Abstract
Epilepsy surgery poses significant challenges for the neuroanesthesiologist considering the unique patient characteristics and requirements of various surgical procedures. The success of epilepsy surgery depends upon accurate localization of the epileptogenic and functional deficit zones as well as the localization of essential brain regions during presurgical evaluation. Patients thus often require anesthesia for various diagnostic and therapeutic procedures. Awareness of potential proconvulsant and anticonvulsant properties of various anesthetics and their effect on intraoperative electrophysiological mapping procedures, presence of concomitant medical problems occasionally associated with epilepsy, pharmacological properties of antiepileptic medications, and their interactions with drugs used in perioperative period is imperative for adequate management of these patients.
Epilepsy surgery done under general anesthesia has similar goals as any other neurosurgical procedure, except in patients with temporal lobe epilepsy requiring intraoperative electrocorticography and functional cortical mapping. Awake craniotomy is preferred in these patients to minimize the effect of general anesthetics on intraoperative electrophysiological mapping procedures and to optimize the extent of resection, with minimal postoperative functional deficits. In addition, anesthetists are frequently faced with patients with epilepsy undergoing emergency or elective nonneurosurgical procedure. Appropriate perioperative management of antiepileptic drugs is vital in maintaining seizure control in these patients.
Keywords
Antiepileptic drugs, Awake craniotomy, Cortical mapping, Epilepsy surgery, Intraoperative electrocorticography
Outline
Introduction 285
Surgical Management of Epilepsy 286
Types of Surgical Treatment 286
Presurgical Evaluation 287
Anesthesia for Epilepsy Surgery 288
Effect of Anesthetic Agents in Patients With Epilepsy 288
Antiepileptic Drug Interactions 290
Preanesthetic Evaluation and Preparation 291
Anesthetic Management of Preoperative Procedures 292
Anesthesia for Intracranial Electrode Insertion 294
Anesthetic Management of Resection of Seizure Focus 295
Awake Craniotomy 295
Preoperative Assessment 295
Perioperative Management 295
Premedication 296
Positioning 296
Monitoring 296
Anesthetic Regime 297
Complications 299
Resection of Epileptogenic Focus Under General Anesthesia 300
Neurostimulation for Drug-Resistant Epilepsy 301
Anesthetic Management of the Patient With Epilepsy for Incidental Surgery 302
Abbreviations 303
References 304
Introduction
Epilepsy is a clinical brain disorder characterized predominantly by paroxysmal recurring seizures. The International League Against Epilepsy (ILAE) proposed the definition as a “disorder of the brain characterized by an enduring predisposition to generate epileptic seizures and by the neurobiologic, cognitive, psychological, and social consequences of this condition.”
With an estimated incidence of 34–76 per 100,000 new cases per year (median incidence of 50.4/100,000 per year), epilepsy affects about 70 million people worldwide. The median incidence of epilepsy in developed countries is around 45.0/100,000 per year [interquartile range (IQR) 30.3–66.7] while for low- and middle-income countries it is almost double with an incidence of 81.7/100,000 per year (IQR 28.0–239.5). The higher incidence of head trauma and of infections and infestations of the central nervous system (CNS) such as malaria, neurocysticercosis, and invasive bacterial infections may be important causes for this disparity. There are very few incidence studies from India, and the one reported in 2010, suggests an age-standardized incidence rate of 27.3/100,000 per year.
Surgical Management of Epilepsy
In 2001, Wiebe et al. demonstrated the effectiveness of surgical treatment over continued pharmacotherapy in patients with long-standing pharmacoresistant temporal lobe epilepsy (TLE) with an absolute risk reduction for seizure recurrence with surgery being 50% by intention-to-treat and 56% by efficacy analysis. As a result of this study and a literature review, the American Academy of Neurology Practice Parameter concluded that surgery is the treatment of choice for medically intractable TLE (Class I Evidence).
Nonetheless, over the past decades indications for epilepsy surgery (ES) have varied considerably. The published literature is plagued with inconsistencies about ES indications and acceptable seizure outcomes, and there are no internationally accepted indications for an ES referral. Seizure severity, type, and frequency are no longer appropriate indications for ES. The ultimate goal of epilepsy treatment is now no seizures and no side effects. Curative surgical resection is a consideration in patients with drug-resistant, uncontrolled, disabling focal epilepsy (FE) if the seizures originate from a region that can be removed with minimal risk of disabling neurologic or cognitive dysfunction. ILAE has defined drug resistant epilepsy (DRE) as “failure of adequate trials of two tolerated, appropriately chosen and used antiepileptic drugs (AEDs) schedules (whether as monotherapies or in combination) to achieve sustained seizure freedom.” Sustained seizure control refers to an interseizure interval of either 1 year or three times the longest preintervention interseizure interval, whichever is longer. In appropriately selected patients with drug-resistant TLE, the combination of ES with medical treatment is four times as likely as medical treatment alone to achieve freedom from seizures.
Patients with chronic, intractable epilepsy due to discrete lesions such as mesial temporal sclerosis; low-grade neoplasms such as meningiomas, gangliogliomas, dysplastic neuroepithelial tumors, astrocytomas, and oligodendrogliomas; vascular malformations; encephalomalacia from stroke or trauma; and focal cortical dysplasias are common surgical candidates. Good surgical outcomes are also possible in patients with normal magnetic resonance imaging (MRI) if the seizures are electrographically well localized to the temporal lobe.
