Fig. 12.1
Course of the fetal circulation in late gestation. Note the selective blood flow patterns across the foramen ovale and ductus arteriosus. From Greeley WJ, Berkowitz DH, Nathan AT. Anesthesia for pediatric cardiac surgery. In: Miller RD, editor. Anesthesia. 7th ed. Philadelphia: Churchill Livingstone; 2010; with permission
In summary, the fetal circulation possesses a physiology characterized by parallel circulations, the presence of shunts (intracardiac and extracardiac), and an increased pulmonary vascular resistance (Table 12.1). Although structural heart disease in the fetus can be associated with hemodynamic alterations and abnormal blood flow patterns, the presence of shunts to a great extent compensates for these changes, ensuring fetal viability until delivery in most cases.
Table 12.1
Characteristic features of the fetal and neonatal circulations
Feature | Fetal circulation | Neonatal circulation |
---|---|---|
Arrangement of the circulation | In parallel | In series |
Shunts | Present (essential) | Absent |
Pulmonary vascular resistance | High | Low |
Cardiac output | Low | High |
Site of gas exchange | Placenta | Lungs |
Transitional Circulation
At birth, major changes occur as follows: (1) with ligation of the umbilical cord, the placenta is excluded from the circulation and the lungs assume the function of gas exchange, (2) expansion of the lungs results in a large reduction in pulmonary vascular resistance, (3) an associated significant increase in pulmonary blood flow occurs, and (4) removal of the low-resistance placental vascular bed leads to an increase in systemic vascular resistance, a rise in LV afterload, and a decrease in the volume of blood returning to the IVC. At this time, the shunts between the pulmonary and systemic circulations during fetal life (ductus venosus, foramen ovale, and ductus arteriosus) normally close. The ductus venosus usually closes within 24 h of birth. Although the mechanisms involved in this process are incompletely understood, it is thought to occur primarily as a passive process. Postnatal functional closure of the foramen ovale occurs as the LA pressure exceeds RA pressure. An increase in LA pressure is a consequence of the marked augmentation of pulmonary venous return associated with increased pulmonary blood flow. A reduction in RA pressure is due to the concomitant decrease in IVC pressure/flow. Patency of the foramen ovale can result in atrial level shunting, the direction being influenced by the atrial pressures. It is not unusual for occasional right-to-left shunting or bidirectional shunting across the foramen to occur during the first few hours or days of life; this normally has no hemodynamic consequence. A change in the direction of shunting across the ductus arteriosus occurs, from right-to-left in fetal life to left-to-right postnatally. This change in flow fills the ductus with blood having a greater oxygen tension, which stimulates ductal closure. Constriction of the ductus arteriosus is linked to the local effect of increased oxygen tension plus the reduction in plasma concentrations of circulating prostaglandins that ensues at birth. Functional closure of the ductus arteriosus occurs within 10–25 h after birth, whereas complete obliteration of the ductal lumen occurs in the first few weeks of postnatal life. If the normal increase in the arterial pO2 does not take place, because of either pulmonary or cardiac disease, or the constrictor response to oxygen is diminished (i.e., prematurity), the ductus can remain open.
It is important to recognize that all these changes that take place at birth, essential to the normal infant, may significantly compromise the circulation of the neonate with structural heart disease [1]. A reduction or lack of shunting across anatomic fetal structures in the neonate with CHD at birth, for example, can be catastrophic postnatally until restoration of these communications is established.
Postnatal Circulation
In the neonate, the adult pattern of the blood flow through the heart is established; RV output (pulmonary blood flow) and LV output (systemic blood flow) are equal and, normally, no shunts are present. In summary, in contrast to that of the fetus, the circulation operates in series, lacks shunts, and is characterized by a progressive decrease in pulmonary vascular resistance (Table 12.1).
Cardiac Output and Distribution of Blood Flow
In the fetus, both the RV and the LV eject into the systemic circulation, denoting that cardiac output of the two ventricles is in parallel. The total volume of blood ejected by both ventricles in the fetus is referred to as the combined ventricular output (CVO). The RV contributes two-thirds of the CVO, whereas the LV ejects only one-third. A small amount of the CVO, in the range of 5 to 10 %, reaches the pulmonary circulation, and 55 to 60 % crosses the ductus arteriosus into the Desc Ao. Approximately 3 % of the CVO reaches the heart and 22 % the upper body. Only 10 % of the CVO courses through the Ao isthmus into the Desc Ao. Throughout gestation, a gradual reduction occurs in the fraction of CVO that is distributed to the placenta as the ventricular output increases to meet the increased demands of developing fetal organs [3]. Cardiac output increases immediately after birth to meet the metabolic demands of the neonate, with associated significant increases in blood flow to the lungs, kidneys, and gastrointestinal system; the LV output increases to approximate that of the RV.
Developmental Aspects in the Myocardium
The fetal myocardium is structurally and functionally immature and has limited potential to increase cardiac output. The ultrastructure of the neonatal myocardium is not well organized. The neonatal heart has fewer myocytes, the organization of the myofibrils is relatively poor, and the proportion of contractile elements is less than that of the adult myocardium [4]. In essence, the neonatal heart operates with an incompletely developed contractile system. Additional characteristics of the neonatal myocardium include the following: (1) control of contractility is more dependent on adrenal function and circulating catecholamines than on direct autonomic influences, (2) the sarcoplasmic reticulum, the primary source of calcium storage for excitation-contraction coupling, is poorly developed, and (3) deficient T tubules result in a significant dependence on transmembrane calcium fluxes [5, 6]. The neonatal heart is, therefore, not able to increase contractility under conditions of demand.
Other important aspects of the neonatal myocardium that impacts its ability to augment cardiac output include: (1) less compliant ventricles that are less able to augment stroke volume, (2) a greater dependence upon the heart rate to increase cardiac output, (3) limited tolerance to changes in preload and less ability to recruit the Frank-Starling mechanism, (4) less ability to significantly increase the contractile state, and (5) poor compensation for changes in afterload. These characteristics dictate many of the principles of neonatal cardiac perioperative practice including the frequent need for inotropic support, the value of calcium boluses/infusions, and the use of cardiac pacing. These factors also explain the greater sensitivity of the neonatal myocardium to anesthetic drugs [7]. The major differences between the neonatal and adult myocardium are summarized in Table 12.2.
Table 12.2
Summary of major differences between neonatal and mature myocardium
Parameters | Neonatal heart | Mature heart |
---|---|---|
Physiology | ||
Contractility | Limited | Normal |
Heart rate dependence | High | Low |
Contractile reserve | Low | High |
Preload tolerance | Limited | Better |
Afterload tolerance | Low | High |
Ventricular interdependence | Significant | Less |
Ca++ handling | ||
Predominant site of Ca++ flux | Sarcolemma | Sarcoplasmic reticulum |
Dependence on normal iCa++ | High | Lower |
Circulating catecholamines | High | Lower |
Adrenergic receptors | Downregulated insensitive β2, β1 predominant | Normal β1 predominant |
Innervation | Parasympathetic predominates; sympathetic incomplete | Complete |
Cytoskeleton | High collagen and water content | Lower collagen and water content |
Cellular elements | Incomplete SR, disorganized myofibrils | Mature SR organized myofibrils |
An important aspect of neonatal cardiac function is the interdependence of ventricular function. Failure of one ventricle is likely to impact the filling and hence the function of the other.
Epidemiology of Congenital Heart Disease
CHD is the most common type of birth defect in humans. In the United States, these defects affect approximately 8 out of 1,000 live births (Table 12.3) [8]. A recent systematic review and meta-analysis comprising a large study population reported that the worldwide prevalence of CHD has increased substantially over time, from <1 per 1,000 live births in 1930 to 9 per 1,000 live births in recent years, corresponding to 1.35 million worldwide live births affected with CHD every year [9]. The prevalence may be even greater than previously thought as a recent study in a large cohort of neonates undergoing echocardiographic screening revealed a live birth prevalence of CHD of 26.6 per 1,000 [10]. To further complicate the epidemiology of CHD in neonates, a large regional variation in the prevalence has been reported in various countries [11]. The prevalence of CHD in preterm infants is twice that in infants born full term [12]. Approximately 16 % of infants presenting with CHD were born preterm. CHD may also be associated with a genetic pattern of inheritance (10 %) (e.g., trisomy 18, 21, 4p deletion, and 22q11 deletion) and epigenetic and/or a syndromic pattern (as in omphalocele and Holt-Oram syndrome) [13–15].
Table 12.3
Annual birth prevalence of congenital cardiovascular defects in the United States
Type of presentation | Rate per 1,000 live births | Estimated number (variable with yearly birth rate) |
---|---|---|
Fetal loss | Unknown | Unknown |
Invasive procedure during the first year | 2.4 | 9,200 |
Detected during the first yeara | 8 | 36,000 |
Bicuspid aortic valve | 13.7 | 54,800 |
CHD is a predominant cause of death in the first year of life [16]. Without treatment, the pathology is fatal in many neonates. Increased mortality is observed in preterm infants [12]. Twenty-five percent of infants with CHD require intervention in the first year of life in order to limit mortality [17]. Neonates account for nearly 20 % of patients included in the Congenital Heart Surgery Database (The Society of Thoracic Surgeons) that undergo cardiothoracic surgical interventions each year (6,571 of 33,979 patients as per the Fall 2013 report, mostly from North American Centers), emphasizing the need to appreciate the considerations for anesthetic care in neonates.
Clinical Presentation and Diagnosis of Congenital Heart Disease in the Neonate
The presentation of CHD in the neonate depends upon the nature and severity of the pathology [18]. Although in many cases, CHD is detected during fetal screening or immediately after birth, in some instances the diagnosis is made at a later age. A particularly ominous presentation is that of the apparently healthy neonate who develops life-threatening symptoms after discharge from the nursery, which require urgent medical attention. Early recognition and appropriate management of the infant with critical CHD are essential to maximize outcome [19].
The neonate with significant CHD varies in his/her manifestations of the heart disease from no signs immediately after birth to the gradual onset of signs as their physiology changes (i.e., ductal closure, changes in pulmonary vascular resistance). The most common clinical manifestations of CHD in the neonate are respiratory distress, cyanosis, a heart murmur, and signs of reduced cardiac output [18, 20]. Respiratory distress (i.e., tachypnea, labored breathing, retractions) usually is associated with lesions that result in an increased LA volume/pressure. These symptoms may reflect pulmonary over-circulation (left-to-right shunts), obstructed pulmonary venous drainage, or pathology that leads to increased LV end-diastolic volume/pressure. Clinical cyanosis is usually apparent when the concentration of reduced hemoglobin exceeds 5 g/dL. Although often due to lung disease, cyanosis may indicate cardiac disease and is secondary to reduced pulmonary blood flow, right-to-left shunting, or mixing physiology. The presence of a heart murmur in the neonate is sometimes, but not always, associated with CHD. Conversely, serious CHD can be present in the absence of a murmur. Hypotension may indicate impending or frank hemodynamic decompensation and often implies serious pathology requiring immediate intervention, aimed at stabilization of the infant and prevention of vital organ damage.
In the neonate with suspected CHD, physical examination should include four extremity blood pressure determinations and pulse oximetry measurement in pre- and post-ductal regions. A hyperoxia test may be performed in an effort to distinguish cardiac disease from other causes of cyanosis. It consists of measuring the arterial oxygen tension (PaO2) in the right radial artery (pre-ductal site) and in a lower extremity/umbilical artery (post-ductal site) during the administration of room air and 100 % oxygen. If the cyanosis is related to pulmonary disease, supplemental oxygen can be expected to increase the PaO2 > 150 mmHg. In contrast, in the case of CHD, supplemental oxygen will have no or minimal effect, and the PaO2 will typically remain less than 100 mmHg. This response in the neonate with CHD is referred to as failure of the hyperoxia test. Additional studies such as a chest radiograph and a complete electrocardiogram that includes right-sided leads (V3R and V4R) are routinely obtained. Echocardiography is the primary modality for the initial evaluation and serial assessment of most types of CHD. It is diagnostic in most neonates, and in only selected cases are additional studies such as chest computed tomography, magnetic resonance imaging, or cardiac catheterization and angiography required to further delineate the anatomical or functional abnormalities.
Classification of Congenital Heart Disease
Numerous classification schemes have been proposed for CHD [21, 22]. These categorize malformations based on (1) complexity of the lesion as simple versus complex pathology, (2) presence or absence of cyanosis, (3) whether pulmonary blood flow is increased or decreased, (4) whether an obstruction affects the RV or LV, and (5) the direction of shunting patterns (i.e., left-to-right, right-to-left). Other classification schemes consider the underlying physiologic alterations or common features of the anomalies. An alternate approach that facilitates differential diagnosis in the neonate with CHD considers the clinical presentation and categorizes defects based on the presence of cyanosis, congestive heart failure, or murmur [23]. Yet another scheme relevant to newborn screening for CHD suggests three main categories in terms of clinical significance as follows [24]:
Life-threatening congenital heart defects: those in which collapse is likely (e.g., transposition of the great arteries, coarctation/interrupted aortic arch, aortic stenosis, pulmonary atresia, and hypoplastic left heart/mitral atresia)
Clinically significant congenital heart defects: those with effects on heart function but where collapse is unlikely (e.g., ventricular septal defect, complete atrioventricular septal defect, atrial septal defect, tetralogy of Fallot)
Clinically nonsignificant congenital heart defects: those with no functional clinical significance (e.g., ventricular septal defect only detectable by echocardiography and requiring no treatment)
This scheme is useful for determining the gravity of the situation and the immediate action needed once the diagnosis is made. Life-threatening lesions, often because of ductal dependency for pulmonary or systemic blood flow or other reasons, require prompt attention (Table 12.4). Clinically significant CHD, although important, may not have major hemodynamic manifestations within the first few weeks of life and is less likely to necessitate urgent care. Defects considered clinically insignificant have little to no potential of impacting the physiology in the neonate.
Table 12.4
Ductal-dependent congenital heart disease in the neonate
Ductal-dependent lesions for pulmonary blood flow | Ductal-dependent lesions for systemic blood flow |
---|---|
Critical pulmonary stenosis | Coarctation of the aorta |
Pulmonary atresia with intact ventricular septum | Critical aortic stenosis |
Complex lesions associated with severe pulmonary outflow tract obstruction or pulmonary atresia | Hypoplastic left heart syndrome Interrupted aortic arch |
Severe form of Ebstein anomaly with anatomic or functional pulmonary atresia | Complex lesion associated with systemic outflow tract obstruction or aortic atresia |
d-Transposition of the great arteriesa |
Although any cataloging system has limitations, a pathophysiological classification is particularly helpful in the practice of anesthesia, as it allows for the formulation of hemodynamic goals based on the main consequences of the defect. One such classification system of CHD is presented in Table 12.5 [25].
Table 12.5
Physiologic classification of congenital heart defects and respective salient features
Lesions characterized by volume overload |
• Due to communications at the atrial, ventricular, and/or arterial level |
• Frequently the result of physiologic left-to-right shunting |
• If communication proximal to the mitral valve (i.e., atrial septal defects, partial anomalous pulmonary venous return, or unobstructed total anomalous pulmonary venous return), right heart dilation is present; if the shunt is distal to the mitral valve (i.e., ventricular septal defect, patent ductus arteriosus), dilation of left-sided cardiac structures is seen |
• Symptoms related to magnitude of the shunt and pulmonary to systemic blood flow ratio |
• Shunting influenced by the pulmonary vascular tone and relationships between the pulmonary and systemic vascular resistances |
• Diuretic therapy and afterload reduction are main medical management strategies |
Lesions characterized by obstruction to systemic blood flow |
• Include those with ductal-dependent systemic blood flow (i.e., critical aortic stenosis, severe aortic coarctation, aortic arch interruption, hypoplastic left heart syndrome) |
• Prostaglandin E1 therapy required to maintain ductal patency and systemic blood flow until an intervention is undertaken |
• Diuretic therapy and manipulation of the systemic and pulmonary vascular resistances may be required to control increased pulmonary blood flow |
• Inotropic and/or mechanical ventilatory support frequently necessary |
Lesions characterized by obstruction to pulmonary blood flow |
• Include those with ductal-dependent pulmonary blood flow (i.e., critical pulmonary valve stenosis and pulmonary atresia with intact ventricular septum) |
• Prostaglandin E1 therapy is indicated for the treatment of arterial hypoxemia until obstruction can be relieved or alternate sources of pulmonary blood flow are established |
Parallel circulation |
• Classic lesion is that of d-transposition of the great arteries where the pulmonary and systemic circulations run in parallel |
• Associated with cyanosis |
• Intercirculatory mixing is essential for survival |
Single ventricle lesions |
• Include those with atrioventricular valve atresia (i.e., tricuspid atresia), severe ventricular hypoplasia (i.e., double-inlet left ventricle), or anomalies where a biventricular repair is not feasible (i.e., unbalanced atrioventricular septal defect) |
• Common among these lesions is complete mixing of the systemic and pulmonary venous blood at the atrial or ventricular level, and aortic or pulmonary outflow tract obstruction, accounting for the presence of cyanosis |
• An important goal of early management involves optimization of the balance between the pulmonary and systemic circulations |
Congenital Cardiovascular Anomalies of the Neonate: Anatomy, Pathophysiology, and Management, with Anesthetic Considerations
The progressive advances in perioperative care that have taken place during the last 50 years have allowed the evolution of neonatal congenital heart surgery and resulted in increased survival rates and greatly improved outcomes [26]. These advances have permitted new approaches for many lesions; early corrective surgery is now preferred to initial palliation and later repair. The rationale for early correction is based on the premise that by restoring the anatomy and physiology towards normal early in life, subsequent morbidity will be minimized, thus permitting the most favorable long-term outlook. As a consequence, the volume of neonatal corrective surgery has markedly increased.
Patent Ductus Arteriosus
Anatomic Features
The ductus arteriosus is a vascular communication between the pulmonary artery (PA) and the Desc Ao (Fig. 12.2). This structure is an essential component of fetal life, serving as a conduit for RV output into the Desc Ao. In some cases, normal closure does not take place, resulting in persistent ductal patency. Prematurity and respiratory distress syndrome are risk factors for developing a patent ductus arteriosus (PDA). This lesion affects nearly a 33 % of infants weighing less than 1,500 grams at birth. PDA occurs with increased frequency in certain genetic disorders (e.g., Holt-Oram syndrome), in association with in utero viral infections (Rubella) and within utero drug ingestion (sodium valproate) [27].


