not yet been described.
Preoperative Evaluation
History and Review of Systems
The majority of patients presenting for interventional bronchoscopic procedures are classified as American Society of Anesthesiologists physical status 3 or higher. Tobacco abuse is a common risk factor for the majority of upper airway and pulmonary pathological conditions necessitating bronchoscopic intervention. Unsurprisingly, smoking-related diseases such as obstructive or less commonly restrictive pulmonary disease, coronary artery disease, congestive heart failure, peripheral vascular disease, cerebral ischemic disease, hypertension, malnutrition, and malignancies outside the lungs and airway are extremely common in this population. Heavy alcohol use, type 2 diabetes mellitus, and obesity are also common. Sequelae of the aforementioned pathological conditions that can affect anesthetic management include chronic kidney disease, obstructive sleep apnea, gastroparesis and gastroesophageal reflux disease, and limited neck mobility. The review of systems should further focus on the patient’s functional status, symptom control of baseline pulmonary or cardiac disease and any environmental triggers, presence of orthopnea, specific triggers for cough, and the severity of reflux disease.
Rigid bronchoscopy requires neck hyperextension, so patients presenting for this procedure should be assessed for risk factors for cervical spine subluxation such as trisomy 21 (Down syndrome) cervical ankylosis, or rheumatoid or other inflammatory arthritis with cervical involvement. Inquiring about previous chemotherapy is important, particularly the use of bleomycin and its associated risks for pulmonary fibrosis and acute oxygen toxicity, as well as doxorubicin and its potential for causing cardiomyopathy. Patients with previous lung transplantation or cystic fibrosis require increased sedative dosing during flexible bronchoscopy. Interestingly, advanced age alone does not confer an increased risk for complications from either flexible or rigid bronchoscopy.
Preprocedure Studies
Patients with suspected chronic obstructive pulmonary disease (COPD) should undergo spirometry and also should have a baseline arterial blood gas sample drawn if COPD is determined to be severe (FEV 1 <40% predicted and/or room air oxygen saturation [Sa o 2 ] <93%). If biopsies are to be performed, coagulation tests, including international normalized ratio, partial thromboplastin time, and platelet count, may be considered. Other laboratory studies such as a complete blood count or metabolic panel should not be routinely ordered unless prompted by the history and review of systems. Radiographic images must be reviewed, particularly when central airway obstruction is present or suspected, allowing the anesthesiologist to assess the potential for clinical airway compromise. Large bullae typical of severe emphysema suggest an increased risk for pneumothorax or other barotrauma with jet ventilation or even conventional positive-pressure ventilation.
Preoperative Evaluation
History and Review of Systems
The majority of patients presenting for interventional bronchoscopic procedures are classified as American Society of Anesthesiologists physical status 3 or higher. Tobacco abuse is a common risk factor for the majority of upper airway and pulmonary pathological conditions necessitating bronchoscopic intervention. Unsurprisingly, smoking-related diseases such as obstructive or less commonly restrictive pulmonary disease, coronary artery disease, congestive heart failure, peripheral vascular disease, cerebral ischemic disease, hypertension, malnutrition, and malignancies outside the lungs and airway are extremely common in this population. Heavy alcohol use, type 2 diabetes mellitus, and obesity are also common. Sequelae of the aforementioned pathological conditions that can affect anesthetic management include chronic kidney disease, obstructive sleep apnea, gastroparesis and gastroesophageal reflux disease, and limited neck mobility. The review of systems should further focus on the patient’s functional status, symptom control of baseline pulmonary or cardiac disease and any environmental triggers, presence of orthopnea, specific triggers for cough, and the severity of reflux disease.
Rigid bronchoscopy requires neck hyperextension, so patients presenting for this procedure should be assessed for risk factors for cervical spine subluxation such as trisomy 21 (Down syndrome) cervical ankylosis, or rheumatoid or other inflammatory arthritis with cervical involvement. Inquiring about previous chemotherapy is important, particularly the use of bleomycin and its associated risks for pulmonary fibrosis and acute oxygen toxicity, as well as doxorubicin and its potential for causing cardiomyopathy. Patients with previous lung transplantation or cystic fibrosis require increased sedative dosing during flexible bronchoscopy. Interestingly, advanced age alone does not confer an increased risk for complications from either flexible or rigid bronchoscopy.