In tuberous sclerosis complex, resection of an epileptogenic tuber may sometimes palliate seizures. Similarly in patients with Sturge–Weber syndrome, resection of brain affected by the leptomeningeal venous angioma may control seizures. The most effective treatment is, however, hemispherectomy in patients who have developed severe hemiparesis. Hypothalamic hamartomas are rare developmental lesions attached to the tuber cinereum or the mammillary bodies, sometimes resulting in intractable epilepsy with gelastic (laughing) seizures. Complete microdissection by an endoscopic technique, or radiosurgery, can be effective.
Indications for ES differ in children from those in adults. In children, the goal of ES is to stop seizures as soon as possible to prevent epilepsy-induced encephalopathy and associated declines in cognition and neurodevelopment. Many children with early-onset epilepsy have lesions that are very large, often occupying an entire cerebral hemisphere, such as Rasmussen syndrome or hemimegalencephaly. Thus, children undergoing ES have or are at risk for neurological deficits from their etiology and the surgical procedure. Nonetheless, an early surgery can take advantage of developmental brain plasticity, which helps mitigate some of the existing and imposed neurological deficits associated with surgery.
Types of Surgical Treatment
ES usually consists of ablation of an epileptogenic zone, defined as the cortical area necessary for the generation of clinical epileptic seizures, in an attempt to achieve a seizure-free outcome. Surgical resection has been categorized into two broad categories, anatomical standardized resection and tailored resection ( Table 16.1 ). The anatomically standardized resections rely on the concept that resection of classic mesial pathology seen on imaging studies will include the epileptogenic zone, and that resection of eloquent areas will be avoided by conforming to certain anatomical boundaries. In contrast, tailored resections emphasize altering the degree of resection based on individual pathophysiology, functional mapping of eloquent cortex, and intraoperative electrocorticography (ECoG).
|
Most commonly performed curative surgical procedures include lesionectomy, anterior temporal lobectomy, corticectomy, multiple subpial transections, corpus callosotomy, and various combinations of these procedures. In addition, various other surgical alternatives have been proposed for FE, which involve selective disconnection of the epileptic foci instead of removing it. These include comissurotomies, hemispherotomy in its different varieties, temporal lobotomy and amygdalohippocampotomy. The most common surgical procedures performed in children are focal resection, hemispherectomy, and vagal nerve stimulator placement.
Presurgical Evaluation
An epileptogenic focus has been described to consist of several different zones based on the generation of the seizures and the electrophysiologic manifestations of epilepsy. The success of ES depends upon accurate localization of the epileptogenic zone, assessments of the functional deficit zone, as well as the localization of eloquent regions of brain during presurgical evaluation. Thus, an accurate prognosis for postoperative seizure control as well as functional capacities for individual patients can be achieved.
Presurgical evaluation begins with a complete history of the patient’s epilepsy, physical and neurologic examination, imaging of the brain to assess structural abnormalities, and neuropsychological testing. The structural neuroimaging techniques include MRI, diffusion-weighted imaging, tractography, electroencephalography (EEG), and magnetoencephalography (MEG).
Functional MRI, magnetic resonance spectroscopy, single-photon emission computed tomography (SPECT), and positron emission tomography (PET) scans are common functional neuroimaging methods that are currently used to evaluate patients with TLE, either through routine application or through investigational use. These tools aid the clinician in accurately assessing the lateralization of memory and language, to identify the epileptogenic region, and to predict and prevent postoperative complications. The intracarotid amobarbital (or Wada) test was initially used to localize the lateralization of language and memory functions across the left and right hemispheres. However, this test does not provide specific information for surgical guidance, depends highly on the symmetric arterial supply of the two hemispheres, and is invasive. Formal neuropsychological testing is mandatory in all patients for detection of subtle neurological alterations that are typical to each patient, tailoring of intraoperative testing according to the patient, and to assess the neuropsychological risks of surgery.
The reliability and spatial resolution of noninvasive means are generally considered too low to be the sole basis of surgical resection. Invasive monitoring by ECoG may still be needed in patients with refractory FE to better define the cortical areas to be resected and/or to minimize the risks of postoperative unacceptable deficits by cortical mapping of functional cortex. Continuous ECoG recordings also monitor for the occurrence of electrical seizures, or even clinical seizures during the resection. Despite its benefits, ECoG has certain limitations. The main shortcomings of intraoperative ECoG are the short duration of its recordings, the poorly defined influence of anesthetics, its inability to seldom capture the seizures, and the localization usually relying on interictal abnormal activity. Therefore, intraoperative ECoG is restricted to the definition of the irritative zone and has limitations for sufficiently delineating the seizure-onset zone or eloquent cortex.
Anesthesia for Epilepsy Surgery
Patients with epilepsy often require anesthesia for particular diagnostic and therapeutic procedures. In addition, certain anesthetic drugs are often employed during ES to assist in the localization of the seizure focus and in functional mapping of the brain. A continuum of critical care is thus required throughout the perioperative period, keeping in mind the unique patient characteristics and requirements of surgical procedures.
Potential concerns associated with the use of anesthesia include:
- 1.
potential proconvulsant and anticonvulsant properties of various anesthetics;
- 2.
pharmacokinetic and pharmacodynamic interactions between AEDs and anesthetic medications;
- 3.
the adverse effect profile of various AEDs;
- 4.
the presence of concomitant medical problems occasionally associated with epilepsy;
- 5.
effect of general anesthetics on intraoperative electrophysiological mapping procedures;
- 6.
delayed emergence from anesthesia (i.e., difficulty awakening) following surgery; and
- 7.
risk of perioperative seizures.