Fig. 12.2
Graphic representation of a patent ductus arteriosus. The communication between the aorta (Ao) and pulmonary artery (PA) is shown. The usual direction of shunting, from left-to-right, in the isolated lesion is noted. IVC inferior vena cava, LA left atrium, LV left ventricle, RA right atrium, RV right ventricle, SVC superior vena cava
A PDA may be found in isolation or in association with other forms of CHD. In the isolated form, it accounts for nearly 10 % of all congenital heart defects. It is important to recognize that in some cardiovascular malformations, ductal patency may be essential for either pulmonary or systemic blood flow and, therefore, survival. The following discussion addresses the isolated defect.
Pathophysiology
Communication at the level of the great arteries permits shunting between the systemic and pulmonary circulations. Two factors determine the direction and magnitude of the shunting: the relative vascular resistances of the pulmonary and systemic vascular beds and the size of the communication. The pulmonary vascular resistance significantly affects the direction of shunting. As the resistance decreases postnatally, the typical direction of blood flow through an isolated PDA is left-to-right, resulting in increased pulmonary blood flow and volume loading of the heart.
The clinical manifestations of a PDA depend on the magnitude of the shunt and the pulmonary and cardiac responses to the shunt. Some shunts are restrictive (small diameter) in nature and limit the blood flow to some extent. Other shunts are large communications that permit a large blood flow from the Ao to the PA. In the latter case, the neonate may develop signs of pulmonary overload (labored breathing, radiologic evidence of increased interstitial lung water, LA/LV dilation, cardiomegaly) and overt congestive heart failure. The preterm neonate is particularly vulnerable to the hemodynamic effects of a left-to-right shunt, and pulmonary edema can develop [28]. Frequently, a PDA is associated with respiratory impairment, a requirement for mechanical ventilatory support, and/or failure to wean from support. PDA in preterm infants is a risk factor for bronchopulmonary dysplasia and intracranial hemorrhage. The diastolic runoff, (left-to-right shunt) also called pulmonary steal, causes hypoperfusion of systemic vascular beds with potential end-organ complications (i.e., impaired myocardial perfusion, necrotizing enterocolitis). The lack of significant ductal restriction and the increased pulmonary blood flow leads to pulmonary hypertension, imposing a further pressure load on the RV.
Management
The goals of medical therapy are designed to control pulmonary over-circulation and ventricular volume overload. In most cases, this includes fluid restriction and diuretic therapy [29]. The administration of indomethacin or ibuprofen in an effort to alter prostaglandin metabolism and promote ductal closure in the premature infant is a well-established clinical practice [30]. Drug therapy may not be effective in the very low-birth-weight infant. It may actually be contraindicated due to side effects affecting renal, gastrointestinal, and cerebral perfusion. Surgical management usually consists of placing a clip on the communication, most commonly via a small posterior lateral thoracotomy. Although ductal closure using video-assisted thoracoscopy has been reported in the neonate and even in preterm infants, this approach is more likely to be considered for the older infant or child [31, 32]. A catheter-based procedure to occlude the PDA is an option in the term infant.
Anesthetic Considerations
The need for intervention for a hemodynamically significant PDA in the full-term neonate is extremely rare and is more likely to occur if other coexisting disease is present. Infants undergoing catheter-based therapies may suffer vascular injury, rhythm disturbances, and hemodynamic instability. These problems result from factors such as difficulty with vascular access, catheter manipulations, or blood loss. In the neonate undergoing surgical ductal ligation, standard anesthetic practice for thoracotomy procedures in the small infant should be followed. The use of regional anesthesia techniques can improve perioperative pain management. If the premature infant requires surgical ligation, a left thoracotomy is often performed in the neonatal intensive care unit in many centers, obviating the need to transport the infant to the operating room, thereby decreasing the risk of problems such as hypothermia and accidental tracheal extubation. This is particularly useful if the infant requires high-frequency oscillating ventilation. An opioid-muscle relaxant-based intravenous anesthetic technique is the most common practice.
Specific Issues
Intravascular volume. Fluid restriction and diuretic therapy in the neonate with a PDA can result in intravascular volume depletion in the presence of congestive symptoms. This problem alone or in combination with surgical manipulations that impair ventricular filling can predispose the infant to hemodynamic alterations during the ligation procedure, necessitating fluid administration or other acute interventions.
Ventilation. Ductal ligation requires manual manipulation of thoracic structures and retraction of the non-dependent lung to achieve optimal surgical exposure. These maneuvers frequently impair gas exchange to some extent. Therefore, vigilance is of outmost importance combined with monitoring the arterial saturation measured by pulse oximetry (SpO2) continuously and assiduously. Preoperative placement of additional “reserve” oximetry probes may be considered advisable! Frequent adjustments to the ventilation parameters, guided by variables being monitored to optimize gas exchange, may be necessary.
Pulmonary blood flow. An important hemodynamic goal prior to surgical ligation is to minimize increases in pulmonary blood flow that can compromise systemic output or myocardial function. Although limiting the inspired oxygen concentration is desirable in this instance, in addition to the concern of the risk of retinopathy of prematurity (refer to Chap. 10), this objective should be balanced with that of providing adequate systemic oxygen delivery during the procedure [33].
Blood loss. Even a small amount of blood loss can have a major hemodynamic impact in the preterm or term neonate due to the relatively small blood volume. Thus, as in any major vascular surgery, appropriate vascular access and immediate availability of blood products is imperative. Blood loss is usually minimal, but disastrous hemorrhage can occur.
Anemia. Many preterm infants are anemic and this increases the risk of congestive heart failure. In many infants, red cell transfusion to correct anemia will significantly improve the cardiac status.
Inadvertent ligation of other structures. Unintentional ligation of adjacent thoracic structures such as the left pulmonary artery (LPA), left mainstem bronchus, and Desc Ao is a well-known complication during ductal ligation [34]. Pre- (right upper extremity) and post-ductal (lower extremity) monitoring with a pulse oximeter/blood pressure cuff in some cases can permit early recognition of these complications. Ductal closure is associated with narrowing of the pulse pressure and an increase in diastolic and, frequently, systolic blood pressures. Confirmation of these changes during ductal ligation is reassuring.
Postoperative problems. Other important issues that are associated with ductal ligation deserve mention. One is the potential for nerve injury and related morbidity. The recurrent laryngeal nerve courses around the ductus arteriosus and can be injured during the procedure, resulting in vocal cord paralysis [35]. Diaphragmatic paralysis secondary to phrenic nerve injury leading to significant ventilator dependency also has been reported [36]. Chylothorax due to thoracic duct injury is a rare complication. Other concerns after ductal ligation in the preterm infant relate to the effects of obliteration of the left-to-right shunt on cardiopulmonary function. In some cases, the acute increase in LV afterload can be detrimental to myocardial performance, particularly in the presence of preexistent ventricular dysfunction, [37] which can manifest as a low cardiac output state and hypotension. Others may have a potential immediate need for increased mechanical ventilatory support due to changes in pulmonary compliance.
Coarctation of the Aorta
Anatomic Features
Coarctation of the aorta (CoA) represents 5 to 8 % of all congenital cardiovascular defects. The anomaly is characterized by narrowing of the lumen of the thoracic Ao near the region of the ductus arteriosus or ligamentum, resulting in obstruction to the systemic blood flow (Fig. 12.3). The constriction can be discrete or diffuse. In the neonate, this lesion can be part of a complex setting with hypoplasia of the transverse Ao arch and Ao isthmus (region proximal to the coarctation between the left subclavian artery and the ductus arteriosus). This constellation of findings is less likely to occur in older infancy or later in life.


