13 Anesthesia for Thoracic Surgery
General Perioperative Considerations
Inhalational anesthetic agents are commonly administered in 100% O2 during maintenance of anesthesia. Isoflurane may be preferred owing to less attenuation of hypoxic pulmonary vasoconstriction compared with other inhalational agents, although this has not been studied in children.1 Nitrous oxide is avoided. IV opioids have a sparing effect on the concentration of inhalational anesthetics required, and therefore may limit the impairment of hypoxic pulmonary vasoconstriction. Alternatively, total IV anesthesia may be used.
A variety of approaches have been described to prevent and treat pain after videoscopic procedures. Bupivacaine infiltration at incision sites before skin incision has been shown to decrease postoperative pain.2,3 Bupivacaine infiltration was found to be superior to IV fentanyl or tenoxicam for reducing postoperative pain.4 The combination of general anesthesia with regional anesthesia that also contributes postoperative analgesia, is particularly desirable for thoracotomy, but may also be beneficial for thoracoscopic procedures. This is especially true when thoracostomy tube drainage, a source of significant postoperative pain, is used after surgery. In addition, regional blockade for postoperative analgesia facilitates deep breathing and coughing, which may limit atelectasis and pneumonia. A variety of regional anesthetic techniques have been described for intraoperative anesthesia and postoperative analgesia, including intercostal and paravertebral blocks, intrapleural infusions, and epidural anesthesia (see Chapters 41, 42, and 43).
Ventilation and Perfusion during Thoracic Surgery
In adults with unilateral lung disease, oxygenation is optimal when the patient is placed in the lateral decubitus position with the healthy lung dependent (“down”) and the diseased lung nondependent (“up”).5 Presumably, this is related to an increase in blood flow to the dependent, healthy lung and a decrease in blood flow to the nondependent, diseased lung because of the hydrostatic pressure (or gravitational) gradient between the two lungs. This phenomenon promotes matching in the adult patient undergoing thoracic surgery in the lateral decubitus position.
In infants with unilateral lung disease, however, oxygenation is improved with the healthy lung “up.”6 Several factors account for this discrepancy between adults and infants. Infants have a soft, easily compressible rib cage that cannot fully support the underlying lung. Therefore functional residual capacity is closer to residual volume, making airway closure likely to occur in the dependent lung even during tidal breathing.7 When the adult is placed in the lateral decubitus position, the dependent diaphragm has a mechanical advantage because it is “loaded” by the abdominal hydrostatic pressure gradient. This pressure gradient is reduced in infants, thereby reducing the functional advantage of the dependent diaphragm. The infant’s small size also reduces the hydrostatic pressure gradient between the nondependent and dependent lungs. Consequently, the favorable increase in perfusion to the dependent, ventilated lung is attenuated in infants.
Finally, the infant’s increased oxygen requirement, coupled with a small functional residual capacity, predisposes to hypoxemia. Infants normally consume 6 to 8 mL of O2/kg/min compared with adult rates of 2 to 3 mL of O2/kg/min.8 For these reasons, infants are at an increased risk of significant hemoglobin desaturation during surgery in the lateral decubitus position.
A modest increase in Paco2 may be beneficial in children during thoracoscopic procedures. In a study of 12 children undergoing video-assisted thoracoscopic surgery for patent ductus arteriosus closure, hypercapnea targeting Paco2 values between 50 and 70 mm Hg increased cardiac output, central venous and arterial oxygen tension.9
Thoracoscopy
With the miniaturization of instruments, progress in video technology, and growing experience among pediatric surgeons, video endoscopic surgery of the chest, or thoracoscopy, is being performed for an increasing number of pediatric surgical indications (Table 13-1). Advantages of thoracoscopy include smaller chest incisions, reduced postoperative pain, and more rapid postoperative recovery compared with thoracotomy (Table 13-2, Fig. 13-1).10,11 Endoscopes that can be passed through a needle and trocar system are now manufactured, and digital video signals can be electronically modified to yield sharp, detailed, color images with a minimum light intensity. Digital cameras are designed to maintain an image in an upright orientation regardless of how the telescope is rotated. They are also equipped with an optical or digital zoom to magnify the image or give the illusion of moving the telescope closer to the object of interest. The smallest of telescopes use fiberoptics and are less than 2 mm in diameter (Fig. 13-2). Two-millimeter disposable ports, mounted on a Veress needle, are used for introduction of these small instruments. Larger instruments and ports are used in larger children and for more complex cases.