Effect of Anesthetic Agents in Patients With Epilepsy
Depending on clinical situation, many anesthetic drugs have both pro- and anticonvulsant properties. The true incidence of clinical seizure activity in individuals without epilepsy in response to general anesthetic drugs is largely unknown. In patients with a seizure disorder undergoing general anesthesia (GA) for diagnostic studies or nonneurosurgical procedures, seizures were observed in 2% anesthetic procedures during intubation or after the procedure prior to recovery from GA. Seizures associated with anesthesia occurred in 0.8% of adult procedures and 3% of pediatric procedures. In general, there is a greater propensity for anesthetics to induce epileptiform activity in patients with epilepsy than in normal controls.
Anesthetic-related seizures are caused by excitation applied to a mass of neurons, which are primed to react to the excitation by going into an oscillatory seizure state. Increased GABAergic inhibition can sensitize the cortex so that only a small amount of excitation is required to cause seizures. In addition, the ratio of affected inhibitory or excitatory neurons in both the cortical and subcortical brain structures changes with depth of sedation. Without EEG monitoring, a number of nonseizure-related movements (e.g., myoclonus, dystonic reactions, extreme shivering) could mimic seizures. EEG-identified epileptiform activity occurs most commonly with anesthetic medications during induction or emergence from anesthesia, when the anesthetic drug concentration is relatively low.
Excitatory movements including myoclonus, tremor, and dystonic posturing have been observed during induction and emergence of anesthesia with etomidate, thiopental, methohexital, propofol, enflurane, and sevoflurane.
Nitrous Oxide (N 2 O)
In humans, 70% N 2 O alone in oxygen was found to produce characteristic fast oscillatory EEG activity in a predictable fashion, persisting for up to 1 h after discontinuation, but there was no evidence of seizure activity. Nevertheless, N 2 O has been observed to cause myoclonus in volunteers exposed to hyperbaric oxygen therapy (1.5 atm) and convulsions with spike and wave activity in EEG when used in combination with isoflurane.
N 2 O either alone or in combination with other anesthetics, has been shown to have a suppressive effect on the interictal spike activity, and hence it is recommended that N 2 O should be avoided during ECoG. On the contrary, Hosain et al. and Fiol et al. did not observe any effect on interictal spikes with the use of N 2 O in doses employed routinely during ES. The authors thus recommend its use during ES without concerns about suppression of epileptiform activity. N 2 O alone has never been demonstrated to possess anticonvulsant properties in humans. In one patient with refractory convulsions, 60% N 2 O alone slowed but did not eliminate tonic-clonic or EEG seizure activity.
Inhalational Anesthetics
Sevoflurane and enflurane enhances nonspecific spike activities, while the epileptogenic potential of isoflurane, desflurane, and halothane appears low. Inhaled drugs have also been shown to affect the background ECoG by suppressing the spontaneous interictal spikes even at 1 minimum alveolar concentration (MAC) levels. Accounting for their anticonvulsant property, both isoflurane and desflurane have been used in treatment of refractory status epilepticus (RSE).
Neuroexcitatory effects of isoflurane during or following anesthesia are controversial, particularly in epileptic patients. In children undergoing intraoperative and chronic invasive ECoG, isoflurane (0.5–1%, FiO 2 0.5) decreased the spike frequency under GA but it reliably reflected the awake interictal spiking pattern when spike frequency was >1 spike/min. Among the inhaled drugs, enflurane has maximum epileptogenecity and has been shown to activate both interictal spikes and ECoG seizures. With an alveolar enflurane concentration between 2.5% and 3%, hyperventilation produces an increase in the frequency, magnitude, and synchrony of the spiking activity in epileptics. This technique has been used for pharmacoactivation of silent epileptogenic foci intraoperatively to delineate the site of seizure activity before discrete surgical excision.
Sevoflurane has a stronger epileptogenic property than isoflurane. Sevoflurane provoked seizure-like activity is seen particularly in children and where high concentrations are used, even in normal brains. Sevoflurane mask induction elicits epileptiform EEG patterns both in patients with controlled hyperventilation and also during spontaneous breathing of sevoflurane. The effect of hyperventilation on epileptogenicity of sevoflurane is, however, variable. Iijima et al. demonstrated suppression of spike activity during hyperventilation. In contrast, multiple studies report an increase in the epileptogenicity of sevoflurane when high concentrations are used in conjunction with hyperventilation regardless of its timings. Sevoflurane causes widespread nonspecific neuroexcitatory activity, and thus do not facilitate seizure focus localization in patients with TLE. Hyperventilation further exaggerates this nonspecific activation and decreases the prediction specificity of leads with ictal spikes. With balanced anesthesia technique using fentanyl-based anesthesia, sevoflurane (1.5 MAC) suppresses ECoG activities. Hence, when used for ES, careful attention should be paid to the concomitantly administered anesthetic, concentration of sevoflurane used, and ventilatory status during intraoperative ECoG.
The epileptogenic potential of desflurane is low. Compared with sevoflurane, a rapid increase in the concentration of desflurane induces tachycardia but is not associated with epileptiform EEG.
Intravenous Anesthetics
Barbiturates : EEG or clinical seizure activity has not been reported in nonepileptic patients treated with ultrashort-acting barbiturates including thiopental and methohexital. However, excitatory phenomena such as abnormal muscle movements, hiccoughing, and tremor may occur with both these drugs, more commonly with methohexital. Generalized tonic-clonic seizures (GTCS) have been reported immediately following administration of methohexital. Low-dose (<0.5 mg/kg) methohexital causes a high percentage of spike activation (50–85%) in patients with psychomotor TLE. This technique has been used during intraoperative ECoG to activate epileptic foci during temporal lobectomy. In humans, barbiturates (thiopental and phenobarbital) have well-known anticonvulsant properties and are found effective in the treatment of RSE.