Fig. 12.3
Graphic representation of coarctation of the aorta. The posterior shelf in the descending thoracic aorta (Ao) in this lesion is shown. IVC inferior vena cava, LA left atrium, LV left ventricle, PA pulmonary artery, RA right atrium, RV right ventricle, SVC superior vena cava
Associated pathology in CoA may include a PDA, bicuspid Ao valve, aortic stenosis (AS), subaortic obstruction, and ventricular septal defect (VSD). CoA also can be part of the group of defects identified in Shone’s complex, characterized in addition by subaortic obstruction, parachute mitral valve, and supravalvar mitral ring [38].
Pathophysiology
This lesion has a spectrum of severity, as is the case in many other forms of CHD; presentation in a young infant usually implies more severe obstruction or the presence of coexisting anomalies. The hemodynamic consequences of CoA relate to obstruction of the systemic blood flow and inadequate distal perfusion. When the PDA provides most or essentially all of the entire distal systemic perfusion via the RV, CoA is considered a ductal-dependent lesion for systemic blood flow. Ductal constriction will have profound repercussions in this setting. In the case of less severe obstruction, ductal closure results in increased LV afterload and end-diastolic pressure, myocardial work, and wall tension, which assumes a potential for myocardial ischemia. Increased LA pressures may lead to left-to-right shunting at the atrial level and increased pulmonary blood flow. Pulmonary hypertension results from increased pulmonary venous pressures related to LA hypertension and from the increased pulmonary blood flow. Coexistent defects can contribute to the volume or pressure load of the myocardium.
Management
Neonates with severe obstruction frequently require treatment for congestive heart failure and possible ventricular dysfunction. A presentation of poor cardiac output is more ominous as manifested by decreased peripheral perfusion, metabolic acidosis, lactic acidemia, renal insufficiency, poor ventricular function, or shock [39]. This condition requires immediate intervention with tracheal intubation and mechanical ventilation in addition to prostaglandin E1 (PGE1) therapy to reestablish/maintain ductal patency and improve systemic perfusion. Additional care includes inotropic support and, potentially, cautious afterload reduction. In most cases of moderate to severe narrowing, surgical relief of the obstruction is the treatment of choice. Selection of the surgical approach, median sternotomy versus lateral thoracotomy (discussed later in the chapter), is influenced by the associated pathology, particularly the presence of arch hypoplasia. The goal of the surgical procedure, regardless of the details of the specific technique or approach, is to resect the narrowed segment and remove adjacent ductal tissue that can be part of the pathology and carries the potential for recurrence of the obstruction. Aortic continuity in the neonate is established preferably using native tissue. Associated significant Ao arch hypoplasia requires more extensive reconstruction [40, 41].
Endovascular balloon dilation has been performed in the neonate, even in those with significant transverse arch hypoplasia [42]. Many centers, however, favor surgical intervention to achieve the most effective long-term outcome.
Anesthetic Considerations
Adequate vascular access is essential. Related to Ao cross-clamping is a potential for acidosis and end-organ dysfunction (the spinal cord, kidneys, gut) due to hypoperfusion of respective vascular beds. This concern is usually less for older children because of the development of collateral circulation. Several strategies have been used in the neonate and young infant in efforts to minimize the potential for spinal cord injury [43]. Inducing mild hypothermia to 34–35 °C before applying the Ao clamp is standard of care at some centers, although the incidence of spinal cord injury in the absence of hyperthermia is exceedingly rare [44]. Hemodynamic changes during CoA surgery via thoracotomy include hypertension upon application of the Ao cross-clamp, hypotension associated with its release, and paradoxical or rebound hypertension after the repair is complete. This latter problem has been linked to altered baroreceptor responses and abnormalities in the renin-angiotensin system [45]. Recent data also suggest that underlying pathological adjustment of autonomic cardiovascular homeostasis occurs in these infants [46].
Specific Issues
Arterial pressure monitoring. The selection of monitors (sites and types) varies according to the surgical plan and specific approach (e.g., lateral thoracotomy versus median sternotomy; resection with end-to-end repair, extended Ao arch repair, Ao arch advancement, and the need for cardiopulmonary bypass). Monitoring of arterial blood pressure at sites proximal and distal to the obstruction should be considered. Right radial artery blood pressure monitoring is ideal in most cases. A blood pressure cuff in a lower extremity can also be helpful.
Surgical considerations. In view of the fact that associated Ao arch hypoplasia requires more extensive reconstruction aimed not only at immediately relieving the obstruction but also at providing the best long-term outcome, cardiopulmonary bypass (CPB) is frequently needed that may include the use of special bypass techniques (e.g., selective cerebral perfusion). Depending on these strategies, additional monitors (e.g., neuromonitors) may enhance the safety of these interventions.
d-Transposition of the Great Arteries
Anatomic Features
d-Transposition of the great arteries (d-TGA) accounts for 6 % of all CHD. This malformation is identified in 10 % of neonates with critical heart disease and represents the most common cause of cardiac cyanosis during the neonatal period. A male predominance is seen among affected infants.
In d-transposition the Ao arises from the anatomic RV and the PA from the LV (Fig. 12.4). In most cases, the Ao is spatially oriented anterior and to the right of the PA, in contrast to the posterior location of the Ao in the normal heart. This malformation is thought to result from abnormal rotation and septation of the conotruncus, resulting in discordant ventriculoarterial connections.


Fig. 12.4
Graphic representation of d-transposition of the great arteries. The discordant connections between the ventricles and great arteries in this lesion are depicted. Intercirculatory mixing is essential for survival in this lesion and is allowed for by flow across a patent ductus arteriosus (PDA) and atrial septal defect (ASD). Ao aorta, IVC inferior vena cava, LA left atrium, LV left ventricle, PA pulmonary artery, RA right atrium, RV right ventricle, SVC superior vena cava
In the most common form of the defect, an intact ventricular septum or a small VSD is present. d-Transposition is associated with a VSD in 10 to 25 % of cases. In complex forms of the defect, a large VSD and various degrees of pulmonary stenosis (PS) or left ventricular outflow tract (LVOT) obstruction is seen. Other anomalies include additional VSDs, coronary artery variants, and Ao arch obstruction.
Pathophysiology
In d-TGA, the systemic and pulmonary circulations operate in parallel (separate) rather than in series. This anomaly results in recirculation of blood, deoxygenated blood in the systemic circulation and oxygenated blood in the pulmonary circulation. Intercirculatory mixing is essential for survival. The typical presentation is that of cyanosis shortly after birth as the ductus closes in an otherwise healthy-appearing neonate. There is minimal or no response to the administration of oxygen. A restrictive foramen ovale can result in poor mixing and profound arterial hypoxemia, potentially progressing to metabolic acidosis and severe shock due to compromised tissue oxygenation. Less commonly, a high pulmonary vascular resistance accounts for severe cyanosis despite ductal patency and an adequate anatomic interatrial communication.
Symptoms of congestive heart failure are not likely to occur even in the presence a PDA, VSD, or CoA within the first few days of life. In the case of concomitant shunts such as at the ventricular or ductal levels, the relative high pulmonary vascular resistance limits significant shunt volume.
Management
Without intervention, this defect is almost uniformly fatal. Preoperative management is determined by the adequacy of mixing between the parallel circulations. PGE1 therapy frequently is used to enhance intercirculatory mixing. The goal of maintaining ductal patency is to increase the pulmonary blood flow and the volume of pulmonary venous blood returning to the LA, which in turn raises the LA pressure to the extent that it stretches the interatrial communication, enhancing intercirculatory mixing. Balloon atrial septostomy is required to enlarge a restrictive interatrial communication in the presence of significant hypoxemia.
The arterial switch operation (Jatene procedure) is the surgical procedure of choice for uncomplicated d-transposition (Fig. 12.5). The intervention achieves anatomic correction and restores normal physiology. The operation involves transection of the great arteries above their semilunar valves, anastomotic connections to their appropriate respective outflows, translocation of the coronary arteries to the neoaortic root, closure of existing intracardiac communications, and repair of additional defects, as indicated. Early in the surgical experience, certain coronary patterns were considered to preclude this type of surgery, given the need to mobilize and reimplant these tiny vessels. Today, however, the concern is much less, and, in fact, many centers do not regard abnormal coronary patterns a contraindication for the arterial switch operation. Timing of the operation is relevant as it is undertaken before pulmonary vascular resistance falls in order to prevent deconditioning of the LV, given that it will become the systemic ventricular chamber upon completion of the operation [47]. Hence, in most cases, this type of surgery is performed within the first few weeks of life while the LV afterload is high. Beyond the neonatal period, the approach to this lesion depends on numerous factors, but importantly on the ability of the LV to support the systemic circulation based on the presence or absence of associated defects and their impact on LV “preparedness.”


Fig. 12.5
Graphic representation of the arterial switch operation in d-transposition of the great arteries. Upper left panel, the ductus arteriosus/ligamentum is divided, and the great arteries are transected above the level of their native semilunar valves and roots. Coronary artery buttons are then removed from the typically anterior and rightward aorta. Upper right panel, the coronary arteries are translocated to the posterior “neoaortic” root (native pulmonary root). Left lower panel, the Lecompte maneuver is performed allowing for the main pulmonary artery to be mobilized in front of the neoaorta. Right lower panel, the aortic anastomosis is completed and after repairing the defects in the native aorta (“neopulmonary artery”) where the coronary buttons were removed, the pulmonary arterial anastomosis is completed
Anesthetic Considerations
If balloon atrial septostomy is required prior to performing corrective surgery, it can be undertaken at the bedside or in the cardiac catheterization laboratory. Frequently, the intervention is of an urgent nature due to profound arterial hypoxemia. In this situation, the main goal is to maintain cardiovascular stability while an atrial communication is enlarged or created. Marked clinical improvement is seen with adequate atrial mixing. Access to emergency equipment, medications, and blood products is essential in this setting. Some centers routinely perform balloon atrial septostomy in most affected neonates to allow for adequate mixing while awaiting surgical intervention.
Specific Issues
Myocardial ischemia. Translocation of the coronary arteries involves manipulation, probing, and, in some cases, potential stretching or distortion of the vessels. These maneuvers in addition to other aspects of the repair can predispose the neonate to coronary artery spam and/or myocardial ischemia. Thus, these cases require careful surveillance for potential compromise of myocardial blood flow (electrocardiographic monitoring of ST segments, regional wall motion assessment by transesophageal echocardiography, or TEE) and a low threshold to consider inadequate coronary blood flow as the etiology of ventricular dysfunction, failure of separation from CPB, or intractable ventricular arrhythmias. At some centers, the postoperative administration of a nitroglycerine infusion in these neonates is routine.
Left ventricular compliance. The LV in the neonate with d-transposition is relatively “stiff” or noncompliant immediately after surgery, which is manifested by extreme sensitivity to the administration of volume, resulting in a significant increase in LA pressure and a fall in cardiac output. Chamber overdistension is poorly tolerated, so fluids should be administered cautiously and guided by LA pressure, systemic arterial blood pressure, and qualitative echocardiographic assessment of LV size.
Pulmonary hypertension. Because surgical correction is performed early in infancy prior to the normal fall in pulmonary vascular resistance, pulmonary hypertension can be a problem in the immediate post-bypass period and postoperatively. Strategies that support the RV, as well as the use of pulmonary vasodilators, should be considered.
Tetralogy of Fallot
Anatomic Features
Tetralogy of Fallot (TOF) is the most common cause of cyanotic heart disease in childhood, accounting for approximately 6 to 11 % of CHD. The absence of cyanosis or the failure to recognize mild cyanosis immediately after birth explains why TOF is often diagnosed beyond the neonatal period and is not recognized as the most common cyanotic heart defect in the neonate.
The four components of TOF in the classic lesion include a large VSD, right ventricular outflow tract (RVOT) obstruction, Ao override, and RV hypertrophy (Fig. 12.6). The wide spectrum of clinical manifestations in this lesion is due to the variable anatomic features, particularly the severity of the RVOT obstruction. Associated pathology includes an atrial communication, additional VSDs, a persistent connection from a left superior vena cava (LSVC) to the coronary sinus, coronary artery anomalies, and variants of Ao arch laterality or branching pattern. Concomitant lesions such as that of a complete atrioventricular septal defect can be present in some cases (colloquially referred to as “tet-canal” defect). Pulmonary atresia with a VSD is considered to represent an extreme form of TOF.