Preprocedure Studies
Patients with suspected chronic obstructive pulmonary disease (COPD) should undergo spirometry and also should have a baseline arterial blood gas sample drawn if COPD is determined to be severe (FEV 1 <40% predicted and/or room air oxygen saturation [Sa o 2 ] <93%). If biopsies are to be performed, coagulation tests, including international normalized ratio, partial thromboplastin time, and platelet count, may be considered. Other laboratory studies such as a complete blood count or metabolic panel should not be routinely ordered unless prompted by the history and review of systems. Radiographic images must be reviewed, particularly when central airway obstruction is present or suspected, allowing the anesthesiologist to assess the potential for clinical airway compromise. Large bullae typical of severe emphysema suggest an increased risk for pneumothorax or other barotrauma with jet ventilation or even conventional positive-pressure ventilation.
Management of Anesthesia: Pharmacology
Premedication
Anticholinergics
In a consensus statement on the use of sedation and topical anesthesia during flexible bronchoscopy, the American College of Chest Physicians advised against the routine use of anticholinergic medication as a bronchodilator or antisialagogue. However, glycopyrrolate 0.005 mg/kg (approximately 0.2 to 0.4 mg for an average-size adult) is not unreasonable in selected cases, such as when aerosolized local anesthetic is to be used, and is generally better tolerated and more effective than atropine in decreasing secretions.
Sedatives
Oral clonidine, at a dose of 150 mcg or up to 3 mcg/kg, has been suggested as a premedicant because it has some sedative effect and can blunt the hemodynamic response to bronchoscopy or laryngoscopy, though additional sedative medicines are likely to be needed during awake fiberoptic bronchoscopy. Other preprocedure sedatives and anxiolytics such as midazolam (0.25 to 2 mg intravenously) or diazepam (2.5 to 10 mg intravenously) should be minimized because of the risk for respiratory depression in an undermonitored patient. Oversedation with benzodiazepines also can lead to disinhibition, complicating a bronchoscopic procedure that could otherwise be performed in a lightly sedated and cooperative patient. Supplemental oxygen should be routinely provided before administering any sedatives, and anxiolytics should be used in only extremely anxious patients.
Steroids
Multiple studies have suggested that a 24-hour course of dexamethasone or other corticosteroid can reduce laryngeal edema and decrease the risk for postextubation stridor or obstruction in a critical care setting, but there is no solid evidence that the routine use of corticosteroids has any benefit in most bronchoscopic procedures. Nonetheless, many practitioners administer dexamethasone 0.2 to 0.5 mg/kg (up to 10 mg) intravenously to prevent airway swelling in cases of longer duration or when extensive inflammation of vocal cords or airway mucosa is expected. This may not be an unreasonable approach, because at least two small studies have suggested that a prophylactic dose of dexamethasone 0.2 mg/kg can at least decrease the incidence of postoperative sore throat and hoarseness in patients intubated for relatively short procedures. Although high-dose steroids have many potential adverse effects, the risk for significant complications after a single prophylactic dose of dexamethasone is likely negligible.
Antiemetics
Published guidelines on postoperative nausea and vomiting (PONV) prophylaxis do not recommend routine antiemetic administration. However, the risk for aspiration during and after bronchoscopic interventions may be higher than that for other procedures. Given that many of the patients undergoing such procedures have limited pulmonary reserve, aggressive PONV prophylaxis may be warranted. Because multiple drug classes have similar efficacy, medication selection is based on side effect profile, patient factors, physician preference, availability, and cost. Dexamethasone given preprocedurally is an effective antiemetic and is often given for other reasons, as discussed earlier.
Antacids and Gastrokinetics
Reducing gastric acidity may decrease the risk for chemical pneumonitis in patients who aspirate, although no evidence supports the routine use of acid-reducing drugs in patients undergoing bronchoscopic procedures. Although protein pump inhibitors are the most potent class, they may take more than 24 hours for full effect and thus have a limited role as preoperative medications. In contrast, histamine-2 (H 2 ) antagonists may produce a clinically significant increase in gastric pH and reduction in gastric volume 30 to 60 minutes after an intravenous dose or 2 to 3 hours after an oral dose. Metoclopramide is often used as a prokinetic in patients with gastroparesis. Less effective in increasing gastric pH than H 2 blockers, metoclopramide also has more potential side effects and drug and disease interactions, making it a less attractive option. In patients with high aspiration risk presenting without sufficient time for other medications to take effect, a nonparticulate antacid such as 0.3 M sodium citrate can be administered with a resultant immediate increase in gastric pH.