Etomidate : Involuntary myoclonic movements seen during induction of anesthesia with etomidate possibly represent subcortical seizure activity. Etomidate (0.2 mg/kg) has shown up to 95% successful spike activation in patients with intracranial electrodes. During electroconvulsive therapy, etomidate is preferred over thiopental for producing seizures of adequate duration in patients who have very high seizure thresholds. At high doses, etomidate produces burst suppression patterns analogous to the barbiturate compounds. Successful termination of EEG-documented status epilepticus (SE) has been demonstrated after etomidate administration.
Ketamine : EEG seizure activity has not been reported in nonepileptic patients during ketamine administration. However, the occurrence of myoclonic and seizure-like motor activity, representing subcortical seizure activity, has been observed clinically in patients given low doses [0.5–2 mg/kg intravenous (IV)] of ketamine. Ketamine at doses ≥2 mg/kg activates epileptogenic foci in patients with known seizure disorders, but lacks specificity with ECoG. In humans, ketamine has been used successfully in the management of RSE owing to its N -methyl- d -aspartate receptor antagonist property.
Propofol : Seizures or seizure-like phenomena, which are mostly convulsive, have been observed during the induction, maintenance, and withdrawal phases of propofol administration. In a systematic review, GTCS were observed in 43%, events presenting as increased tone with twitching and rhythmic movements in 36% and involuntary movements in 16% of nonepileptic patients during and after propofol anesthesia. In patients with epilepsy, 64% had GTCS during emergence. Studies have demonstrated that sedative doses of propofol have minimal effect on intraoperative ECoG recordings. Despite the claims that propofol has proconvulsant activity, there is significant evidence to the contrary. Continuous propofol infusion is an effective treatment for RSE.
Benzodiazepines : Midazolam has been shown to induce nonepileptic abnormal movements and a dystonic posture within seconds after administration and to induce choreoathetosis following prolonged IV administration. All benzodiazepines in clinical practice (diazepam, midazolam, and lorazepam) possess potent anticonvulsant properties and are widely used to terminate episodes of SE.
Dexmedetomidine : Since locus coeruleus plays a role in dexmedetomidine-induced modulation of mesolimbic dopamine pathways, it has been postulated that the large dose and extended use of dexmedetomidine leads to a reduction in the anticonvulsant activity of the locus coeruleus due to altered noradrenergic systems. Dexmedetomidine sedation elicits an EEG pattern similar to that of stage II sleep and does not hinder EEG interpretation suggesting that it may be a uniquely useful agent for EEG recording in children. Dexmedetomidine is useful during intraoperative ECoG recording in ES as it enhances or does not alter spike rate in most of the cases, without any major adverse effects.
Opioids : Fentanyl, alfentanil, sufentanil, remifentanil, and morphine have been reported to cause seizure-like movements. Synthetic opioids can be used safely during AC without a significant increase in the risk of perioperative seizures or changes in ECoG activities. Remifentanil administered at sedation doses does not adversely affect intraoperatively recorded interictal spike activity. However, high bolus doses of all the synthetic opioids can result in their increase.
The high effectiveness (ranging from 67.4% to 100%) and specificity of μ-agonists has led to their routine use in pharmacoactivation of potential epileptiform activity during intraoperative ECoG at the time of focal cortical resection. During ECoG, alfentanil produces spike activation at wide clinical doses (20–100 μg/kg) and has been shown to be most effective and specific when compared with remifentanil and fentanyl. Alfentanil as such is the preferred opioid for intraoperative activation of the ECoG in neurosurgical patients undergoing resection of a TLE focus. Remifentanil, however, may supplant alfentanil owing to its short elimination half-life, thereby facilitating neurologic function testing immediately after surgery. Fentanyl and its analogs have not been shown to possess any anticonvulsant properties.
Local anesthetics ( LAs ): LAs can possess both proconvulsant and anticonvulsant properties because of their membrane-stabilizing effects. At subtoxic doses, LAs can act as anticonvulsants, sedatives, and analgesics followed by generalized convulsions at higher doses. High blood levels result from an accidental IV administration, accumulation after repeated injections, or rapid systemic absorption from a highly vascular area. IV lidocaine has been used to treat SE, mainly in children.
Neuromuscular blocking agents : In humans, none of the nondepolarizing muscle relaxants (NDMRs) have been reported to have any proconvulsant or anticonvulsant effects. Laudanosine, the primary metabolite of atracurium, has been known to cause EEG and clinical evidence of seizures in animals. This has not been replicated in humans, but the possibility should be considered in patients with hepatic failure in whom the half-life of laudanosine is significantly prolonged. Succinylcholine produces EEG activation with increases in cerebral blood flow and intracranial pressure (ICP) related to an increase in afferent muscle spindle activity and to increased muscle carbon dioxide (CO 2 ) production. It has not been associated with seizure activity.
Anticholinergics and anticholinesterase : In clinically relevant doses, neither atropine nor scopolamine would be expected to have a significant therapeutic effect on seizure activity in humans. Similarly, none of the cholinesterase inhibitors used in clinical anesthesia has been reported to have proconvulsant or anticonvulsant properties in humans.