Fig. 12.6
Graphic representation of tetralogy of Fallot showing the classic features of this lesion: ventricular septal defect, pulmonary stenosis, overriding aorta (Ao), and right ventricular hypertrophy. The potential various levels of right ventricular outflow tract obstruction are noted (subvalvar, valvar, and supravalvar). The right-to-left shunt across the ventricular septal defect (shown by the arrow) accounts for the presence of cyanosis in this defect. IVC inferior vena cava, LA left atrium, LV left ventricle, PA pulmonary artery, RA right atrium, RV right ventricle, SVC superior vena cava
Pathophysiology
The RVOT obstruction in TOF, which is often found at multiple levels, is characterized by variable dynamic and fixed components. The dynamic obstruction occurs only in the muscular infundibular region. In contrast, the fixed component occurs at the subvalvar, valvar, and supravalvar regions and/or the branch pulmonary arteries. Cyanosis is caused by decreased pulmonary blood flow and right-to-left shunting across the VSD, implying lower pulmonary blood flow relative to systemic blood flow. The nonrestrictive nature of the VSD together with the RVOT obstruction account for equalization of RV and LV pressures. Hypercyanotic episodes (“tet spells”) result from increases in RVOT obstruction and right-to-left intracardiac shunting, which can lead to significant morbidity or even death. Fortunately, it is a very unusual occurrence during the neonatal period.
TOF has a spectrum of clinical presentations. At one end is the large VSD and minimal RVOT obstruction. In this case, shunting across the ventricular communication is typically in the left-to-right direction and the neonate is acyanotic. In fact, some degree of heart failure from pulmonary over-circulation often is present, accounting for the “pink” form of tetralogy. At the other end of the spectrum is the neonate with severe pulmonary stenosis/near pulmonary atresia who is quite cyanotic, ductal dependent, and in need of an intervention to establish a reliable source of pulmonary blood flow. In the middle of this continuum is the “classic” form of TOF, characterized by moderate pulmonary outflow tract obstruction and associated mild cyanosis at rest (systemic oxygen saturation ~ 80 to 90 %). This condition is the case in most neonates, although increasing cyanosis can occur with crying, agitation, or pain.
A particular variant of TOF with potential significant clinical implications in the neonatal period is that associated with absent pulmonary valve syndrome. This pathology is characterized by a dysplastic pulmonary valve, various degrees of valvar stenosis, and regurgitation, in addition to the usual findings in TOF. Massive dilation of the main and branched pulmonary arteries in this defect accounts for abnormalities of the tracheobronchial tree. These abnormalities can result in severe respiratory compromise due to air trapping and are frequently associated with significant morbidity related to poor lung mechanics.
Management
Ongoing controversy exists regarding the favored surgical approach in the neonate or very young infant with TOF associated with severe RVOT obstruction and significant cyanosis [48–50]. Some centers advocate initial palliation consisting of a systemic-to-PA shunt, most frequently in the form of a modified Blalock-Taussig shunt (graft between subclavian artery and PA), via a lateral thoracotomy or median sternotomy approach, followed by corrective surgery later in infancy. Others prefer, even in very young infants, a single-stage definitive repair consisting of closure of the VSD, relief of the RVOT obstruction, and repair of associated defects. Many technical aspects of the surgical intervention are variable and surgeon/institution dependent [51]. The relief of the RVOT/pulmonary obstruction can be accomplished through transatrial and/or PA access. An incision across the pulmonary annulus and placement of a transannular patch is necessary in some cases, usually related to annular hypoplasia, which is more likely to be the case in younger infants. With regard to closure of the VSD, the approach can be a transatrial, transventricular, or combined, based on the surgeon’s preference. In view of the fact that ventriculotomy, or the need for a transannular patch, can impact on the long-term outcome, some prefer palliation rather than total correction in very young infants [52]. Alternative medical temporizing measures at some centers include the use of beta-adrenergic receptor antagonists to minimize the risk of infundibular “spasm” and associated hypercyanotic events. In selected cases, cardiac catheterization procedures such as percutaneous balloon pulmonary valvuloplasty or stenting of the ductus arteriosus can be beneficial while the infant awaits corrective surgery.
Anesthetic Considerations
During anesthetic care, the main concern in the unrepaired neonate with TOF is the potential for hypercyanotic episodes that can result in profound hypoxemia and significant morbidity. These episodes can be triggered by crying, light anesthesia, hypovolemia, sympathetic stimulation (or sympathomimetic drugs), or anesthetic-related decreases in systemic vascular tone with augmentation of the right-to-left shunting. Immediate management of an acute spell usually requires titration of a systemic vasoconstrictor drug (phenylephrine, norepinephrine, or vasopressin). Augmenting the cardiac preload by administering fluids to facilitate forward flow across the obstructed RVOT can also help. Beta-blockers (e.g., esmolol or propranolol) can be given in an effort to decrease heart rate and enhance diastolic filling time, while also relaxing the dynamic RVOT, but should be used with caution in the neonate. A measure that is usually considered once the neonate has been stabilized is blunting of the sympathetic tone by increasing the anesthetic depth by the administration of a sedative, opioid, or inhalational agent. Carefully titrated inhalational agents can be especially advantageous in view of their cardiac depressant effects, especially as exerted on the RVOT. Halothane was ideal for the child with TOF but is not readily available now. Isoflurane is a good second best! Rarely during cardiac surgery, a hypercyanotic episode is refractory to treatment and emergent initiation of CPB is required. The main goals of anesthetic care of the neonate with TOF are to preserve myocardial function, promote forward pulmonary blood flow, and minimize the potential for further increases in right-to-left shunting. Even issues that may not significantly influence the physiology of other forms of CHD can impact that of the neonate with TOF. An example is the potential detrimental effect of mechanical ventilation on intrathoracic pressure, further limiting pulmonary blood flow.
Specific Issues
Pulmonary vascular resistance. Although, in general, pulmonary vascular tone does not play a major role in this lesion, the normally increased pulmonary vascular resistance in the neonate can impede forward flow across the outflow tract. It can have important implications regarding perioperative management and surgical decision-making as it complicates the assessment of the severity of the RVOT obstruction. Therefore, a reasonable anesthetic goal is to minimize acute increases in pulmonary vascular tone.
Coronary artery anomalies. These anomalies are present in 5 to 12 % of patients with TOF and potentially impact the surgical procedure. The left coronary artery, for example, can originate anomalously from the right coronary artery and course across the RVOT, near or at the location of a planned transannular incision, requiring a change in the surgical plan [53].
Arterial pressure monitoring. The presence of an aberrant subclavian artery, a relatively common associated lesion, should be considered in the selection of sites for arterial line placement. In this setting, insertion and manipulation of a TEE probe during complete repair may compress the retroesophageal vessel leading to blunting or disappearance of the arterial pressure tracing. When palliation in the form of a modified Blalock-Taussig shunt is anticipated, the arterial catheter is best placed in a vessel other than the one in which the vascular graft is planned to allow for uninterrupted assessment of arterial blood pressure throughout the case.
Arch laterality. During palliative surgery via thoracotomy, Ao arch sidedness can influence the site of shunt placement and, hence, the surgical approach (right versus left thoracotomy). Although Ao arch anatomy is determined preoperatively, it is a matter of consideration during surgical positioning.
Effects of surgery. It is important to highlight the impact of the surgical intervention on the physiology and influence on perioperative care as follows:
Transannular incision and patch in the definitive surgical repair of TOF invariably lead to pulmonary regurgitation because the intervention violates the integrity of the annulus and valve. In some cases, the relatively “stiff” RV limits the regurgitant volume, and this restrictive physiology is well tolerated initially [54]. However, in other infants, the acute RV volume load exacerbates an underlying element of diastolic dysfunction. This issue, compounded by some degree of systolic impairment related to myocardial edema, ischemic time, mechanical effects of the manipulations of the heart, and injury to the anterior RV wall resulting from the ventriculotomy/transannular patch, can complicate the postoperative course. The consequence is a low cardiac output state manifested in the first 24 h postoperatively. In patients with severe low cardiac output syndrome, spontaneous or negative pressure pulmonary ventilation may improve cardiac output and cerebral oxygenation [55, 56].
After complete repair, RV function can be impaired as previously described, and inotropic support may be required. However, it should be emphasized that inotropic agents can influence the postoperative assessment of the adequacy of the repair, because tachycardia and increased inotropy can exacerbate any residual RVOT gradient.
Pathology such as residual VSDs or RVOT obstruction, or significant tricuspid regurgitation may not be well tolerated in this particular patient group.
An intentional atrial communication, in the form of a patent foramen ovale, might be created during corrective surgery to facilitate postoperative care in anticipation of decreased RV compliance. This communication allows for maintenance of cardiac output at the expense of a small amount of right-to-left shunting at the atrial level and a mild degree of hypoxemia.
Junctional ectopic tachycardia. This rhythm disturbance can occur in the immediate postoperative period following corrective interventions in children, including those with TOF repair [57]. The arrhythmia is characterized by a narrow QRS tachycardia (heart rate greater than 170 beats per minute), atrioventricular dissociation, and a ventricular rate faster than the atrial rate. The loss of the atrial contribution to ventricular filling and shortened diastolic filling time can have significant hemodynamic effects in this vulnerable patient group. Strategies applied in the management of this arrhythmia include sedation (to reduce sympathetic tone), decrease in the level of inotropic support, surface cooling, correction of electrolyte disturbances, various forms of pacing, and administration of magnesium. Pharmacological treatment with intravenous amiodarone or procainamide may be indicated in some cases [58]. In the extremely unstable neonate, circulatory support may be necessary. Pretreatment with a beta-blocker may reduce the incidence of JET [59].
Total Anomalous Pulmonary Venous Return
Anatomic Features
Total anomalous pulmonary venous return (TAPVR) accounts for 2 % of all CHD. It is characterized by drainage of all pulmonary venous blood back into the pulmonary circulation through remnants of vascular connections normally present during fetal life. The lesion represents an obligatory shunt as oxygenated blood from the pulmonary veins is diverted to the RA. This anomaly is classified into several types according to the main pathway of drainage of the anomalous pulmonary veins (Fig. 12.7): supracardiac, via a vertical vein to innominate vein or right SVC; cardiac, into the coronary sinus or RA; infracardiac, via a common pulmonary vein into the portal system, from there through the ductus venosus to the IVC; or mixed, a combination of the various types. In two-thirds of the cases, it is an isolated lesion. An atrial communication and PDA almost always are present. The remaining one-third of cases is associated with other defects (VSD, CoA, and complex CHD such as heterotaxy syndromes).


Fig. 12.7
Graphic representation of total anomalous pulmonary venous return. Panel a, supracardiac drainage. In this lesion the path of pulmonary venous drainage is from a vertical vein to the innominate vein into the right atrium (RA). In most defects an associated atrial septal defect is present. Panel b, cardiac drainage. In this pathology, the pulmonary veins (PVs) typically empty into the coronary sinus, draining into the RA. Panel c, infracardiac drainage. In this setting, PVs drain through the ductus venosus to the RA. Frequently this anomaly is associated with pulmonary venous obstruction. IVC inferior vena cava, LA left atrium, LV left ventricle, RV right ventricle, SVC superior vena cava
Pathophysiology
Anomalous pulmonary venous return is well tolerated in the fetus because of the limited pulmonary blood flow associated with the high pulmonary vascular resistance. In the first few days/weeks of life, the presence or absence of obstruction along the pulmonary venous pathway plays a major role in the physiologic consequences of the defect. In the absence of pulmonary venous obstruction and high pulmonary blood flow, a nonrestrictive ASD is associated with a relatively high systemic arterial oxygen saturation and adequate systemic cardiac output. Over time, however, RV volume overload and congestive symptoms ensue. This physiology can result in a late presentation. In contrast, a restrictive atrial communication leads to a greater degree of arterial hypoxemia and compromised systemic output. Pulmonary venous obstruction almost always is the norm in infradiaphragmatic TAPVR related to constriction of the ductus venosus. This condition is characterized by profound hypoxemia caused by decreased pulmonary blood flow and pulmonary venous congestion, pulmonary hypertension, and respiratory compromise. Relative “hypoplasia” of left-sided cardiac structures can be seen in the affected neonate with TAPVR.
All forms of this lesion have complete mixing of systemic and pulmonary venous returns at the level of the RA. Right-to-left atrial shunting allows mixed blood to enter the LA, which is then ejected by the LV into the systemic circulation. The degree of hypoxemia depends on the severity of the pulmonary venous obstruction; it can be mild, mimicking, respiratory distress syndrome, or profound, in the case of a moribund infant with obstructed pulmonary venous drainage.
Management
Initial efforts are directed at stabilization of the neonate and respiratory/hemodynamic support as necessary. Surgical intervention can be undertaken on an elective basis if minimal to no pulmonary venous obstruction is present and the infant is doing well clinically. Most cases with obstruction require urgent surgery. The neonate with obstructed pulmonary venous return represents an emergency because profound hypoxemia generally is the case. Some providers consider the administration of PGE1 potentially detrimental in the presence of severe obstruction as it further increases pulmonary blood flow, aggravating the obstruction and worsening oxygenation. Others regard PGE1 beneficial as it influences vascular smooth muscle tone and may maintain patency of the ductus venosus, relieving the obstruction. Regardless of the details of the anatomy in TAPVR, the surgical correction aims at rerouting the drainage of the anomalous pulmonary veins to the LA.
Anesthetic Considerations
The main issues regarding anesthetic care in these neonates before the pathology is addressed center around respiratory and hemodynamic support. In the presence of pulmonary venous obstruction and severe hypoxemia, relatively high ventilatory settings that include the use of high peak inspiratory and positive end-expiratory pressures usually are required. The repair is performed frequently under deep hypothermic circulatory arrest (DHCA) or using low-flow perfusion. Upon separation from CPB, support of the RV with inotropic agents and pulmonary vasodilators is important in order to maintain forward flow in the face of potentially increased ventricular afterload resulting from an elevated pulmonary vascular resistance. This management aims at limiting the likelihood of negative ventricular interactions. In addition, the LV in this lesion is relatively noncompliant and subject to failure, with increases in blood volume resulting in reductions in stroke volume. Thus, the administration of fluid deserves caution to prevent LV wall overdistension.
Specific Issues
Pulmonary vascular reactivity. There is a propensity in these infants to develop acute pulmonary hypertensive crises in the post-bypass or postoperative period due to a reactive pulmonary vascular bed. These episodes are prevented/managed by deep sedation, measures to decrease pulmonary vascular tone, and the administration of pulmonary vasodilators, including inhaled nitric oxide, as required. In cases at risk, PA pressure monitoring via a direct transthoracic catheter facilitates management.
Partial anomalous pulmonary venous drainage. Defects that include anomalous drainage of only a few pulmonary veins are not uncommon in association with other forms of CHD, but usually this is of little physiological consequence during the neonatal period.
Truncus Arteriosus
Anatomic Features
Truncus arteriosus (TA) is a relatively uncommon malformation, representing only 1 to 2 % of congenital cardiac defects. Approximately one-third of infants with this anomaly have deletions of chromosome 22 (e.g., DiGeorge syndrome). In TA, also referred to as persistent truncus arteriosus or common arterial trunk, a single arterial root emerges from the heart, giving rise to the Asc Ao, PA, and coronary arteries (Fig. 12.8). An unrestrictive outlet, VSD, almost always is present, and the origin of the common arterial trunk characteristically straddles the defect. This defect is thought to be caused by failure of the normal process of conotruncal septation that results in two great arteries.