Bronchodilators
Many authorities recommend that patients with asthma or COPD with a reversible component routinely receive a short-acting bronchodilator preprocedurally, although such premedication may not produce any benefit in COPD. Atropine has been long suggested to have protective bronchodilatory properties when given before bronchoscopic procedures. However, more recent studies failed to show meaningful improvement in lung function or sustained benefit postprocedurally.
Local Anesthetics and Airway Reflex Suppression
Successful bronchoscopy in a patient not receiving general anesthesia requires adequate suppression of airway reflexes to facilitate the procedure. The degree and location of necessary reflex suppression depend on the planned airway management and route of bronchoscopy. Perineural injections, topicalization with local anesthetics, or a combination of these techniques may be used. In either case, lidocaine is the preferred local anesthetic because of its rapid onset, appropriately short duration of action, and relative safety. The total cumulative dose of lidocaine used through all procedures described in the following section should absolutely not exceed 8.2 mg/kg ; most practitioners are more conservative and limit maximum lidocaine administration to 5 mg/kg.
Nasal Anesthesia
Adequate nasal anesthesia usually can be achieved using a combination of a vasoconstricting nasal spray, such as oxymetazoline or phenylephrine, followed by application of viscous lidocaine jelly. This process usually provides adequate sensory blockade and lubrication to allow passage of a nasal trumpet, bronchoscope, or endotracheal tube (ETT). Alternatively, the pterygopalatine ganglion (providing sensory input to the anterior third of the nasal septum and posterior nasal cavity) can be blocked by soaking a cotton ball or cotton-tipped applicator in 2% to 4% lidocaine with epinephrine (1:200,000) or phenylephrine (1:20,000), passing it slowly to the posterior wall of the nasopharynx, and leaving it in place for 5 to 10 minutes.
Anesthesia of the Posterior Oropharynx and Upper Airway
For an awake patient to tolerate either nasotracheal or orotracheal bronchoscopy or intubation, sequential blockade of the superior laryngeal nerve and recurrent laryngeal nerve is required, via either perineural injections or less invasive mucosal saturation. Direct regional blockade of the superior laryngeal nerve is performed bilaterally at the level of the thyrohyoid membrane, just inferior to the cornu of the hyoid bone. A small-bore needle is directed anteroinferomedially until the greater cornu is contacted; the needle is then withdrawn slightly, and after negative aspiration is confirmed, 2 mL of 2% to 4% lidocaine with epinephrine 1:200,000 is injected. The block is repeated in the same fashion on the contralateral side. Recurrent laryngeal nerve blockade is necessary to suppress coughing when the bronchoscope is passed through the vocal cords and into the trachea and can be readily achieved by translaryngeal block. A 20- or 22-gauge needle on a syringe under constant negative pressure is advanced through the cricothyroid membrane until air is aspirated, and then 4 mL of 2% to 4% lidocaine with epinephrine is injected. Patients invariably cough with the delivery of the local anesthetic solution, which helps disperse the local anesthetic but increases the risk for puncturing the back wall of the trachea with the needle. To minimize this risk, some practitioners prefer to use a 20- or 22-gauge peripheral intravenous catheter-over-needle to access the trachea. After aspiration of air, the needle is removed, air aspiration is repeated to confirm catheter location in the airway, and the local anesthetic solution is delivered. Ultrasound guidance has recently been used to facilitate this procedure. Remember that the induction of coughing potentially could be life-threatening in patients with severe central airway obstruction, and another approach to airway management may be required.