Antiepileptic Drug Interactions
Awareness of pharmacological properties of AEDs and potential interactions with drugs used in perioperative period is essential for adequate management of patients undergoing ES. Most of the first-generation AEDs such as phenobarbital, primidone, phenytoin, and carbamazepine are inducers of cytochrome P450 isoenzymes involved in hepatic metabolism. Enzyme induction results in increased metabolism, decreased serum concentrations and pharmacological effect of the affected drug, and possibly loss of seizure control. The process is reversed when the inducer is withdrawn, resulting in increased serum concentrations and potential for toxic side effects of the affected drug. Hence, it is important to monitor the drug concentrations closely 2–4 weeks following addition or withdrawal of a drug. In addition, phenytoin, benzodiazepine, and valproic acid are highly protein bound. Other effects on free drug level may arise from competition for protein-binding sites.
Similarly, it is preferable to avoid enzyme-inducing AEDs in patients with chronic medical conditions other than epilepsy since two-thirds of drugs will undergo increased clearance as a result of enzyme induction, including the antiarrhythmic drugs particularly amiodarone, digoxin, calcium channel blockers (nifedipine, felodipine, nimodipine, and verapamil), β-blockers (propranolol, metoprolol), lipid-lowering agents, warfarin, antibacterial agents, antiretroviral agents, many antifungals, chemotherapeutic agents, immunosuppressives, and psychiatric medications, including some antidepressants and antipsychotics. An increase in warfarin dose is required to maintain the target international normalized ratio, with close monitoring during AED therapy in patients with epilepsy.
The net effect of AEDs (phenytoin, carbamazepine, or phenobarbital) on NDMRs is the result of several processes. At first, they inhibit the release of acetylcholine (Ach) quanta at the nerve terminal. Patients on AEDs, therefore, experience an acute increase in sensitivity to NDMRs. Over a few weeks, decreased ACh quanta results in an increase in ACh receptors. Along with induction of NDMR liver metabolism and increased release of acute-phase reactant proteins (which bind some NDMRs and change volume of distribution), this results in observed resistance to NDMRs, including pancuronium, vecuronium, rocuronium, and cisatracurium, but less so to atracurium. Moreover, epileptic patients receiving AEDs require higher doses of fentanyl to maintain a comparable depth of anesthesia. The cause or causes of this resistance to opioids is unknown. Possibilities include changes in the number of receptors and/or alterations in drug metabolism.
Macrolide antibiotics inhibit carbamazepine metabolism by inhibiting CYP3A4, giving rise to potential serious toxicity if antibiotics are administered. Valproate is an inhibitor of hepatic microsomal enzyme systems and may reduce the clearance of many concurrently administered medications, including other AEDs. Concomitant use of carbapenem antibiotics can lead to a significant decrease in serum valproate concentrations.
The newer AEDs are less interacting because their pharmacokinetics are more favorable. Gabapentin, lacosamide, levetiracetam, pregabalin, and vigabatrin are essentially not associated with clinically significant pharmacokinetic interactions. However, since the newer AEDs are often used as adjunctive therapy with older AEDs, they are susceptible to interactions by the addition of comedication.
Preanesthetic Evaluation and Preparation
Preanesthetic evaluation in patients with epilepsy should take into consideration the type of procedure to be performed. Anesthesia may be required for preoperative diagnostic studies, placement of intracranial electrodes, resection or disconnection of an epileptic focus, or placement of a neurostimulator device. The preanesthetic evaluation should include detailed history for neurologic and medical comorbidities and associated syndromes, in addition to the basic preoperative evaluation. The nature and manifestations of patient’s seizure as well as any recent change in seizure frequency or type should be known to help identify perioperative seizures accurately. While certain anesthetic agents can provoke seizures, recovery from anesthesia can be associated with shivering, myoclonus, or emergence delirium, which does not indicate epilepsy. Similarly, patients with epilepsy may experience breakthrough seizures in the perioperative period, but psychogenic nonepileptic attacks can also occur in this setting.
There are several factors unique to the perioperative period of these patients that may increase the risk of perioperative seizures including timing of AED administration, missed doses of AED, altered gastrointestinal absorption of AED, electrolyte disturbances, and sleep deprivation. In addition, a number of medications routinely used in the perioperative period can affect the seizure threshold or have significant interactions with AED (discussed previously). To prevent perioperative drug-induced seizures in epileptic patients, current AED therapy should be continued till morning of surgery or replaced with suitable alternatives if the oral administration route is unavailable. However, if the preoperative plan includes cessation of the AED to facilitate EEG monitoring and localization after grid placement, AED should be discontinued after discussion with neurologist and neurosurgeons. Regular drug dosing should be established as early as possible after surgery.
Patients with refractory seizure disorders often have associated syndromes, genetic abnormalities, or metabolic problems that may include other organ systems. Tuberous sclerosis is a multisystem genetic disorder that usually presents with cutaneous and intracranial hamartomatous lesions, the later leading to medically intractable epilepsy. Typically, the onset of epilepsy occurs in early childhood, usually in the first year of life. The hamartomatous lesion infiltrates the cardiac, renal, and pulmonary systems as well. Cardiac rhabodomyomas can be detected in a majority of these patients (more commonly in children than adults) and can lead to obstruction of intracardiac blood flow, congestive heart failure, dysrhythmias, and abnormal conduction through the bundle of His. All patients with tuberous sclerosis should have a preoperative echocardiogram and electrocardiograph to detect any functional defects. Renal lesions often result in hypertension and azotemia, both of which may complicate the conduction of anesthesia. Upper airway nodular tumors, fibromas, or papillomas involving the tongue and the palate and, less commonly, the pharynx and the larynx have also been documented, which may interfere with airway management. A diagnosis of tuberous sclerosis complex has been found to be associated with a platelet function abnormality.