Fig. 12.8
Graphic representation of truncus arteriosus demonstrating the common truncal root with a biventricular origin. In this lesion, there is obligatory mixing of the systemic and pulmonary venous returns. Ao aorta, IVC inferior vena cava, LA left atrium, LV left ventricle, PA pulmonary artery, RA right atrium, RV right ventricle, SVC superior vena cava
TA is subdivided into several subtypes (types I, II, III) based on the anatomical origin of the pulmonary arteries from the truncal vessel as described by Collett and Edwards [60]. The most common variant is one somewhere between types I and II, colloquially referred to as truncus arteriosus type 1½. Associated lesions include truncal valve issues (abnormal number of cusps, dysplasia, stenosis, regurgitation), abnormal origin and course of the coronary arteries, and Ao arch anomalies (right Ao arch and Ao arch interruption).
Pathophysiology
Hypoxemia associated with this anomaly is due to complete admixture of systemic and pulmonary venous blood as it emerges from both ventricles into the single arterial vessel. The hemodynamic repercussions of the defect relate to the direct physical communication between the systemic and pulmonary circulations at the level of the truncal root. This arrangement imposes a pressure and volume load to both ventricles further exacerbated by truncal valve pathology (stenosis or regurgitation), if present. The clinical features depend largely on two factors: the pulmonary vascular resistance and the presence of any intrinsic stenosis in the pulmonary arteries. These variables determine the volume of blood that enters the pulmonary vascular bed from the truncal root.
The neonate usually is well compensated immediately after birth due to the normal relatively high pulmonary vascular resistance. As the resistance decreases, symptoms related to pulmonary over-circulation and congestive heart failure ensue. In the presence of branched PA stenosis, the neonate can remain clinically stable to the degree that pulmonary blood flow is adequately restricted. If the obstruction is severe, cyanosis can be significant.
Management
Anticongestive therapy is indicated preoperatively in most cases and in some instances, inotropes. The current preferred approach is surgical repair during the neonatal period consisting of removal of the PA(s) from the truncal root, repair of the ensuing defect, patch closure of the VSD, RVOT reconstruction, and repair of associated pathology. Early correction is undertaken in view of the potential for rapid development of pulmonary vascular obstructive disease in this lesion.
Anesthetic Considerations
A major challenge in the perioperative care of the neonate with TA pertains to balancing the pulmonary and systemic vascular resistances to achieve cardiovascular stability during the pre-bypass period. A low pulmonary vascular resistance results in hemodynamic compromise because pulmonary over-circulation is associated with a steal phenomenon characterized by high systemic arterial oxygen saturation, low diastolic arterial pressures, and a wide pulse pressure. This runoff condition leads to impaired systemic output, hypotension, compromised oxygen transport caused by hypoperfusion of distal beds, and potential for myocardial ischemia, implying a high likelihood for morbidity and critical events. In fact, this defect is considered one of the congenital lesions associated with a very high risk of adverse perioperative events [61].
The anesthetic management of the neonate with unrepaired TA therefore focuses largely on controlling pulmonary blood flow and maintaining adequate systemic output. Increasing pulmonary vascular resistance by decreasing the inspired oxygen concentration, if possible to near room air, and increasing arterial carbon dioxide tension (PaCO2) are the most common strategies for limiting pulmonary blood flow. Due to anesthetic effects, positive pressure ventilation, and changes in intravascular volume, managing pulmonary over-circulation during the pre-bypass period can be difficult and systemic arterial blood pressure can decrease. In fact, electrocardiographic changes associated with myocardial ischemia may occur. Care should be taken in the administration of agents that depress the myocardium. Potential strategies to address hypotension include volume administration, increasing the hematocrit level, and the use of inotropes for ventricular support/vasoconstrictors as required. During cardiac surgery, a temporary snare can be placed around one of the PA branches to mechanically restrict pulmonary blood flow and augment systemic output. As would be expected, this approach is associated with systemic arterial desaturation, and frequently the inspired oxygen concentration needs to be increased.
Specific Issues
Residual lesions. After surgery, volume loading the LV, as imposed by a residual VSD or truncal valve regurgitation, may not be well tolerated. The post-repair TEE examination plays a major role in this assessment as well as being able to exclude hemodynamically significant truncal valve stenosis and RVOT obstruction, to interrogate for atrioventricular valve regurgitation, and to evaluate ventricular function.
Pulmonary hypertension. Affected neonates are particularly vulnerable to the development of acute increases in PA pressures during the post-bypass period and in the intensive care unit. This vulnerability can lead to acute decompensation, significant morbidity, and even death. The perioperative management of pulmonary hypertensive crises is addressed in subsequent sections of this chapter (refer to page x).
Critical Aortic Stenosis
Anatomic Features
Congenital AS is present in 3 to 6 % of all patients with CHD; however, symptomatic disease in the neonate or infant accounts for fewer than 10 % of cases of Ao valve stenosis consistent with the rare nature of the lesion. The valve in critical AS is characterized by leaflet fusion, frequently exhibiting a unicuspid appearance, or might be severely thickened and dysplastic (Fig. 12.9). The Ao annulus, root, and Asc Ao typically display some element of hypoplasia. In many cases, LV dilation in combination with poor function and mitral regurgitation is present. This condition is frequently associated with atrophy and infarction of papillary muscles as well as endocardial fibroelastosis (EFE). Various degrees of LV hypoplasia and mitral and Ao arch hypoplasia also can be present in some infants.


Fig. 12.9
Graphic representation of critical aortic stenosis. Ao aorta, IVC inferior vena cava, LA left atrium, LV left ventricle, PA pulmonary artery, RA right atrium, RV right ventricle, SVC superior vena cava
Pathophysiology
Obstruction to LV ejection due to a restricted aortic valvar orifice leads to increased LV systolic pressure, a transvalvular pressure gradient, increased myocardial force, and LV wall stress. The thickened myocardium is at risk for developing subendocardial ischemia as a consequence of an imbalance in the ratio of myocardial oxygen supply and demand. LV EFE results from compromised subendocardial oxygen delivery secondary to myocardial ischemia. Fibrotic myocardial changes lead to severe ventricular functional impairment and associated marked increases in LV end-diastolic and LA pressures. The presence of EFE along with LV dilation/dysfunction carries a poor prognosis. A large gradient across the Ao valve places a severe stress on systemic function of the LV.
Critical AS in the fetus can lead to hydrops caused by ongoing subendocardial ischemia and severe myocardial dysfunction. In most cases of critical disease in the neonate, antegrade flow through the Ao is inadequate to maintain cardiac output, and right-to-left shunting through the ductus arteriosus accounts for most of the systemic blood flow. In fact, retrograde flow through the arch via the ductus may in some cases be responsible for coronary and cerebral perfusion.
The neonate can exhibit signs and symptoms of severe heart failure or frank shock, usually related to ductal constriction or closure. Clinical evidence of congestive heart failure in the neonate includes tachypnea, poor feeding, diaphoresis with feeds, failure to thrive, hepatomegaly, and a gallop rhythm. A low cardiac output state is associated with severe ventricular dilation and dysfunction characterized by poor peripheral perfusion, paleness, cool extremities, and lactic acidemia. Papillary muscle infarction can be part of a grim presentation. In the neonate, systemic compensatory responses consist of systemic vasoconstriction, increased blood volume, and increased heart rate. The physiologic alterations are complex and impact not only the cardiovascular system but also other major organs.
Management
Fetal congestive heart failure and hydrops present a unique challenge. Transplacental digitalization has been used to manage fetal heart failure. In recent years, fetal interventions for critical AS have been attempted at specialized centers in hopes of altering the natural history of the condition in utero and potentially obviating progression of the disease [62]. Critical AS without intervention carries a high mortality rate in the neonate. PGE1 therapy maintains systemic perfusion and is life saving. Concomitant therapy in the critically ill neonate consists of mechanical ventilatory support to reduce the work of breathing, diuretic therapy, and infusion of inotropic agents to augment systemic output as needed.
Options for management include percutaneous balloon valvuloplasty and surgery. In some cases, catheter interventions are favored; however, this is controversial. If surgery is undertaken, the approach is influenced by factors such as size of the Ao annulus, root, subaortic area, adequacy of the mitral valve and LV, and coexisting defects. Options include aortic valvotomy (open/closed), commissurotomy, aortic homograft placement, Ross procedure, Ross-Konno operation or other root-enlargement procedures, Damus-Kaye operation, and Norwood palliation. In some infants, cardiac transplantation may be the only feasible option. Mechanical circulatory support may be considered at several points along the care of the affected neonate as a bridge to an intervention, for postoperative failure, or while awaiting cardiac transplantation.
Anesthetic Considerations
The care of the neonate with critical AS can be extremely challenging. Concerns relate to anesthesia as well as the procedure itself. Hemodynamic decompensation can occur during induction of anesthesia or at any time during either a catheter or surgical intervention. Even the most careful induction can result in cardiovascular instability because sedatives/anesthetic agents expectedly decrease sympathetic tone, which may represent the main compensatory mechanism in the neonate. Cardiac massage may not be effective because of the restrictive Ao valve orifice; therefore, one should recognize the potential for untoward events and proceed extremely cautiously. Having immediate access to emergency drugs and defibrillation therapy is vital. In addition, these cases require advanced discussions regarding the potential need for urgent circulatory support during either the catheter-based intervention or the pre-bypass phase.
Regardless of the management or stage of a given intervention, it is important to optimize ventricular filling and function without overlooking the potential detrimental effects of volume overload in the failing heart or the increased myocardial work and decreased ventricular filling times associated with the use of inotropic drugs. The potential myocardial depressant effects of drugs, anesthetic agents, and other perioperative interventions must be carefully considered.
Specific Issues
Transcatheter interventions. Complication rates for transcatheter procedures are greater in the neonate. Potential problems include bleeding, ventricular arrhythmias, transient myocardial ischemia during balloon inflation, development of or increase in the severity of aortic regurgitation, and arterial compromise due to vascular access.
Post-bypass problems. In the post-bypass setting, major issues include residual systemic outflow obstruction, aortic regurgitation resulting from or exacerbated by the intervention, hemodynamic perturbations due to associated defects, and myocardial dysfunction.
Hypoplastic Left Heart Syndrome
Anatomic Features
It is estimated that hypoplastic left heart syndrome (HLHS) accounts for 2 % of CHD. The morphologic features of this malformation include aortic atresia or stenosis, mitral atresia or stenosis, Ao arch hypoplasia, CoA, PDA, and an atrial level communication (Fig. 12.10). Although HLHS is considered a spectrum of disease and the degree of LV hypoplasia varies, the LV in the classic lesion (aortic and mitral atresia) is significantly underdeveloped and the Asc Ao is markedly hypoplastic [63].


Fig. 12.10
Graphic representation of hypoplastic left heart syndrome. Note the small left-sided cardiac structures and direction of flow across the ductus arteriosus (right-to-left shunting). The ductus allows for antegrade blood flow into the descending aorta (Ao) and retrograde flow around the aortic arch. An atrial communication allows for unobstructed egress of left atrial blood. ASD, atrial septal defect, IVC inferior vena cava, LA left atrium, LV left ventricle, PA pulmonary artery, PDA patent ductus arteriosus, RA right atrium, RV right ventricle, SVC superior vena cava
Physiology
The diagnosis of HLHS usually is made either in utero, immediately or very soon after birth. Most affected neonates are born at term and appear normal at birth. Because right-to-left shunting across the ductus arteriosus provides for the entire systemic blood flow in the case of aortic atresia, it is considered a “ductal-dependent circulation.” The atrial communication allows for egress of pulmonary venous return into the RA, where it mixes with the systemic venous blood entering the RV and MPA.
The clinical presentation in HLHS varies; some infants display cyanosis and/or features of pulmonary venous congestion and others present with ominous signs of low cardiac output and impending or frank cardiovascular collapse. The acute decompensation is associated with ductal constriction and hypoperfusion of systemic vascular beds, resulting in metabolic acidosis and lactic acidemia. The shunted blood across the ductus arteriosus in most cases not only serves as the entire source of systemic cardiac output but also provides coronary blood flow because there is absent (aortic atresia) or virtually no antegrade flow through the Ao valve (severe AS). An aspect of the pathology that influences the clinical presentation is the degree of severe restriction across the interatrial communication or in some patients, an intact atrial septum. On occasion, a decompressing vein can be present, allowing for the egress of LA blood elsewhere in the circulation. If this is not the case, the critically ill neonate displays profound hypoxemia and acidosis requiring immediate attention. This condition represents a major clinical problem associated with a poor outcome [64].
Management
The initial management of HLHS includes optimization of the clinical status of the neonate and maintenance of ductal patency with an infusion of PGE1 [65]. Mechanical ventilation, correction of acid–base status, and inotropic support may be necessary. Strategies to manipulate the ratio of pulmonary to systemic blood flow (Qp/Qs) and to optimize oxygen delivery are the mainstay of management (Fig. 12.11) [66]. Commonly, steps are taken to maintain a relatively high pulmonary vascular resistance, thereby limiting pulmonary blood flow and increasing systemic blood flow. In most cases, maintaining normocarbia or allowing mild hypercarbia, and/or limiting the fraction of inspired oxygen concentration, can achieve this goal. The administration of nitrogen to deliver a hypoxic gas mixture also has been used as has carbon dioxide in an effort to increase pulmonary vascular tone and balance the Qp/Qs [67, 68]. In a study that assessed the effects of inspired gas mixtures to reduce pulmonary over-circulation and improve systemic perfusion, inspired carbon dioxide (3 %) improved cerebral oxygenation and mean arterial pressure, whereas a hypoxic mixture (17 % inspired oxygen) affected neither [69]. The neonate with a high PaO2 caused by pulmonary over-circulation may have inadequate systemic blood flow. This condition will be associated with severe metabolic acidosis and with problems secondary to decreased perfusion of metabolically active organs (e.g., necrotizing enterocolitis resulting from impaired splanchnic blood flow).