Although direct regional blockade of the superior and recurrent laryngeal nerves is possible, adequate anesthesia usually can be achieved less invasively with topical local anesthetic application and mucosal saturation. After administration of a drying agent such as glycopyrrolate to reduce oropharyngeal secretions and improve local anesthetic absorption, 5 mL of 4% lidocaine or another local anesthetic can be aerosolized via a standard nebulizer attached to a face mask or mouthpiece and is well tolerated in nearly all patients. With enough time, aerosolized local anesthetic usually provides adequate initial anesthesia of the oropharynx and upper airway. Denser anesthesia of more distal structures can then be achieved through a combination of gargled, swallowed, or sprayed liquid or viscous local anesthetic. Benzocaine spray can be used, but excessive administration can cause methemoglobinemia. Finally, the working channel of a flexible bronchoscope can be used to sequentially inject 1 to 2 mL of 2% to 4% lidocaine over the epiglottis, just above the vocal cords, just below the cords, and at the carina. Systemic lidocaine levels are generally negligible even when administered to mucosa using a combination of methods.
Moderate Sedation
Standard flexible bronchoscopy in an otherwise healthy patient usually requires little more than moderate conscious sedation with adequate airway reflex suppression via any of the previously described techniques. Intravenous fentanyl 2 to 2.5 mcg/kg titrated over the duration of the procedure in 12.5- to 50-mcg aliquots and midazolam 0.5 to 2 mg usually provide adequate sedation, anxiolysis, and some degree of cough suppression. Higher doses of benzodiazepines tend to cause disinhibition and may actually lead to increased coughing. Between 500 to 1000 mcg of alfentanil was used more commonly in the past as an alternative to fentanyl to achieve adequate sedation for flexible bronchoscopy. Adding midazolam to alfentanil may increase the risk for hypoxia without improving sedation or decreasing discomfort.
When anesthesiologists are consulted, the most common choice for moderate sedation is propofol, administered either by intermittent boluses (10 to 20 mg) or as a low-dose infusion (10 to 50 mcg/kg/min). In contrast to the combination of midazolam and fentanyl, propofol offers more rapid recovery and easier titration in bronchoscopic cases lasting more than 20 minutes.
Remifentanil by infusion can be used as a sole agent for moderate sedation or as an infusion after 0.5 to 2 mg of midazolam for particularly anxious patients. The initial infusion rate should be 0.1 mcg/kg/min started 5 minutes before beginning bronchoscopy, with the infusion then weaned to approximately 0.05 mcg/kg/min as tolerated during the procedure. This regimen usually provides adequate sedation and cough suppression for bronchoscopy and has even been used for more stimulating procedures, such as tracheoplasty using combined laser and balloon dilation, although providers may find that patients need to be “coached” to breathe. Remifentanil offers an advantage as a sole agent over other opioids because patients predictably awaken within 4 to 5 minutes of discontinuation of its infusion, regardless of infusion duration.
Dexmedetomidine has been studied as a sedative for bronchoscopic procedures but has the disadvantage of requiring a loading infusion, typically 0.5 to 1 mcg/kg over 10 to 20 minutes, before the initiation of a maintenance infusion of 0.2 to 0.7 mcg/kg/hr. Given the relatively short duration and fast turnover of most procedures performed in a bronchoscopy suite, this relatively long loading time may result in significant inconvenience and cost ineffectiveness. Nonetheless, the use of dexmedetomidine is appealing because of its lack of blunting of respiratory drive while providing analgesia. Dexmedetomidine can be associated with clinically significant bradycardia and hypotension at any point during its use, as well as occasional hypertension during the initial load.
General Anesthesia
Induction
General anesthesia may be necessary for long cases, procedures in which immobility is necessary, or when significant stimulation is anticipated. Induction of general anesthesia should be relatively rapid and without coughing while maintaining hemodynamic stability.
Inhaled sevoflurane can be used to gently induce general anesthesia and may be the preferred choice of induction in cases of critical airway stenosis because spontaneous ventilation is maintained. A single-breath vital capacity technique in which the patient, beginning from maximal exhalation, deeply inhales 8% sevoflurane and then holds the breath is reported to be most effective in healthy volunteers. Although a single-breath induction has been successfully reported in patients with airway stenosis, patients with pulmonary disease tend to do better with a tidal volume technique in which the concentration of sevoflurane is slowly titrated up, with the patient taking regular normal tidal volume breaths, because many cannot take large breaths and hold them without coughing.
