div class=”ChapterContextInformation”>
14. Neonatal Anesthesia
Keywords
Endotracheal intubation, neonateTracheo-esophageal fistula, anesthesiaNeonatal apnea and anesthesiaNeonatal pharmacologyNeonatal laparotomy anesthesiaThis chapter outlines differences between the neonate and older patients, some common neonatal conditions, and some aspects to consider in the care of the preterm neonate so that trainees will have some background knowledge if they are involved with these patients.
14.1 The Neonate
Various terms used to describe the age of preterm neonates
Term | Description |
---|---|
Gestational age | Time between the first day of the last menstrual period and delivery. A term baby is 37–40 weeks gestation |
Chronological age (Post-natal age) | Time since birth. A baby celebrates its first birthday 52 weeks after birth |
Postmenstrual age (PMA) or Post-conceptual age (PCA) | Gestational age + chronological age. A 6 week old baby born at 35 weeks gestation has a PMA of 41 weeks |
Corrected age | Chronological age minus the number of weeks born before 40 weeks of gestation. A 6 week old baby born at 35 weeks has a corrected age of 1 week |
14.2 The Neonatal Cardiovascular System
Respiratory and cardiovascular differences between neonate and adult
Parameter | Neonate | Adult |
---|---|---|
Oxygen consumption | 7 mL/kg/min | 3 mL/kg/min |
RR (breaths/min) | 30–60 | 15–20 |
MV | 220 mL/kg/min | 100 mL/kg/min |
Tidal volume | 6 mL/kg | 7 mL/kg |
FRC | 30 mL/kg | 34 mL/kg |
Anatomical dead space | 2.2 mL/kg | 2.2 mL/kg |
Cardiac output | 200 mL/kg/min | 70 mL/kg/min |
Characteristics of the cardiovascular system in neonates
Cardiovascular system characteristics | Reason |
---|---|
Contractility dependent on extracellular calcium concentration | Poorly developed sarcoplasmic reticulum |
Rate dependent cardiac output | Relatively fixed stroke volume from non- compliant ventricle |
Poorly developed sympathetic nervous system | Unable to increase systemic vascular resistance |
Parasympathetic nervous system predominance | Prone to bradycardia |
14.2.1 Patent Ductus Arteriosus (PDA)
The ductus arteriosus is a vascular communication between the pulmonary artery and descending aorta, and is an essential component of fetal life. It generally closes soon after birth but in some types of congenital heart disease, ongoing patency may be essential for survival. A small PDA is usually benign and NSAIDs may be used to promote closure. Left-to-right shunting through a large PDA risks pulmonary overload and congestive heart failure. This is managed with fluid restriction and diuretics. Surgical closure may be required, either by cardiac catheter or by thoracotomy.
14.3 The Neonatal Airway
Summary of airway differences
Neonatal airway features |
---|
Occiput relatively large—more difficult to position for optimal intubating conditions |
Obligate nasal breathing, most resistance in nose, don’t cope with nasal obstruction. 40% of term babies can convert to oral breathing if nasal obstruction |
Underdeveloped mandible with little space in mouth for tongue |
Larynx higher in neck with fewer vertebral joints above larynx that can flex |
Long, floppy, U-shaped epiglottis |
Larynx appears to be more anterior at laryngoscopy |
Vocal cords angled forward (more likely to catch ETT on anterior commissure) |
Short trachea makes right endobronchial intubation more likely |
Note
Positioning for intubation is different in neonates compared to adults: A head ring to stabilize the relatively large head; a small roll under the shoulders if the head is particularly large; mild head extension (too much and the epiglottis may be pushed against the tongue base); no neck flexion needed because of their high larynx.
14.4 The Neonatal Respiratory System
Neonates have several differences that place them at risk of respiratory and ventilatory failure.
14.4.1 Lung Development
The lung is underdeveloped at birth—alveoli develop late in gestation and lung development continues after birth. A preterm baby has only terminal sacs with underdeveloped alveolar ducts. Term babies have 20–50 million alveoli and the number increases to the adult number of 300 million by 8 years. Surfactant production begins around 23 weeks gestation and sufficient levels are present from early in the third trimester through to birth. Surfactant deficiency is a problem in preterm neonates, resulting in reduced compliance, atelectasis and respiratory distress syndrome (RDS). Surfactant is so important for lung function in neonates that antenatal steroids are given to mothers to stimulate surfactant production if delivering at 34 weeks or less. Preterm neonates born at less than 30 weeks gestation are given surfactant via an ETT. Bronchopulmonary dysplasia (BPD) refers to lung damage caused by mechanical ventilation and subsequent inflammatory reaction.