Fig. 12.11
Physiologic balance between the systemic and pulmonary circulations required for hemodynamic stability in the infant with hypoplastic left heart syndrome prior to and following palliation. Reproduced with permission from Hansen DD, Hickey PR. Anesthesia for hypoplastic left heart syndrome: use of high-dose fentanyl in 30 neonates. Anesth Analg 1986,65:127–132. PBF pulmonary blood flow, PVR pulmonary vascular resistance, SBF systemic blood flow, SVR systemic vascular resistance
Various approaches have been utilized in the management of this lesion and reflect the ongoing challenges in the care of these infants even today [70]. Options include comfort care and no intervention, in which case death is almost assured; a stepwise palliative surgical strategy; an initial combined catheter-surgical approach (hybrid procedure) and subsequent step palliation; and cardiac transplantation [71, 72].
The initial surgical strategy for HLHS during the neonatal period is referred to as Stage I palliation or the Norwood procedure. It involves neoaortic reconstruction using the native pulmonary root and homograft material to relieve the systemic outflow tract obstruction, an atrial septectomy to allow for unobstructed drainage of the pulmonary venous return into the RA, and creation of a reliable source of pulmonary blood flow through either a modified Blalock-Taussig shunt (Fig. 12.12) or an RV to PA conduit (Sano modification; Fig. 12.13) [73]. The RV becomes the chamber that supports both circulations at this stage of surgical palliation. Complications after this operation are associated with significant morbidity and risk of mortality [74].



Fig. 12.12
Graphic representation of the Norwood procedure with a systemic to pulmonary artery shunt (modified Blalock-Taussig shunt)

Fig. 12.13
Graphic representation of the Sano modification of the Norwood procedure with a right ventricular to pulmonary artery conduit. Ao aorta, IVC inferior vena cava, LA left atrium, LPA left pulmonary artery, LV, left ventricle, PDA, patent ductus arterious, RA, right atrium, RPA right pulmonary artery, RV right ventricle, SVC superior vena cava
The hybrid procedure for HLHS represents an alternate palliative option to Stage I reconstruction during the neonatal period (Fig. 12.14) [72]. This approach involves a combined catheter-based and surgical strategy wherein a stent is deployed by the interventionalist across the ductus arteriosus to maintain patency and bilateral bands are placed across the PA branches by the surgeon to restrict pulmonary blood flow. Enlargement of the interatrial communication (via balloon atrial septostomy) usually is performed during or within a few days after this procedure. The hybrid approach delays Ao reconstruction until later in infancy and is considered of potential benefit, as the fragile neonate is not subject to CPB or associated techniques such as DHCA. In these infants, the Norwood reconstruction is subsequently combined with the creation of a superior cavopulmonary connection or Glenn anastomosis (Stage II palliation), thus effectively merging the first two stages of the palliative pathway. Depending on institutional preference, the hybrid approach is either reserved for high-risk neonates with HLHS or used liberally as the favored strategy.


Fig. 12.14
Graphic displaying hybrid palliation for hypoplastic left heart syndrome. The approach consists of ductal stenting, banding of both branch pulmonary arteries, and enlargement of an interatrial communication
Anesthetic Considerations
As initial interventions in HLHS are very high risk, it is essential to procure absolutely reliable vascular access and ensure excellent function of all monitors at the outset of the procedure. In the operating room, the same management principles discussed previously to maintain systemic output, oxygen delivery, and balance of the pulmonary and systemic blood flows are followed. Partial occlusion of a branch PA by the surgeon to mechanically limit pulmonary blood flow also can be of benefit in this setting. The anesthetic technique varies among centers; in some, a high-opioid-muscle relaxant technique is preferred, whereas in others the use of opioid is more limited and volatile anesthetic is favored.
The use of an alpha-adrenergic blocking agent (phentolamine) has been advocated as a means to optimize peripheral vasodilation during the cooling phase of CPB. Long-acting blockade with phenoxybenzamine has been shown to improve systemic oxygen delivery [75]. This is secondary to minimizing regional variations in SaO2-induced vascular resistance, thereby allowing the use of greater oxygen concentrations to improve systemic oxygen delivery in the postoperative period [76].
Specific Issues
Surgical palliative approach. The particular surgical approach for Stage I palliation (systemic to PA shunt or RV to PA conduit), as well as the favored perfusion strategy (circulatory arrest or antegrade cerebral perfusion), remains dependent on the surgeon and the center where the procedure is performed [77]. These factors impact the selection of arterial blood pressure-monitoring sites and may favor the use of additional monitors, including those that allow for monitoring of the brain. In some patients special bypass strategies may be used (refer to page x), and in these cases, additional monitoring may be required (refer to monitoring section, page x). Whereas a modified Blalock-Taussig shunt allows for pulmonary blood flow to occur during the entire cardiac cycle, in a Sano connection, most of this flow occurs during systole. The narrower pulse pressure and relatively greater diastolic blood pressure allowed by the Sano conduit as compared with the modified Blalock-Taussig shunt is regarded as an advantage for end-organ and coronary perfusion. The Sano strategy is preferred at some centers in order to improve the immediate postoperative course. This relatively more stable circulation is also thought to potentially limit the rates of interstage mortality that occurs in these infants while they await a second palliative procedure [78]. In a study that included a large number of infants with HLHS undergoing the Norwood procedure, the transplantation-free survival rate at a year post-operation was better with the RV to PA shunt than with the modified Blalock-Taussig shunt. After that time period, no significant difference in transplantation-free survival was evident between the two groups [77].
Right ventricular optimization. With the RV operating as the systemic pump in HLHS, all efforts to maintain/enhance RV function during the perioperative period should be considered.
Balancing the circulations. After separation from CPB, care is taken to optimize the balance between the pulmonary and systemic blood flows. A conventional management strategy has been to monitor the arterial oxygen saturation for this assessment and to guide this balance by targeting a value between 75 and 80 %. If the arterial oxygen saturation is less than expected after separation from CPB and potential factors such as inappropriate shunt size and shunt occlusion/distortion have been excluded, steps are undertaken to decrease the pulmonary vascular resistance and increase the systemic arterial blood pressure in an attempt to improve the pulmonary blood flow. A relatively high hemoglobin concentration is important in this setting to enhance the delivery of oxygen and to prevent anemia-related low peripheral vascular tone. At the same time, the deleterious effects of overtransfusion and polycythemia should be considered, particularly in view of the potential negative impact on blood flow across the systemic to PA shunt and patency of this connection. If maneuvers that decrease the pulmonary vascular tone are unsuccessful, administration of inhaled nitric oxide as a selective pulmonary vasodilator should be considered. If the arterial oxygen saturation is greater than expected, it is reasonable to reduce the inspired oxygen concentration and ensure normocarbia or mild hypercarbia. If there is evidence of adequate systemic output and tissue perfusion, a relatively high arterial oxygen saturation may be acceptable.
Mixed venous oxygen saturation monitoring. An approach proposed in the neonate with single ventricle physiology following Stage I palliation to ensure systemic oxygenation is the use of continuous mixed venous oxygen saturation (SvO2) monitoring, using transthoracic catheters with oximetric capabilities placed directly by the surgeon at the time of surgery [78]. A reported strategy is to target an SvO2 value over 50 %, a mean arterial pressure over 45 mmHg, normocarbia, and to administer oxygen as required to maintain the SpO2. Relying on SvO2 as an indicator of systemic oxygen delivery and an SvO2-directed strategy has been associated with favorable outcomes in neonates with HLHS [79, 80].
Postoperative issues. Regardless of the surgical technique, patients with HLHS present major challenges of bleeding, myocardial dysfunction, and hemodynamic instability during the post-bypass period. Other potential problems include renal dysfunction and hepatic impairment [81]. As a result of these concerns, sternal closure may be delayed and some may need postoperative mechanical circulatory support. Routine use of circulatory support immediately after the Norwood procedure to facilitate postoperative management has been reported; however, this is not the standard of care at most centers [82].
Hybrid procedure. Deferring the risks associated with the Norwood operation to a later time in infancy, as allowed by a hybrid procedure, can offer potential advantages to the neonate. Although caring for a neonate with a critical lesion, such as HLHS, outside the typical surgical setting can present major challenges, a report on the anesthetic management of neonates undergoing the hybrid procedure documented relatively stable intraoperative and early postoperative hemodynamics [83]. In addition, this experience indicated that most neonates did not require blood transfusions or inotropic support and performing endotracheal extubation was feasible either at the end of the procedure or soon after the infant was admitted to intensive care.
Interrupted Aortic Arch
Anatomic Features
Interrupted aortic arch (IAA), a discontinuity of the Ao arch, is an uncommon lesion representing only 1 % of all CHD. Affected children have a high incidence of DiGeorge syndrome (22q11 microdeletion).
This anomaly is defined in terms of the site of interruption as follows: type A, if distal to the left subclavian artery; type B, if between the left carotid and left subclavian arteries (most common variant; Fig. 12.15); and type C, if between the carotid arteries. The pathology frequently is associated with a posteriorly malaligned VSD, resulting in obstruction of the LVOT. Coexisting anomalies include a right Ao arch, aberrant origin of a subclavian artery, truncus arteriosus, and aortopulmonary window.


Fig. 12.15
Graphic representation of type B interrupted aortic arch. Note the site of interruption between the left carotid and left subclavian arteries. The patent ductus arteriosus (PDA) supplies the systemic circulation beyond the level of interruption. Ao aorta, IVC inferior vena cava, LA left atrium, LV left ventricle, PA pulmonary artery, RA right atrium, RV right ventricle
Pathophysiology
Patency of the ductus arteriosus is essential for survival in this anomaly, as it allows for perfusion of systemic beds distal to the interruption. The presentation of IAA in the neonate is characterized by congestive heart failure, poor perfusion, cardiovascular collapse/shock as the ductus arteriosus closes, and, occasionally, differential cyanosis. In this regard, the physiology resembles that of severe CoA. The presence of a VSD can cause pulmonary over-circulation and the associated consequences.
Management
After the diagnosis is established, stabilization of the infant is critical; PGE1 therapy should be initiated to maintain ductal patency. Anticongestive therapy and inotropes should be administered as needed. Surgery is undertaken in the neonatal period, typically soon after the diagnosis is made. Aortic arch reconstruction, closure of the VSD, and possible resection of subaortic obstruction are performed. Much less commonly, an initial palliative approach is undertaken with Ao arch repair and pulmonary artery banding (PAB), followed by delayed complete repair later in infancy. If the LVOT obstruction is severe (marked subaortic narrowing, annular/Ao root/Asc Ao hypoplasia), alternate approaches, including Ao root-enlargement, replacement, or other complex interventions, may be necessary. A single ventricle strategy is required in some cases.
Anesthetic Considerations
Although echocardiography is diagnostic in most neonates with IAA, additional preoperative studies may be needed to further define the details of the arch anatomy. These examinations in most cases require care of the neonate at remote locations, adding to the management considerations for the sick infant.
Maintenance of the PGE1 infusion is critical before reconstructing the Ao arch. An adequate response generally implies no significant pressure gradient between proximal and distal areas of the interruption. In view of the common association between PGE1 therapy and apnea, and other clinical issues, these neonates frequently are intubated and mechanically ventilated in the critical care unit.
Specific Issues
Monitoring. Selection of the sites for monitoring systemic arterial blood pressure and pulse oximetry is an important consideration in IAA. It is dictated by the Ao arch anatomy and the presence of coexistent anomalies. In the case of a type B interruption with left Ao arch and aberrant right subclavian artery, for example, none of the vessels that supply the limbs are proximal to the interruption site. Thus, the arterial blood pressure proximal to the site of interruption cannot be measured in any extremity. This issue can have implications for the surgical intervention, as the perfusion pressure cannot be recorded while on CPB during the period of Ao arch reconstruction. Neuromonitoring can be reassuring in this setting. With regard to pulse oximetry, an oxygen saturation differential would be expected in the unrepaired neonate, with greater values in the beds supplied proximal to the site of interruption by the Asc Ao and reduced values distally reflecting flow from the ductus arteriosus.
Concerns related to DiGeorge syndrome. In view of the potential for developing hypocalcemia in neonates with DiGeorge syndrome, calcium levels should be measured frequently. In such cases, calcium infusions may be required. The presence of coexisting noncardiac anomalies in this syndrome must be considered including immune deficiency. Irradiated blood products should be used to prevent potentially fatal transfusion-associated graft-versus-host disease.
Surgical considerations. Interventions for IAA can be quite complicated, requiring considerable bypass and myocardial ischemic times, particularly when concomitant LVOT obstruction is present. This can be a very challenging condition to treat.
Critical Pulmonary Stenosis and Pulmonary Atresia with Intact Ventricular Septum
Anatomic Features
Pulmonary stenosis is the most common form of RVOT obstruction among infants, accounting for more than 80 % of the cases. This lesion is reported in approximately 10 % of patients with CHD. In the classic isolated pathology, leaflet tethering/thickening and commissural fusion lead to the formation of peripheral raphes and narrowing of the valve lumen. Systolic valvar doming is usually identified on cardiac imaging.
Critical PS (Fig. 12.16) and pulmonary atresia with intact ventricular septum (Fig. 12.17) represent lesions characterized by pulmonary valve/RVOT obstruction. The pulmonary valve in the neonate with critical PS, the most severe form of valvar obstruction in infancy, displays fused raphes with a restrictive, eccentric pin-size opening. In the uncomplicated or pure form of critical PS, the ventricular septum is intact and an interatrial communication (patent foramen ovale or secundum atrial septal defect) is present. Pulmonary atresia with intact ventricular septum is a lesion characterized by membranous or muscular atresia of the RVOT and wide diversity in the size of the RV cavity, infundibular region, and pulmonary arteries. This defect is the third most common cyanotic congenital pathology in the neonate and accounts for approximately 3 to 4 % of all congenital lesions diagnosed in infancy [84].