In individuals with neurofibromatosis type 1, seizures (often focal) are common and are related to an intracranial neoplasm. Patients may have airway compromise from tumors involving the respiratory tract or from cranial nerve involvement. Painless dislocation of cervical vertebrae has been reported in a patient with multiple neurofibromatosis. Presence of symptoms such as dyspnea, dysphagia, or stridor should warn the anesthesiologist of potential airway complications. Pulmonary status may be compromised from chronic aspiration syndrome, chest wall deformities, pulmonary fibrosing alveolitis, cystic lung disease, pulmonary hypertension, and cor pulmonale. The cardiovascular manifestations include hypertension, which may be associated with pheochromocytoma, catecholamine-secreting nodular plexiform neurofibroma, or renal artery stenosis. Idiopathic hypertrophic cardiomyopathy may also occur. Carcinoid tumors occur in duodenum and may result in jaundice and carcinoid syndrome.
Medication history should include details of all AED and other concomitant drug therapy. A thorough search should be made for the presence of adverse effects of AED and potential drug interactions ( Table 16.2 ). Sedation and lethargy are common side effects of many AEDs and may potentiate the CNS-depressant effects of anesthetics.
Drug | Disadvantages and Adverse Drug Effects |
---|---|
Carbamazepine | Not well tolerated in the elderly (CNS side effects: cognition, diplopia, nystagmus, blurred vision, ataxia, dizziness, sedation, hyponatremia, and rash), drug interactions, hypersensitivity reactions including Stevens–Johnson syndrome and Lyell syndrome (HLA-B∗1502 allele carriers of Asian descent are at a significantly higher risk), may aggravate absence or myoclonic seizures |
Clobazam | Depression, development of tolerance, sedation |
Clonazepam | Sedation |
Ethosuximide | Narrow spectrum of efficacy, difficulty with tolerability in some patients, nausea, hiccups, anorexia, psychotic episodes. |
Felbamate | Aplastic anemia, liver failure, insomnia, gastric irritation, dizziness, blurred vision, ataxia, weight loss, drug interactions (inhibition of P450 enzymes) |
Gabapentin | Efficacy limited to partial epilepsies, inconsistent absorption, multiple daily dosing, weight gain, increased side effect risk in patients with low glomerular filtration rate, mild drowsiness, encephalopathy |
Lamotrigine | Interactions (clearance decreased by valproate, increased by estrogen, and enzyme inducers), slow uptitration, hypersensitivity reactions, hepatic and renal failure, disseminated intravascular coagulopathy, arthritis, fever, red cell aplasia, tics, insomnia |
Levetiracetam | Psychiatric side effects (mainly irritability and aggression), may cause a paradoxical increase in seizures at higher doses |
Oxcarbazepine | Drug interactions (selective P450 inducer: reduces efficacy of oral contraceptives), hyponatremia (particularly in combination with diuretics), seizure aggravation in absence or myoclonic seizures |
Phenobarbital | Drowsiness, sedation, slurred speech, nystagmus, confusion, somnolence, ataxia, respiratory depression, coma, hypotension, behavior disorders |
Phenytoin | Drug interaction (through P450 enzyme induction and extensive protein binding), nonlinear pharmacokinetics (small changes in dose or bioavailability may produce large fluctuations in level) Ataxia, rash, vertigo, slurred speech, nystagmus, diplopia, somnolence, stupor, coma, gingival hyperplasia, hirsutism, hypotension, arrhythmias, weight gain, seizure aggravation in absence or myoclonic seizures |
Pregabalin | Weight gain, aggravation of generalized myoclonic or absence seizures |
Primidone | Sedation and dizziness acutely, then similar to phenobarbital |
Tiagabine | Requires a slow titration, can cause NCSE or encephalopathy that resembles NCSE (even in the absence of prior epilepsy) |
Topiramate | Weight loss, aphasia and cognitive impairment, nephrolithiasis, metabolic acidosis, open angle glaucoma, hypohidrosis, requires slow titration rate |
Vigabatrin | Concentric visual field defects, weight gain |
Valproate | Sedation, gastric disturbance, weight gain, encephalopathy, tremor, parkinsonian syndrome, thrombocytopenia, platelet dysfunction, hepatic failure, hair loss, teratogenicity and permanent adverse cognitive outcome in fetus, drug interaction (due to inhibition of P450 enzymes and extensive protein binding) |
Zonisamide | Weight loss, aphasia and cognitive impairment, nephrolithiasis, metabolic acidosis, anhidrosis in children (fever), allergic reactions, photosensitivity |
Preoperative laboratory tests should be tailored to the proposed neurosurgical procedure. Type and cross-matched blood should be available prior to all craniotomies. Elevations in hepatic enzyme concentrations are prevalent (γ-glutamyl transpeptidase is elevated in 75% and alanine aminotransferase in 25% of patients receiving anticonvulsant therapy), hence asymptomatic elevation of these enzymes should not lead to cancellation of surgery.
Coagulation and/or platelet function abnormalities are frequent in children with refractory epilepsy undergoing ES. Carbamazepine, phenytoin, and valproic acid can cause thrombocytopenia. Additionally, valproic acid and gabapentin have been associated with acquired von Willebrand disease type 1, hypofibrinogenemia, decreased factor XIII, and abnormal platelet function. In contrast, levetiracetam does not appear to affect coagulation parameters in healthy subjects. A preoperative screening with routine coagulation tests (activated partial thromboplastin time, prothrombin time, and platelet count) and detailed history may not be adequate to fully examine underlying coagulation abnormalities. Hence, platelet aggregation studies should be considered in patients planned for ES.