As in general operating rooms, propofol at 1 to 2 mg/kg is the most commonly used primary induction medication in the bronchoscopy suite. Ketamine is an attractive induction agent because of its unique characteristics; as an indirect sympathomimetic, it is a potent bronchodilator with relative preservation of respiratory drive and cardiac function. At an induction dose of 1 to 2 mg/kg, psychotropic side effects are rarely encountered after benzodiazepine premedication (e.g., midazolam 1 to 2 mg). Etomidate 0.2 to 0.3 mg/kg is an alternative induction agent for patients with impaired left ventricular function. Complete opioid-based or benzodiazepine-based induction regimens are rarely appropriate for bronchoscopic procedures because the doses required to achieve adequate anesthetic depth do not allow emergence in a timely manner.
Maintenance
Either TIVA or inhalational anesthetic can be employed for maintenance of general anesthesia, although TIVA is often preferred for bronchoscopic procedures because of its multiple advantages. Most notably, recurrent insertion and removal of the bronchoscope, as well as frequent airway suctioning, cause inconsistent delivery of volatile agents. TIVA ensures uninterrupted delivery of anesthetic separate from ventilation and avoids exposing procedural room personnel to leaked inhalational agent. Other advantages of TIVA when propofol-based include less PONV, decreased cough at emergence, and less depression of bronchial mucous transport velocity. Inhalational anesthetics may predispose patients to increased bleeding at the site of needle puncture during biopsies, perhaps because volatile agents cause local vasodilation in the bronchial mucosa. Finally, jet ventilation and other specialized modes of ventilation may be technically incompatible with the delivery of volatile anesthetic.
Propofol can be used as a sole agent for maintenance of anesthesia for flexible bronchoscopy at a dose of 75 to 250 mcg/kg/min, but it is usually necessary to add an opioid to suppress coughing in nonparalyzed patients or to blunt the hemodynamic response to more stimulating procedures, such as rigid bronchoscopy. Either intermittent low-dose fentanyl (12.5- to 50-mcg aliquots to a total of <2 to 2.5 mcg/kg for the duration of a typical case) or a remifentanil infusion (0.1 to 0.3 mcg/kg/min) are commonly used. A higher dose of remifentanil (0.5 mcg/kg/min) together with propofol more effectively attenuates the hemodynamic response to insertion of a rigid bronchoscope, but more frequently causes hypotension. Remifentanil has a reliably short duration of action, with 3 to 10 minutes to arousal after its discontinuation regardless of the duration or dose of infusion, allowing predictable emergence. Arousal from propofol is short and fairly predictable after short cases typical of bronchoscopic procedures. Prolonged use, however, may lead to unpredictably prolonged emergence. If a target-controlled infusion, currently not available in the United States, is administered, appropriate induction and maintenance plasma concentration targets for propofol are 7 mcg/mL and less than 5 to 6 mcg/mL, respectively, if a remifentanil infusion or intermittent fentanyl boluses are used concurrently. The most commonly reported initial effect-site target concentration for remifentanil infusions in bronchoscopic procedures is 2 to 5 ng/mL.
Muscle Relaxation
It is worth remembering that most procedures performed in the bronchoscopy suite do not absolutely require pharmacological muscle relaxation if quiescent conditions can be achieved by other means. Even procedures requiring rigid bronchoscopy, jet ventilation, laser therapy, and stent placement have been safely performed without paralysis. Potential advantages of avoiding neuromuscular blockade include shortened emergence and no chance of residual paralysis or recurarization of patients with baseline or anticipated limited pulmonary reserve after the procedure. Despite this, many proceduralists and anesthesia providers prefer muscle relaxation for procedures with a high risk for airway injury or hemorrhage or the potential for spontaneous respiratory effort against an obstructed or occluded airway, which can lead to negative-pressure pulmonary edema.