Fig. 12.16
Graphic representation of critical pulmonary stenosis. In this defect, the ductus arteriosus serves as the main source of pulmonary blood flow. Ao aorta, IVC inferior vena cava, LA left atrium, LV left ventricle, PA pulmonary artery, RA right atrium, RV right ventricle, SVC superior vena cava

Fig. 12.17
Graphic representation of pulmonary atresia and intact ventricular septum. Note the associated findings in this defect that usually include a patent ductus arteriosus, hypoplastic right ventricle, atrial septal defect, and, in some cases, some degree of tricuspid regurgitation. The ductus arteriosus provides the source of pulmonary blood flow in this lesion prior to an intervention. The atrial communication allows for right-to-left shunting. Ao aorta, IVC inferior vena cava, LA left atrium, LV left ventricle, PA pulmonary artery, RA right atrium, RV right ventricle, SVC superior vena cava
The tricuspid valve, RV, and pulmonary arteries frequently display abnormalities in both of these defects (abnormal tricuspid valve leaflets, tricuspid annular hypoplasia, reduced size of the RV cavity, main and branch pulmonary arteries), but they are likely to be of a more severe nature in the neonate with pulmonary atresia and intact ventricular septum.
Pathophysiology
Cyanosis is a common presentation in both of these congenital heart anomalies. The severity of the hypoxemia is determined by the decrease in pulmonary blood flow and the extent of interatrial right-to-left shunting. Affected neonates have ductal-dependent pulmonary blood flow. The severity of the obstruction in critical PS is determined by the extent of valvar narrowing. In pulmonary atresia with intact ventricular septum, no antegrade flow is feasible across the valve, as the outflow is completely obstructed. The main physiologic consequence of these pathologies is an increase in the RV systolic pressure that can exceed systemic pressures.
In infants with critical PS, the infundibular region (subpulmonary area) participates in the hypertrophic response, aggravating the outflow obstruction and contributing to the reduction in RV cavity size. Right ventricular hypertrophy serves as a compensatory mechanism to maintain ventricular output over time. However, it is associated with diminished RV compliance and diastolic dysfunction. Subendocardial ischemia resulting in myocardial infarction and fibrosis accounts for systolic impairment, eventual chamber dilation, and congestive heart failure.
In pulmonary atresia with intact ventricular septum, unusual communications between the coronary arteries and the RV can be present, as may a number of coronary abnormalities. When this is the case, the myocardium may rely on coronary perfusion directly from the RV (RV-dependent coronary circulation) [85]. This condition is a vulnerable situation that can predispose the infant to the development of myocardial ischemia and infarction.
In both of these lesions, critical PS and pulmonary atresia with intact ventricular septum, the structural abnormalities of the tricuspid valve frequently are associated with regurgitation, particularly in the presence of RV hypertension, leading to RA dilation.
Management
The mainstay of therapy in lesions with severe RVOT obstruction is stabilization of the neonate and PGE1 therapy to provide for pulmonary blood flow. Options for management are catheter-based or surgical. Unfavorable anatomy or suboptimal results from a catheter intervention may require surgery. In infants with critical PS, the most common cardiac catheterization interventions are percutaneous balloon valvuloplasty and ductal stenting. Surgical options include pulmonary valvotomy with splitting of the commissures and/or partial valvectomy. Concomitant resection of infundibular obstruction or placement of a transannular patch may be necessary. In a minority of cases, valve replacement with an RV to PA conduit is the most suitable option. In rare instances, a systemic to PA shunt is required.
In the neonate with pulmonary atresia and intact ventricular septum, angiographic data are obtained before any intervention to determine the presence/absence of communications between the RV and coronary arteries [86]. Potential significant coronary artery abnormalities (stenosis, interruptions) also need to be characterized for planning interventional strategies. The concern when RV decompression or pulmonary valve perforation is performed is the potential for myocardial ischemia or infarction related to reductions in RV pressure in the case of RV-dependent coronary circulation. If a patent infundibulum is present and other aspects of the anatomy are favorable, radiofrequency valve perforation and balloon dilation may be considered. This procedure has been performed successfully in affected neonates, allowing antegrade flow into the pulmonary arteries [87]. In some cases, however, the amount of pulmonary blood flow is inadequate, as manifested by significant arterial desaturation upon weaning or discontinuation of PGE1 therapy, and the infant benefits from a surgical intervention to augment pulmonary blood flow (i.e., systemic to PA shunt, valvotomy). An approach that combines transventricular valvotomy with a systemic to PA shunt has been reported to promote growth of right-sided structures, increasing the likelihood of an eventual biventricular repair [88]. Ductal stenting also has been performed in these lesions. Another procedure that may be considered, depending on the size of the RV and likelihood of a future biventricular circulation, is reconstruction of the RVOT. If anatomic factors such as severely hypoplastic pulmonary arteries preclude a definitive intervention, palliation consisting of a systemic to PA shunt is performed in order to promote vessel growth. Conditions such as severe coronary obstruction, myocardial ischemia/infarction, and LV dysfunction warrant consideration for cardiac transplantation because of the likely fatal nature of this lesion.
Anesthetic Considerations
An important aspect of the care in the neonate affected by these lesions is to ensure patency of the ductus arteriosus by the continuous intravenous infusion of PGE1. Catheter-based therapy can lead to effective relief of the obstruction, but factors such as abnormal ventricular morphology/geometry, relatively small pulmonary/tricuspid annulus, hypoplasia of the pulmonary arteries, and interatrial right-to-left shunting may not allow for immediate improvement in the arterial oxygen saturation in some infants. It is not unusual for the PGE1 infusion to be continued after the procedure is completed. Hemodynamic changes that occur during catheter-based interventions aimed at relieving the RV obstruction are reasonably well tolerated, provided the ductus arteriosus remains patent and the interatrial communication is adequate to maintain LV filling, particularly during the period of balloon dilation. In infants with pulmonary atresia and intact ventricular septum, the potential coronary abnormalities leading to a predisposition for myocardial ischemia warrant monitoring for this problem. As in the case of all other neonatal cardiac interventions, adequate preparation for these cases is of outmost importance.
After surgery that involves relief of the RVOT obstruction, inotropic support should be used judiciously as it can exacerbate the dynamic RVOT gradient, complicating the assessment of the results of the repair. Additional anesthetic considerations apply depending on the planned procedure, approach, and need for CPB.
Specific Issues
Suicide right ventricle. A potential post procedure problem is that adequate relief of the valvar obstruction, either by a catheter intervention or surgery, can result in a physiology referred to as suicide right ventricle. This results as the hypertrophied infundibulum contracts vigorously and creates significant post-procedural dynamic outflow tract obstruction, in the absence of fixed obstruction. In the case of severe obstruction and associated low cardiac output, therapy with volume expansion and/or beta-blockade may be required. An important goal is to preserve RV function by avoiding significant myocardial depression or increases in RV afterload.
Circular shunt physiology. Another major problem that can occur after these procedures is a circular shunt. This condition represents a morbid state in which the presence of a large PDA (or ductal stent) or placement of a systemic to pulmonary shunt after a pulmonary valve intervention is associated with pulmonary regurgitation. This leads to retrograde shunt flow into the RV, which proceeds to the RA due to an incompetent tricuspid valve. Blood then courses across the atrial communication into the LA, LV, and Ao, thus reentering the shunt. This situation is very precarious because it may lead to significant RV volume overload and a pulmonary steal phenomenon. The unsustainable hemodynamic state requires immediate attention, frequently consisting of escalating support and/or immediate intervention, for survival.
Aortopulmonary Window
Anatomic Features
Aortopulmonary (AP) window, also known as aortopulmonary septal defect, is a rare defect, accounting for only 0.1 % of all CHD. This anomaly is characterized by a defect in the wall between the Asc Ao and the PA, creating a communication between these structures (Fig. 12.18) [89]. From an anatomic and physiologic standpoint, this defect resembles truncus arteriosus; however, unlike truncus arteriosus, two distinct semilunar valves are present. The size and location of the communication varies, and thus the defect has been classified into various types [90]. The lesion can occur in isolation but in most cases is associated with other cardiovascular malformations (PDA, intracardiac communications, TOF, double outlet right ventricle, IAA).


Fig. 12.18
Graphic representation of an aortopulmonary window demonstrating the defect that allows for left-to-right shunting between the great arteries. Ao aorta, IVC inferior vena cava, LA left atrium, LV left ventricle, PA pulmonary artery, RA right atrium, RV right ventricle, SVC superior vena cava
Pathophysiology
The magnitude of the shunt across an AP window depends on the size of the communication, PA pressures, and relative resistances of the pulmonary and systemic vascular beds. Left-to-right shunting across the defect and the increased pulmonary blood flow are associated with elevated PA pressures, left-sided volume overload, and congestive symptoms. An uncorrected communication can lead to pulmonary vascular disease relatively early in life.
Management
The preferred approach to this lesion is considered to be surgical, although successful transcatheter closure of the communication has been reported [91]. In most cases, a patch is required to obliterate the defect and associated pathology is also addressed at the time of surgery.
Anesthetic Considerations
The same anesthetic principles that apply to the management of the neonate with any large vascular communication (i.e., PDA) or any other cardiac defect for which further increases in pulmonary blood flow are detrimental and for which a balance between pulmonary and systemic blood flow should be maintained also apply in this lesion.
Ebstein Anomaly
Anatomic Features
Ebstein anomaly represents the most common congenital malformation of the tricuspid valve, but, overall, is a rare lesion accounting for 0.3 to 0.7 % of CHD. It is characterized by apical displacement of the septal and posterior leaflets of the tricuspid valve towards the RV apex and a redundant, “sail-like” anterior leaflet (Fig. 12.19). The severity of valve displacement and dysplasia varies, accounting for different degrees of tricuspid regurgitation and the diversity of clinical manifestations. The lesion results in an atrialized RV, referring to the region of the RV proximal to the abnormal tricuspid valve attachments. The distal portion of the RV represents the functional cavity. An interatrial communication is present in the majority of affected neonates, and some degree of RV dysplasia and or dysfunction is usually seen. Other potential associated defects include severe pulmonary stenosis/valve atresia and PDA. In some cases, the RV output is decreased to such an extent that it is difficult to distinguish between functional and anatomic pulmonary stenosis/atresia. An association with Wolff-Parkinson-White syndrome is well recognized.