Patients currently being managed with a ketogenic diet should also be identified before surgery. Ketogenic diet is a high-fat, low-carbohydrate diet that promotes ketosis and has been utilized as an adjuvant therapy for intractable epilepsy. However, this ketotic state promotes metabolic acidosis, which can be exacerbated by the use of carbohydrate-containing solutions. Therefore, patients on ketogenic diets should avoid lactated Ringers solution as the IV fluid and should receive normal saline exclusively. However, normal saline should also be administered carefully because of the risk of exacerbating patients’ metabolic acidosis. Overall, there are few case reports concerning anesthetic implications of patients who are on a ketogenic diet. Most importantly, the acid base status and plasma glucose levels should be measured frequently to avoid severe metabolic derangements during the intraoperative period. The plasma ketone body concentration should be similar to that in the preoperative period to maintain therapeutic ketosis. Hence, sugar-containing syrups may be used for premedication, depending on the strictness of ketogenic diet protocol.
A major part of preoperative planning should include a thorough discussion of the modality and type of neurophysiological monitoring to be used during the surgical procedure. Benzodiazepine premedication should be avoided in cases that may include intraoperative electrophysiologic monitoring. Intraoperatively, lower concentrations of inhaled drugs may be required to facilitate ECoG recordings. Hence, patient should be well informed of the risk of awareness before the operation.
Anesthetic Management of Preoperative Procedures
Most noninvasive modalities of presurgical evaluation of patients for ES do not require anesthesia or sedation except in pediatric or uncooperative adult patients. The level of sedation can range from anxiolysis to deep sedation. Whatever agent is chosen, there should be clear communication with the neurologists or radiologist to ensure that the sedative agent does not interfere with testing or influence the results. Both sevoflurane- and propofol-based anesthetic regimes were found suitable to detect hypometabolic cerebral lesions during PET scanning for surgical planning in children with epilepsy.
Anesthesia or sedation for MEG is, however, more complex. MEG recordings are very sensitive to anesthetic drugs as the intracellular currents and magnetic fields are extremely small. In pediatric patients, midazolam premedication was found to result in a high MEG failure rate (73%) as compared to chloral hydrate premedication and propofol maintenance (5.8%). Balakrishnan et al. demonstrated successful detection of interictal epileptiform activity during MEG in 71% of the patients who received GA (with propofol, etomidate, or sevoflurane) compared to 80% success in the larger group of nonanesthetized children as well as adults. Numerous studies have documented decrease in MEG spike frequency with propofol. Hence, propofol may not be the best drug for sedation during MEG.
Dexmedetomidine infusion may be considered ideal for MEG recordings with minimum anesthetic-related and movement artifacts. Etomidate causes an increase in spike frequency and stop movement artifacts during MEG recordings in patients with FE. Etomidate may thus, be considered for pharmacological activation of epileptic foci during presurgical evaluation using PET, SPECT, or MEG.
Anesthesia for Intracranial Electrode Insertion
Precise localization of the seizure focus in patients with generalized seizure frequently requires the insertion of intracranial grid and strip electrodes ( Fig. 16.1 ). Placement of epidural electrodes requires multiple burr holes and can be a lengthy procedure depending on the number of electrodes to be placed. Depth electrodes are multicontact, thin, tubular, and rigid or semirigid electrodes. They are placed stereotactically in the depth of sulci and can reach deep cortical structures such as the hippocampal formation or the insula. As compared to macroelectrodes, microelectrodes are usually not associated with any significant complications. Hence, only noninvasive routine monitoring is required, unless further invasive monitoring is indicated for a medical comorbidity.

Subdural electrodes can be placed in grids or strips (32-contact grid electrodes or 4 to 8 contact strip electrodes), thereby allowing coverage of large areas of cortex. However, the number of subdural electrodes that can be placed is limited by the risks of increased ICP, midline shift, and by bridging veins in the subdural space. Subdural grid electrode placement usually requires a large craniotomy and even multiple craniotomies. Strip electrodes are usually placed in the subdural space via burr holes except in patients who have had a prior craniotomy with heavy scarring, which may indicate the need for epidural placement.
The anesthetic considerations for implantation of electrodes are similar to those for craniotomy procedures in patients with epilepsy. AEDs are usually withdrawn to help detect and characterize the seizure. The test recordings derived from the cortical grids and strips requires the level of anesthesia to be decreased to minimize its depressant effects on the ECoG. Operative risk of significant blood loss and venous air embolism (VAE) necessitates the placement of large-bore IV catheters and an arterial catheter for invasive blood pressure monitoring. The electrodes are bulky, and brain shrinkage may be required. Hyperventilation should be used gently and briefly because hyperventilation may precipitate seizure activity and may be less effective in patients with complex partial seizures, who may have lower CO 2 reactivity of cerebral blood flow. Mannitol is used if additional brain relaxation is required.
Each monitoring technique carries some risks, mainly infection (1.2%) and hemorrhage (3.7%). However, no permanent morbidity or mortality has been reported. Postoperative monitoring of the patient should focus on bleeding and uncontrolled SE in the absence of AEDs. After electrode placement, chronic extraoperative ECoG monitoring is done for several days up to 2 weeks to record the typical seizure activity of patient. The aim of the recordings is to obtain three or more concordant ictal or interictal events, which is usually sufficient to delineate the site for surgical resection.