14.4.2 Airway and Respiratory Mechanics
List of the major differences in respiratory physiology in neonates compared to children and adults
Respiratory physiology in neonate |
---|
Horizontal ribs rather than ‘bucket handle’ |
Piston-like, diaphragmatic breathing which is compromised by gastric or abdominal distension |
Diaphragm has less type I muscle fibers (25% vs adult 60%; adult levels by 9 months) and copes with increased work of breathing poorly. Diaphragm is flatter and develops less pressure for any given muscle tension |
Compliant rib cage which in-draws if upper airway obstruction |
Small diameter, poorly supported airways |
Immature respiratory control |
Note
Neonates and infants younger than 3 months are termed ‘obligate nasal breathers’ because less than half can quickly convert to breathing through their mouth if their nose is obstructed.
14.4.3 Control of Respiration
The respiratory center in the brain stem of the neonate is immature, and respiratory control is not fully developed. Neonates have periodic breathing- the respiratory rate varies and includes periods of self-correcting apnea lasting 5 or 10 s. Neonates also have a biphasic response to hypoxia—they increase ventilation initially, but then become apneic. After about 3 weeks of age the response to hypoxia is sustained hyperventilation as in children and adults. Neonates also have a reduced response to hypercarbia compared with children and adults. Finally, neonates have increased sensitivity to stimulation in the superior laryngeal nerve territory and respond with hypoventilation, apnea or bradycardia.
14.4.4 Apnea and Anesthesia in Neonates
Summary of apnea in infants after anesthesia and sedation
Key features | Notes |
---|---|
‘Apnea’ | Longer then 15 s, or 10 s if associated desaturation or bradycardia Usually within first few hours after anesthesia Risk period extends to 12 h post op Usually responds to stimulation; some require IPPV |
Risk groups | Other preterm baby (less than 35 weeks) until 52 weeks PMA Mildly preterm baby (35–37 weeks) until 48 weeks PMA Term baby until 44 weeks PMA |
Other risk factors in preterm infants | Co-morbidities (especially neurological, respiratory) Intraoperative opioids or sedatives Anesthesia technique and agents Anemia? |
Prevention | Analgesia without opioids Light GA with low-solubility volatile and regional analgesia Caffeine base 10 mg/kg Spinal anesthesia |
14.4.4.1 Risk Factors for Apnea After Anesthesia
Preterm infants aged less than 52 weeks PMA are at risk of apnea after anesthesia or sedation. The risk declines with age, is very low after 46 weeks PMA and is negligible after 52 weeks PMA. Preterm infants under 44 weeks PMA are most at risk. The degree of prematurity at birth also affects the risk—infants born mildly preterm at 35–37 weeks have a lower risk of apnea than neonates born before 35 weeks. Term neonates (born at 37 weeks gestation or more) are at a lower risk of apnea than preterm neonates, but a risk exists until 44 weeks PMA. Co-morbidities including anemia (Hb <100 g/L), lung disease, neurological problems and pre-existing apnea increase the risk of postop apnea.
Keypoint
Term neonates require admission and monitoring for postoperative apnea until a postmenstrual age of 44 weeks, and preterm infants until 52 weeks (some centers still use 60 weeks).
14.4.4.2 Prevention Strategies to Reduce Apnea
The risk of postoperative apnea can be reduced by postponing elective surgery until the infant is older. Term infants should not have day-stay surgery until they are 44 weeks PMA (that is, 4 weeks old if born at 40 weeks gestation, 7 weeks old if born at 37 weeks). Preterm infants should not have day-stay surgery until they are 52 weeks PMA (some centers use 60 weeks). The risk in infants born mildly preterm (35–37 weeks) is lower and some centers allow day-stay surgery after 48 weeks PMA in these infants if there are no other risk factors. This last group still needs to be monitored for 6–8 apnea-free hours before discharge. Although anemia increases apnea risk, many centers accept mild anemia unless there are other reasons for transfusion.
General anesthesia can be modified to reduce the risk. Regional or local anesthesia should be used in place of opioids, allowing a light plane of anesthesia with relatively insoluble agents such as sevoflurane. Longer acting drugs of all classes should be avoided.
Spinal anesthesia was thought to greatly reduce the risk of postop apnea. More recent work suggests spinal anesthesia does not reduce the overall incidence of apnea compared to general anesthesia. However, it does reduce the number of infants needing any intervention greater than stimulation to resolve their apnea, and the number of apneas in the PACU. Spinal anesthesia is discussed in Chap. 10, Sect. 10.5.4. In summary, its disadvantages are technical difficulties with lumbar puncture in small infants, and the short duration of spinal anesthesia in infants.