Fig. 12.19
Graphic representation of Ebstein anomaly displaying the displaced tricuspid valve leaflets, associated tricuspid regurgitation, and right-to-left atrial level shunting. In the neonate with anatomic of functional pulmonary stenosis/atresia, a patent ductus arteriosus is the source of pulmonary blood flow. Ao aorta, IVC inferior vena cava, LA left atrium, LV left ventricle, PA pulmonary artery, RA right atrium, RV right ventricle, SVC superior vena cava
Pathophysiology
Tricuspid regurgitation in this anomaly results in increased RA pressure and right-sided volume overload. As the RA pressure increases, it exceeds LA pressure, stretching an existing interatrial communication. This process allows for right-to-left shunting and results in decreased pulmonary blood flow and clinical cyanosis. The redundant anterior tricuspid valve leaflet can cause functional obstruction to the RV. Another frequently found feature of the pathology is abnormal RV systolic function. These factors can have detrimental effects on the LV because (1) the dilated and/or dysfunctional RV can impair LV filling and (2) the abnormal interventricular septum can affect LV geometry and function (ventricular interdependence).
Ebstein anomaly represents a wide clinical spectrum that ranges from minimal or no symptomatology to intractable congestive heart failure and even death. In utero, it can result in fetal hydrops. The hemodynamic status of the neonate is influenced by factors such as the severity of tricuspid regurgitation, presence/degree of RVOT obstruction, size and function of the RV, and associated structural defects. Cyanosis caused by right-to-left atrial shunting under conditions of elevated pulmonary vascular resistance is the most common presentation. Severe tricuspid regurgitation almost invariably results in congestive heart failure; if intractable in nature, it can lead to circulatory collapse. A neonatal presentation implies a major clinical problem and generally portends a poor prognosis.
In the neonate with Ebstein anomaly, several additional cardiac problems can complicate the clinical course. Atrial arrhythmias related to atrial dilation or abnormal conduction pathways can occur, and pulmonary stenosis or atresia (either functional or anatomic) can further compromise pulmonary blood flow.
Management
Some infants require only conservative treatment and follow-up. In the symptomatic neonate, the main issues requiring intervention are congestive heart failure and hypoxemia. Diuretic therapy and inotropic support are instituted as needed. Initial hypoxemia in the neonate can improve as pulmonary vascular resistance falls, allowing for forward pulmonary blood flow. In cases of severe pulmonary stenosis or atresia, an intervention is required. Distinguishing hypoxemia related to increased pulmonary vascular resistance from that resulting from anatomic RVOT obstruction can be difficult. Hence, PGE1 therapy frequently is instituted to maintain ductal patency until the nature of the hypoxemia can be ascertained or the expected decrease in pulmonary vascular resistance occurs. Initiating other measures aimed at decreasing pulmonary vascular tone and supporting the overall critically ill neonate are warranted.
During the neonatal period, a catheter-based intervention and/or cardiac surgery may be necessary. Catheter therapy targets the relief of RV outflow obstruction and/or to increase pulmonary blood flow (pulmonary valve dilation/perforation, ductal stenting). The choice of surgical procedure is influenced by factors such as details of the anatomy, associated defects, RV size and function, and the clinical status of the neonate. Approaches range from creation of a systemic to PA shunt, tricuspid valve repair, palliative surgery anticipating a future single ventricle strategy, and cardiac transplantation. The overall success of the various surgical interventions in the neonatal period has generally been poor in the presence of severe disease.
Anesthetic Considerations
Anesthetic care, when required, is usually for cardioversion, cardiac catheterization, or surgery. The neonate with Ebstein anomaly presents a challenge to the provider of anesthetic care because of the typically poor clinical status frequently characterized by severe tricuspid regurgitation, cyanosis, congestive heart failure, lactic acidosis, and impending circulatory collapse.
Specific Issues
Respiratory status. In the sick neonate, pulmonary mechanics can be compromised by factors such as prematurity, interstitial lung edema, and lung hypoplasia. The management of mechanical ventilatory support needs consideration of these factors while balancing the need to enhance antegrade pulmonary blood flow.
Pulmonary vascular resistance. Increased RV afterload leads to right heart distention and compromises LV performance. Thus, it is important to optimize not only RV contractility but also to aggressively avoid increases in pulmonary vascular tone that will impair the function of both ventricles.
Rhythm disturbances. Numerous rhythm abnormalities have been reported in these children. Although supraventricular arrhythmias predominate, other abnormalities include atrioventricular block and ventricular arrhythmias. These rhythm disturbances usually are poorly tolerated and require aggressive therapy that may include cardioversion, the administration of antiarrhythmic drug therapy, and/or cardiac pacing.
Circular shunt. This physiology can also occur after interventions in this lesion, as described in a preceding section (refer to page x).
Preoperative Assessment of the Neonate with Congenital Heart Disease
History and Physical Examination
It is important for the anesthesiologist to perform a comprehensive preoperative evaluation to identify and anticipate factors that could influence the perioperative management in the neonate with CHD. This evaluation begins with a review of the prenatal history that includes details of the pregnancy such as maternal illnesses (e.g., diabetes, hypertension), medications, and drug use, as well as a family history. Data regarding fetal studies, if available, should be reviewed as in many cases today the diagnosis of cardiovascular disease is established “in utero.” Specific issues of interest, in addition to the details of the anatomy, include functional assessment of the cardiovascular system, presence of extracardiac abnormalities, genetic syndromes or other disorders of potential impact, as well as an impression of the overall well-being of the fetus. Relevant information to be obtained regarding the delivery includes gestational age, Apgar scores, events related to the neonatal resuscitation, and need for interventions immediately after birth. If the diagnosis of heart disease is made postnatally, details such as clinical presentation, hospital course, results of all diagnostic studies obtained to define the cardiovascular abnormalities, interventions performed, and response to these interventions should be reviewed. It is essential to gather information regarding coexistent medical problems or conditions that could potentially affect other organ systems and impact on the anesthetic management.
The physical examination should note the neonate’s weight and length. General appearance should include the level of distress, if any, presence/degree of cyanosis, and overall clinical status. Vital signs, including blood pressure measured in the upper and lower limbs and any gradients between the limbs, should be recorded. The measured SpO2 at both pre- and post-ductal levels should be noted. A careful examination of the airway and the respiratory and cardiovascular systems should be performed. Respiratory evaluation should note rate and breathing patterns, quality of the breath sounds noting the presence of rales or rhonchi, and the presence of intercostal retractions. If the infant is receiving supplemental oxygen, the inspired oxygen concentration should be recorded. If the airway is intubated, the date the current endotracheal tube (ETT) was inserted should be noted; any difficulties with blockage of the ETT identified, a recent chest radiograph should be reviewed as well as the depth of the ETT at the lips/nose. Similarly, if the lungs are mechanically ventilated, the ventilator mode and settings should be noted. The cardiac exam should include assessment of precordial activity, heart sounds, murmurs, and gallop rhythms. The presence of any existing vascular access, patency of the catheter(s), and appropriateness of catheter tip position should be recorded. The abdomen should be examined for the presence of hepatosplenomegaly. Assessment of the extremities should include examination of pulses, capillary refill, skin temperature and color, and overall perfusion.
A significant number of infants with CHD (1 out of 8) are known to have chromosomal abnormalities. Dysmorphic features or any other noncardiac anomalies that can impact anesthetic care should be noted. Medications being administered, including indications, doses, and route, need to be reviewed.
Ancillary Studies and Laboratory Data
The preoperative electrocardiogram in the neonate with heart disease should be examined to assess for evidence of chamber dilation and/or ventricular hypertrophy, rhythm disturbances, and myocardial ischemia (Fig. 12.20). A recent chest radiograph provides information regarding cardiac size and shape, chamber enlargement, and pulmonary vascularity (Fig. 12.21). In addition, it serves to document the position of the ETT tube, stomach tube, and indwelling vascular catheters. The echocardiogram and additional imaging studies as required (magnetic resonance imaging, computed chest tomography, cardiac catheterization) are instrumental in delineating the structural and functional abnormalities. All of these studies should be reviewed carefully and the findings documented.



Fig. 12.20
Preoperative electrocardiographic recording in a newborn infant with diagnosis of truncus arteriosus. Note the peaked p waves in lead II indicative with right atrial enlargement, the prominent precordial voltages suggestive of biventricular hypertrophy, and the diffuse ST-T wave changes consistent with compromised myocardial blood flow

Fig. 12.21
Radiograph in a neonate with congenital heart disease displaying massive cardiomegaly. The tip of the endotracheal tube (ETT) appears just above the level of the clavicles prompting readjustment. The nasogastric (NG) tube is the stomach and the tip of the umbilical venous catheter (UVC) is in good position
A complete blood count, electrolyte levels, blood glucose, renal/hepatic function tests, and coagulation studies (prothrombin time, partial thromboplastin times, and international normalized ratio [INR]) should be available. The most recent blood gas analysis should be reviewed to assess oxygenation, ventilation, and acid–base status. The results of any other investigations that may have been performed (head ultrasound, renal ultrasound) also should be reviewed.
Informed Consent
The preoperative visit allows the anesthesiologist an opportunity to meet the family, discuss the anesthetic plan, and address questions in preparation for the procedure. A surgical intervention in a neonate with cardiac disease may imply a substantive risk of morbidity and even mortality. In addition, the anesthetic care for cardiac surgery also entails greater risks when compared with other neonatal surgeries [61, 92]. Although it may not be possible to specify the contribution of anesthesia itself to the overall risks of the procedure, it is reasonable to discuss the most likely potential anesthetic problems that may be encountered perioperatively.
Cardiac surgery in the neonate is a major undertaking regardless of the nature of the procedure, and one must always expect the parents to be apprehensive and concerned. In some cases, particularly those with a postnatal cardiac diagnosis or if the infant had been previously discharged from a well-baby nursery, significant parental stress may be present. The preoperative consultation provides an opportunity to defuse parental anxiety, answer questions, and reassure the parents regarding the unwavering commitment of the entire perioperative team towards a good perioperative outcome.
Fasting Period
The established preoperative fasting guidelines for surgery in neonates should be followed [93]. Gastric emptying times can be prolonged in those with CHD [94]. A significant number of infants receive maintenance intravenous fluids preoperatively. Adequate hydration is particularly important in the presence of obstructive pathology, cyanotic disease, or single ventricle physiology, as optimization of ventricular preload can limit potential detrimental hemodynamic changes associated with anesthesia and surgery.
Perioperative Considerations in the Neonate Undergoing Cardiac Surgery
Anesthesia Care Provider
Anesthesia for cardiac surgery in the neonate should be delivered by highly skilled individuals with expertise in the various forms of pediatric heart disease. This requires knowledge of the wide spectrum of anatomic and physiologic abnormalities involved, the natural history of the defects, possible management strategies, and overall short- and long-term outcomes. A comprehensive understanding of the disease process, hemodynamic perturbations, and how they are influenced by the anesthetic and surgical procedure are of outmost importance. Familiarity with the anticipated perioperative course, including potential problems and complications, is essential. In addition to these cognitive skills, the treatment of small infants with critical heart disease requires the technical proficiencies that are essential for the care of small infants. The ability to communicate clearly and work effectively with other members of the team is extremely important to ensure the best possible outcome.
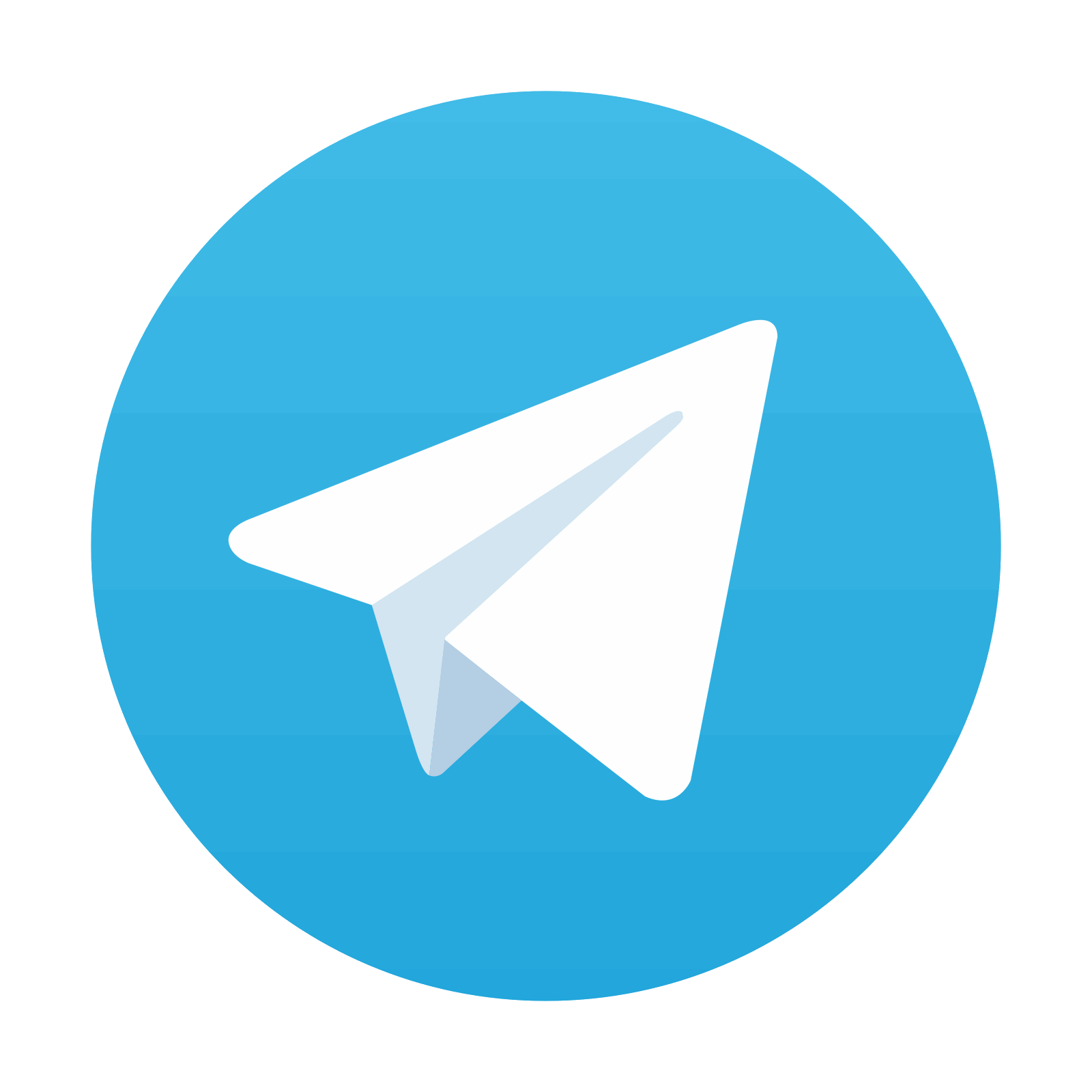
Stay updated, free articles. Join our Telegram channel

Full access? Get Clinical Tree
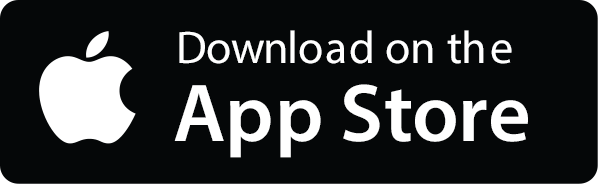
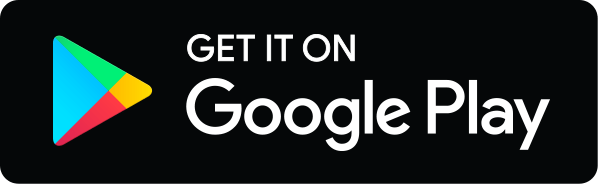