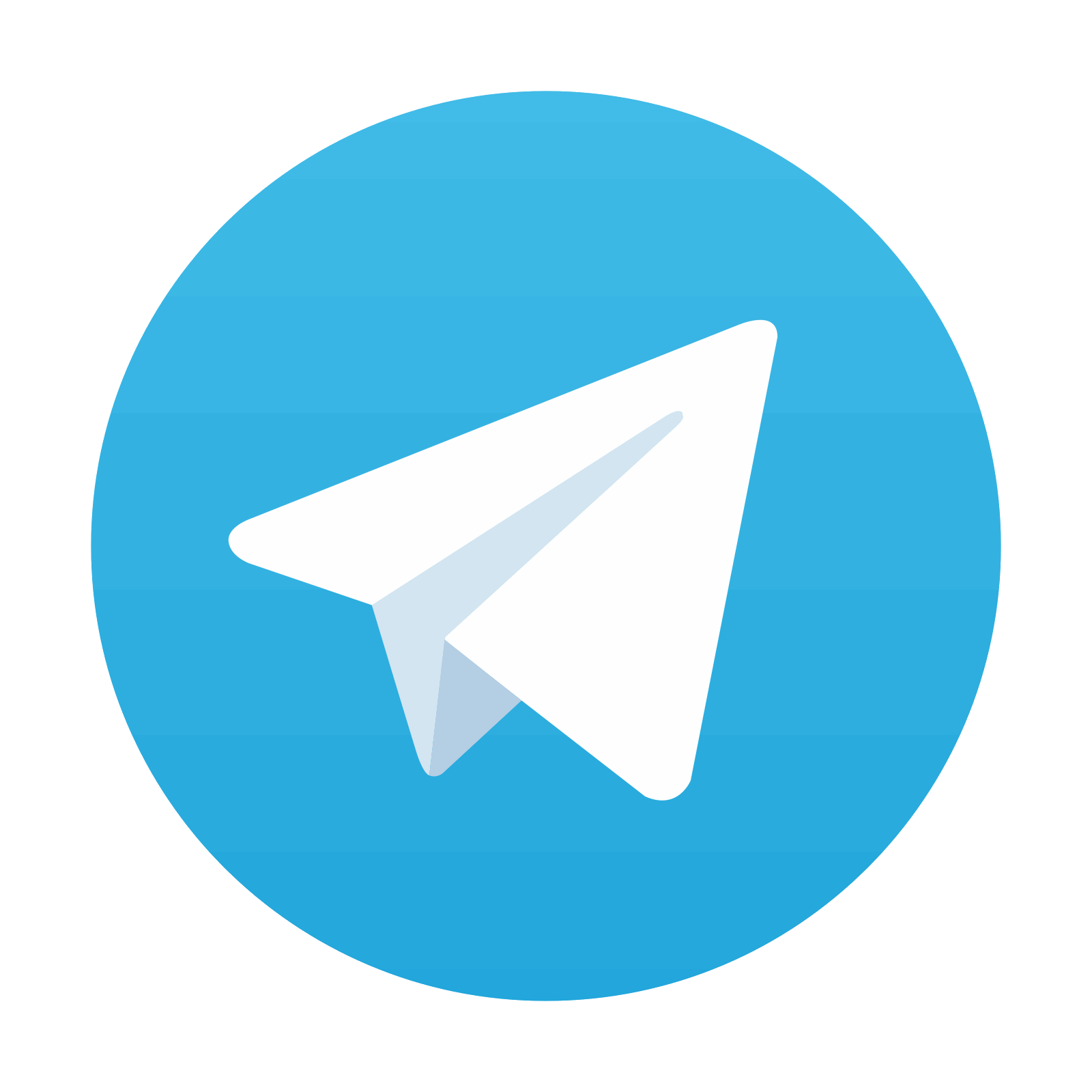
Stay updated, free articles. Join our Telegram channel

Full access? Get Clinical Tree
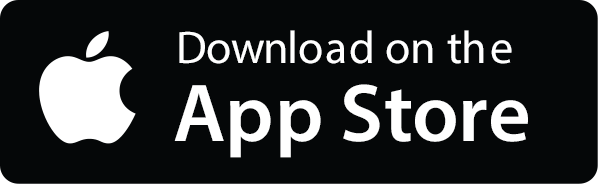
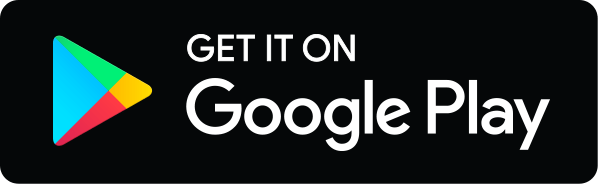