Once all monitoring is completed, intracranial electrodes need to be removed within 4 weeks of implantation because of the risk of infection. Intracranial subdural strip electrodes can be removed without an open surgical procedure by placing gentle traction on the electrodes with the patient under conscious sedation or GA. Removal of the grid requires opening of the craniotomy. If resective surgery is planned at the same time, the relationship of the grid to the underlying cortex must stay unchanged while the craniotomy is reopened. This is when the awake technique is most often required. The dura is opened, with the grid-stabilizing sutures left intact and all relations between electrode contacts and unique underlying cortical topography (e.g., blood vessels) left undisturbed. A limited resection of epileptogenic brain is performed after using bipolar stimulation to identify motor or language sites. Continuous ECoG is performed to rule out confounding subclinical seizure activity as a cause of speech or motor errors.
If resection is performed under GA, it is important to avoid administration of N 2 O until the dura is opened because intracranial air can persist for up to 3 weeks following a craniotomy. N 2 O in this situation can cause rapid expansion of air cavities and result in tension pneumocephalus.
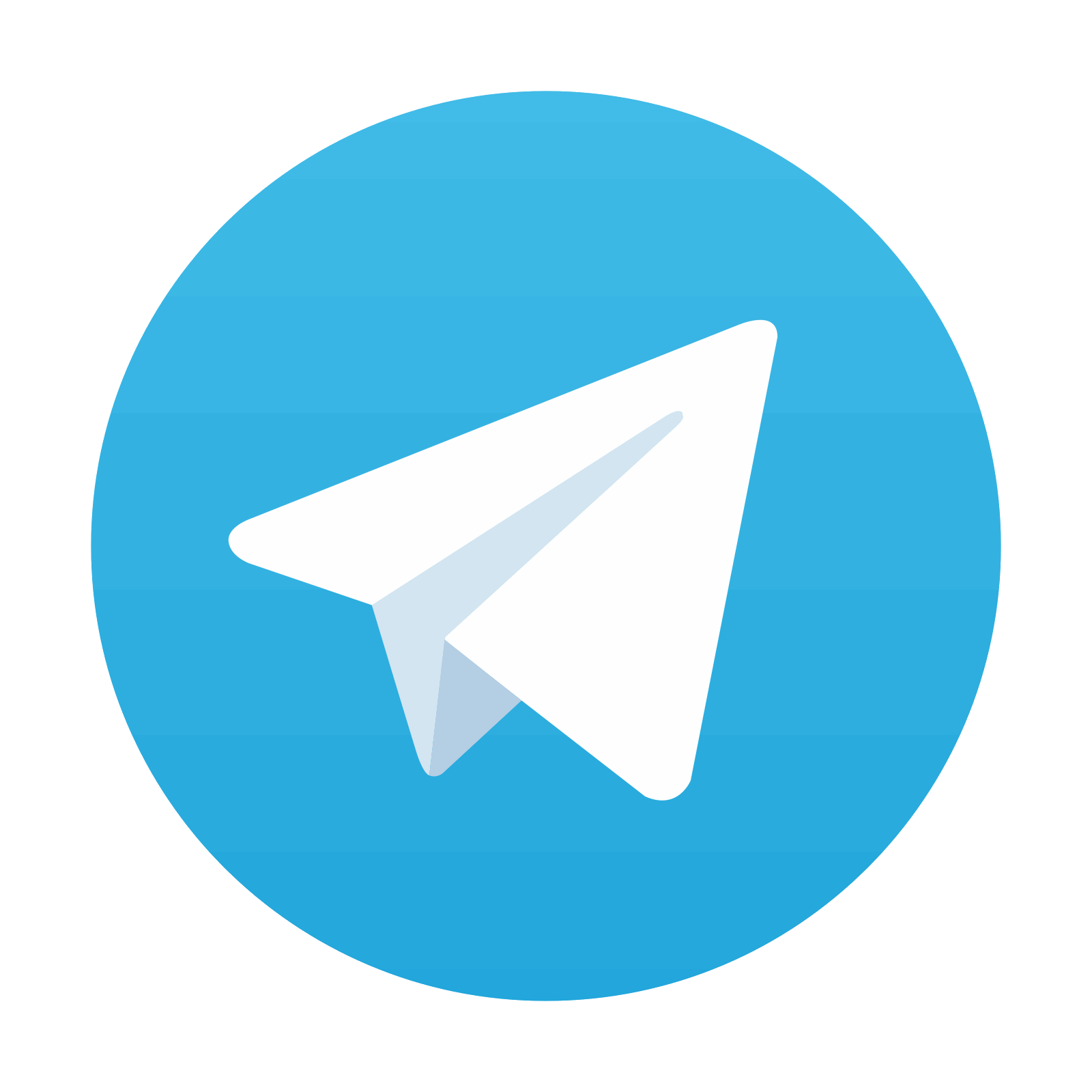
Stay updated, free articles. Join our Telegram channel

Full access? Get Clinical Tree
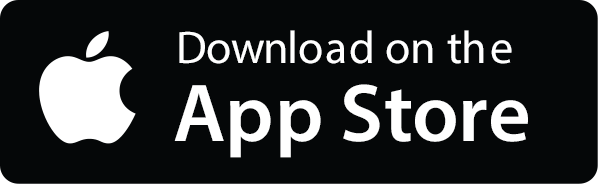
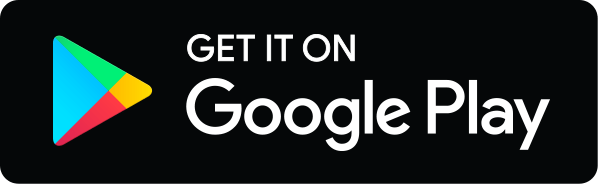