IV caffeine during anesthesia reduces the incidence of postop apnea. Preterm infants at high risk (44 weeks PMA or less) are given caffeine base 10 mg/kg IV (equivalent to caffeine citrate 20 mg/kg) during anesthesia to prevent apnea. Caffeine is also used in the neonatal nursery to prevent apnea in premature neonates, so it is important to check the baby has not already been given caffeine. Aminophylline can be used if IV caffeine is not available, although it has more cardiovascular side effects.
Keypoint
Spinal anesthesia was thought to greatly reduce the risk of postop apnea. It is now realized it does not affect the overall incidence of postop apnea, but does reduce the severity of apneas and incidence of early apneas.
14.4.4.3 Monitoring for Apnea
Detection of apnea prevents hypoxia or hypoxic cardiac arrest. An ‘apnea monitor’ is used, usually in combination with pulse oximetry. The monitor uses ECG leads on the chest and detects respiratory movement via the impedance between the leads and measures heart rate via the ECG. It will not detect obstructive apnea (chest moving but no air flow) until bradycardia develops. Most apnea begins in the first few of hours after anesthesia. The risk diminishes with time and monitoring is ceased when there has been no apnea for 12 h.
Apneas nearly always respond to stimulation alone and rarely require bag-mask ventilation. Groups of infants that require monitoring are those in the ‘risk group’ of Table 14.6.
14.5 Fluid and Glucose Requirements
Differences in body fluid compartments and fluid requirements between neonate and adult
Fluid compartment | Neonate | Adult |
---|---|---|
Total body water | 70–75% | 65% |
Extracellular fluid volume | 50% | 25% |
Blood volume | 90 mL/kg | 70 mL/kg |
Sodium requirement | 3 mmol/kg/day | |
Glucose requirement | 6–8 mg/kg/min | |
Fluid requirements | ||
Day 1 | 60 mL/kg/24/h | |
Day 2 | 75 | |
Day 3 | 90 | |
Day 4 | 105 | |
Day 5 | 120 | |
Day 7 onwards | 150 |

Glomerular filtration rate (GFR) at birth is roughly one quarter of the adult, but reaches the adult level at 2 years of age. Modified from Anderson BJ, Holford NHG. Negligible impact of birth on renal function and drug metabolism. Pediatr Anesth 2018;28: 1015–21
14.5.1 Neonatal Blood
The neonate has predominantly fetal hemoglobin (HbF) which has an oxygen dissociation curve shifted to the left—oxygen extraction at the tissue level is impaired due to the higher venous oxygen levels after birth. The hemoglobin level at birth is variable, but commonly about 16 g/dL. Adult hemoglobin (HbA) is produced from birth, but red cell production is inadequate and the hemoglobin falls, reaching a low point of 8–11 g/dL at 2–3 months (called the ‘physiological anemia’). Nearly all of the hemoglobin at this stage is HbA, and so tissue oxygen delivery is actually improved compared to earlier with HbF.
Note
The presence of HbF in neonates is a key reason for a higher transfusion-trigger hemoglobin in neonates than children.
The coagulation system of the neonate is immature and does not reach adult levels until about 6 months of age. The coagulation changes are due to reduced levels of the vitamin K dependent factors and reduced levels of coagulation inhibitors (Antithrombin III, Protein C and S). Vitamin K is often given to neonates because of this coagulopathy. Platelet numbers are normal, but they do not reach adult activity until the neonate is 2 weeks old. Neonates do not have blood group antibodies in their plasma apart from some transferred through the placenta from the mother. Cross match of blood is performed on maternal serum.
14.6 Temperature
Neonates can only control body temperature over narrow range of environmental temperatures compared to children and adults. Their thermoneutral temperature depends on the age and weight of the baby, but for a naked term baby it is 32–35 °C. Methods to maintain body temperature are during surgery are described in Sect. 14.9.2.
14.6.1 Heat Loss and Production
Neonates have large heat losses and a decreased ability to generate heat, so are at great risk of hypothermia during transport and while in theatre. Losses are through the skin, particularly by convection and radiation. Evaporation is also an important source of heat loss in preterm infants because of their thin skin. Heat losses are high because of the neonate’s large surface area to weight ratio and poor insulation from subcutaneous fat. The head (20% of surface area) is a significant site of heat loss and should be kept covered.
Heat production is limited—neonates do not shiver, or at least not enough to generate any heat. They do however have brown fat that is rich in mitochondria located around the great vessels in the neck and thorax, and also in the axilla and between the scapulae. This fat is used for non-shivering thermogenesis, which can double heat production in neonates and infants until 2 years of age. Non-shivering thermogenesis is inhibited by anesthesia, as is shivering in older children and adults.
Tip
Think of heat loss when you uncover an infant to insert an IV.
Consider underbody or overhead warming, covering patient with a clear plastic sheet, insulating cap for the head.