TABLE 13-1 Thoracoscopic Procedures in Infants and Children
TABLE 13-2 Advantages of Thoracoscopic versus Open-Chest Surgery
Techniques for Single-Lung Ventilation in Infants and Children
Use of a Single-Lumen Endotracheal Tube
The simplest means of providing SLV is to intentionally intubate the ipsilateral main-stem bronchus with a conventional single-lumen endotracheal tube (ETT).12 When the left bronchus is to be intubated, the bevel of the ETT is rotated 180 degrees and the child’s head is turned to the right.13 The ETT is advanced into the bronchus until breath sounds on the operative side disappear. A fiberoptic bronchoscope (FOB) may be passed through or alongside the ETT to confirm or guide placement. Alternatively, fluoroscopy may be used to guide and position the ETT.14 When a cuffed ETT is used, the distance from the proximal cuff to the tip of the ETT must be less than the length of the main-stem bronchus so that the upper lobe orifice is not occluded (Fig. 13-3).15 This technique is simple and requires no special equipment other than a FOB. This may be the preferred technique of SLV in emergency situations, such as airway hemorrhage or contralateral tension pneumothorax.
Variations of this technique have been described, including intubation of both bronchi independently with small ETTs.16–19 One main-stem bronchus is initially intubated with an ETT, after which another ETT is advanced over a FOB into the opposite bronchus. The disadvantages of these techniques include technical difficulties and trauma to the tracheal and bronchial mucosa. Even after successful bilateral bronchial intubation, the inner diameters of the tubes will be small, limiting gas flow and impeding suctioning of the airways.
Use of Balloon-Tipped Bronchial Blockers
A Fogarty embolectomy catheter or an end-hole, balloon wedge catheter may be used for bronchial blockade to provide SLV (Fig. 13-4).20–23 Placement of a Fogarty catheter is facilitated by bending the tip of its stylet toward the bronchus on the operative side. An FOB may be used to reposition the catheter and confirm appropriate placement. Various techniques for placing an end-hole catheter outside the ETT have been described. Using one such method, the bronchus on the operative side is initially intubated with an ETT.20 A guidewire is then advanced into that bronchus through the ETT. The ETT is removed and the blocker is advanced over the guidewire into the bronchus. An ETT is then reinserted into the trachea alongside the blocker catheter. The catheter balloon is positioned in the proximal main-stem bronchus under fiberoptic visual guidance. Alternatively, if an FOB small enough to pass through the indwelling ETT is not available, fluoroscopy may be used (Fig. 13-5). With an inflated blocker balloon the airway is completely sealed, providing more predictable lung collapse and better operating conditions than with an ETT in the bronchus.
One potential problem with this technique is dislodgement of the blocker balloon into the trachea, blocking ventilation to both lungs and/or preventing collapse of the operative lung. The balloons of most catheters currently used for bronchial blockade have low compliance properties (i.e., low volume, high pressure). They require 1 to 3 mL of air or saline to fully inflate. Overdistention of the balloon can damage or even rupture the airway.24 A recent study, however, reported that bronchial blocker cuffs produced lower “cuff-to-tracheal” pressures than double-lumen tubes.25 When closed-tip bronchial blockers are used, the operative lung cannot be suctioned and continuous positive airway pressure (CPAP) cannot be provided to the operative lung if needed.
When a bronchial blocker is placed outside the ETT, care must be taken to avoid injury caused by compression and resultant ischemia of the tracheal mucosa. The sum of the catheter diameter and the outer diameter of the ETT should not significantly exceed the tracheal diameter. Outer diameters for pediatric-size ETTs are shown in Table 13-3. These numbers provide an estimate of the predicted tracheal diameter, which should approximate the size of the uncuffed ETT predicted to produce a seal in the trachea.
TABLE 13-3 Single-Lumen Uncuffed Tracheal Tube Diameters
ID (mm)* | OD (mm) | Equivalent French Size† |
---|---|---|
3.0 | 4.3 | 13 |
3.5 | 4.9 | 15 |
4.0 | 5.5 | 17 |
4.5 | 6.2 | 19 |
5.0 | 6.8 | 21 |
5.5 | 7.5 | 23 |
6.0 | 8.2 | 25 |
6.5 | 8.9 | 27 |
7.0 | 9.6 | 29 |
7.5 | 10.2 | 31 |
8.0 | 10.8 | 32 |
Note: Cuffed tubes have approximately 0.5-mm additional outer diameter. The external diameter may also vary by manufacturer.