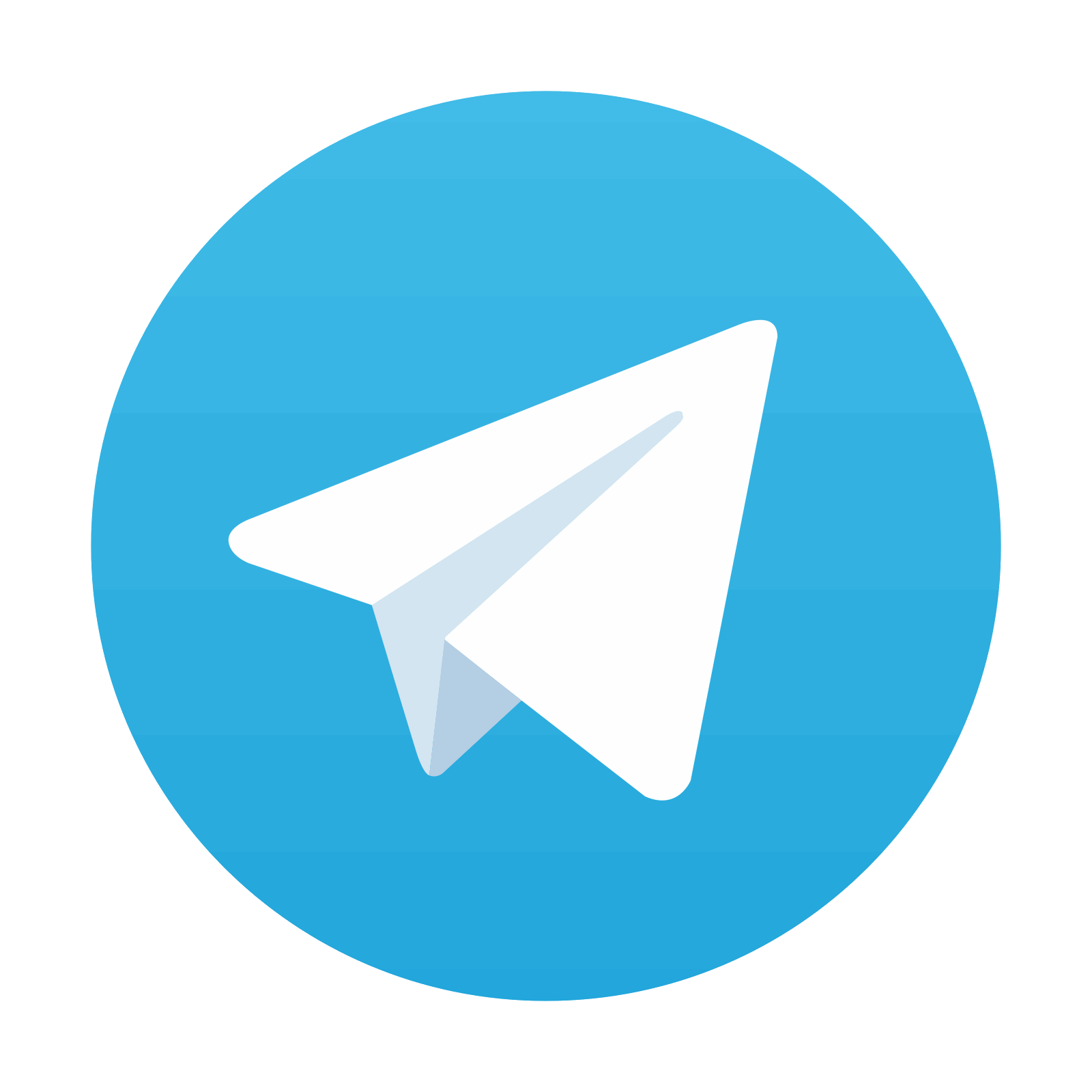
Stay updated, free articles. Join our Telegram channel

Full access? Get Clinical Tree
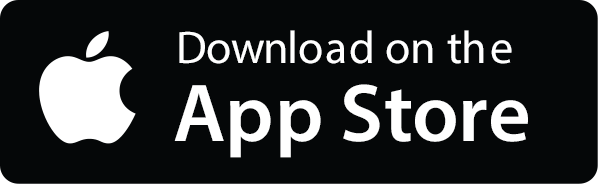
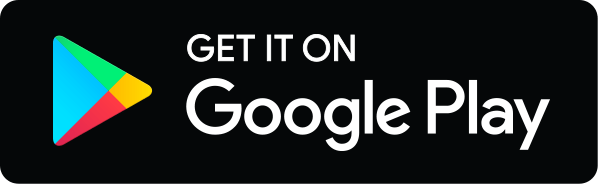