ID, Internal diameter; OD, outer diameter.
*Sheridan tracheal tubes (Hudson RCI, Arlington Heights, Ill.).
†French (F) size is 3 x OD (mm).
Recently, adapters have been developed that facilitate ventilation during placement of a bronchial blocker through an indwelling ETT.26,27 Use of a 5F endobronchial blocker that is designed for use in children, and has a multiport adapter and FOB, has been described (Cook Medical, Inc., Bloomington, Ind.).28 The balloon is elliptical so that it conforms to the bronchial lumen when inflated. The blocker catheter has a maximum outer diameter of 2.5 mm (including the deflated balloon), a central lumen with a diameter of 0.7 mm, and a distal balloon with a capacity of 3 mL. The balloon has a length of 1.0 cm, corresponding to the length of the right main-stem bronchus in children approximately 2 years of age.29 The blocker is placed coaxially through a dedicated port in the adapter, which also has a port for passage of a FOB and ports for connection to the anesthesia breathing circuit and ETT (Fig. 13-6). The FOB port has a plastic sealing cap, whereas the blocker port has a Tuohy-Borst connector that locks the catheter in place and maintains an airtight seal. Because oxygen can be administered during passage of the blocker and FOB, the risk of hypoxemia during blocker placement is diminished, and repositioning of the blocker may be performed with fiberoptic guidance during surgery.
When a FOB is used to guide the placement of a bronchial blocker, both the blocker catheter and FOB must pass through the indwelling ETT. The smallest ETT through which the catheter and FOB can be passed must be larger than the sum of the outer diameters of the catheter and the FOB. The 5F Cook bronchial blocker and an FOB with a 2.2-mm diameter, for example, may be inserted through an ETT with an internal diameter as small as 5.0 mm; for children with an indwelling ETT smaller than this, a blocker catheter can be positioned under fluoroscopy (see Fig. 13-5).30
Use of a Univent Tube
The Univent tube (Fuji Systems Corporation, Tokyo) is a conventional ETT with a second lumen containing a small blocker catheter that can be advanced into a bronchus (Fig. 13-7).31,32 A balloon located at the distal end of this small tube serves as a blocker. Univent tubes require a FOB for successful placement. Univent tubes are now available in sizes with internal diameters as small as 3.5 and 4.5 mm, for use in children older than 6 years of age.33 Because the blocker tube is firmly attached to the main ETT, displacement of the Univent blocker balloon is less likely than when other blocker techniques are used. The blocker tube has a small lumen that allows egress of gas and can be used to insufflate oxygen or suction the operated lung.
One disadvantage of the Univent tube is the large cross-sectional area occupied by the blocker channel, especially in the smaller size tubes. Therefore Univent tubes have a large outer diameter with respect to their inner (luminal) diameters (Table 13-4). Smaller Univent tubes have a disproportionately high resistance to gas flow.34 The Univent tube’s blocker balloon has low-volume, high-pressure characteristics, so mucosal injury can occur during normal inflation.35,36
TABLE 13-4 Univent Tube Diameters
ID (mm) | OD (mm)* |
---|---|
3.5 | 7.5/8.0 |
4.5 | 8.5/9.0 |
6.0 | 10.0/11.0 |
6.5 | 10.5/11.5 |
7.0 | 11.0/12.0 |
7.5 | 11.5/12.5 |
8.0 | 12.0/13.0 |
8.5 | 12.5/13.5 |
9.0 | 13.0-14.0 |
ID, Internal diameter; OD, outer diameter.
Use of Double-Lumen Tubes
All double-lumen tubes (DLTs) are essentially two tubes of unequal length molded together. The shorter tube ends in the trachea, and the longer tube ends in the bronchus (Fig. 13-8). DLTs for older children and adults have cuffs located on the tracheal and bronchial lumens. The tracheal cuff, when inflated, allows positive-pressure ventilation. The inflated bronchial cuff allows ventilation to be diverted to either or both lungs and protects each lung from contamination from secretions, purulent material, or blood originating from the contralateral side.
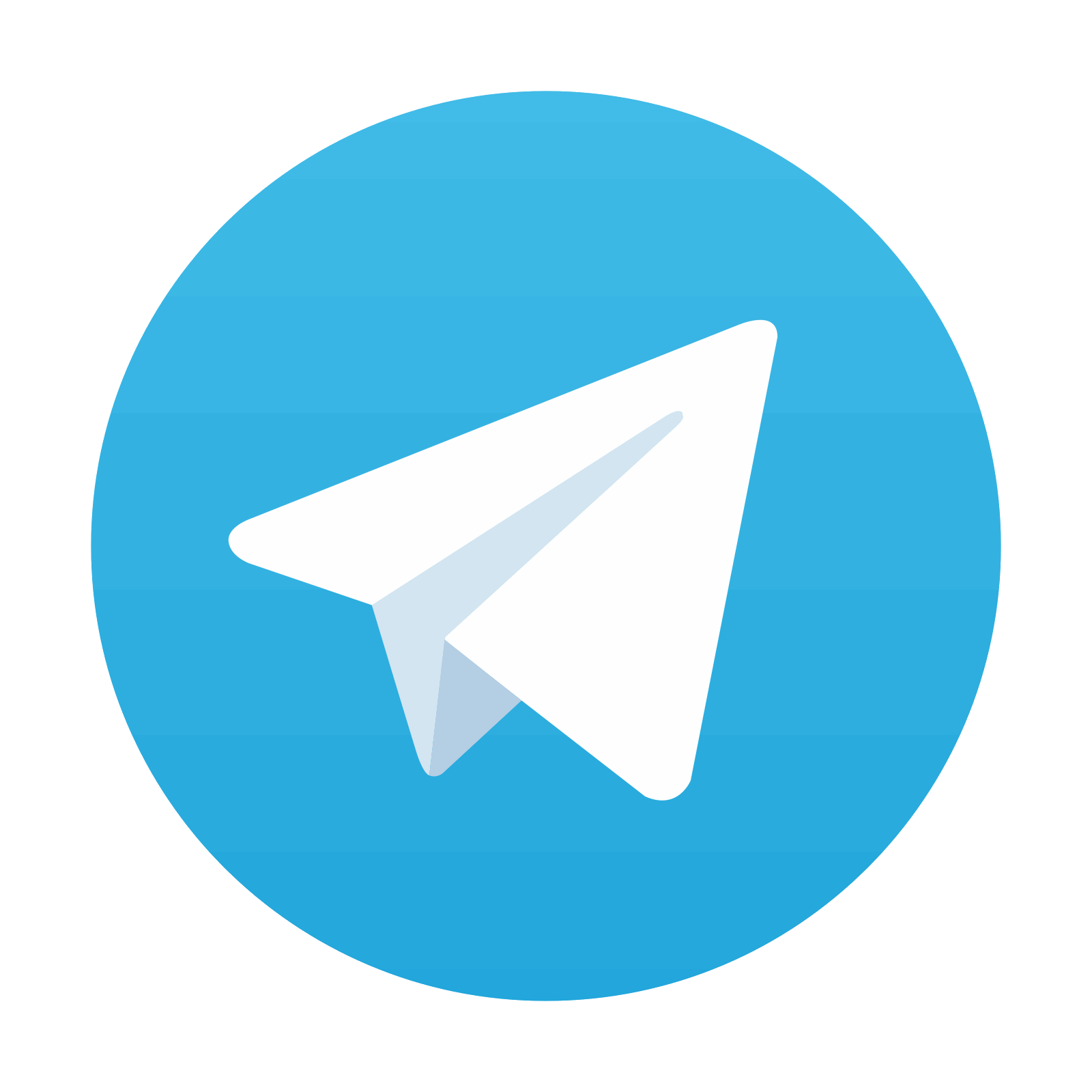
Stay updated, free articles. Join our Telegram channel

Full access? Get Clinical Tree
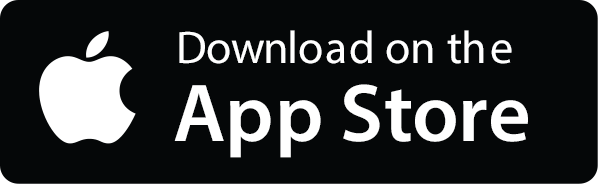
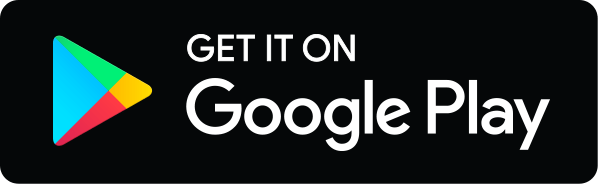