2.2 Anatomy and biomechanics of the pelvis
(Adapted from Chapter 8 of Movement, Stability and Lumbopelvic Pain (Vleeming & Stoeckart 2007).)
In all quadrupeds and bipeds, the pelvic girdle forms a firm connection between the spine and the lower extremities. In bipeds, the pelvis has to serve as a basic platform with three large levers acting on it (the spine and the legs). To allow bipedal gait in humans, evolutionary adaptations of the pelvis have been necessary, i.e. changing the shape of the ilia, flaring out into the sagittal plane, providing a more optimal lateral attachment site for the gluteus medius as an important muscle for hip pelvic stability. In particular, a dramatically increased attachment site for the gluteus maximus muscle has changed this muscle – a relatively minor muscle in the chimpanzee – into one of the largest muscles of the human body (Lovejoy 1988).
Additional evolutionary changes in humans are the muscular and ligamentous connections between the sacrum and ilia: (1) muscles, like the lower lumbar multifidi, that insert into the sacrum and also into the medial cranial aspects of the ilium; (2) changes in the position of the coccygeus and the piriform muscles, and of the gluteus maximus muscle originating from the sacrum and sacrotuberous ligaments; (3) extensive fibrous connections adapted to the typical anatomy of the sacroiliac joints (SIJs), like the interosseous ligaments, surrounding an iliac protrusion fitting in a dorsal sacral cavity, called the axial joint just behind the auricular surfaces of the SIJ (Bakland & Hansen 1984); (4) ventral and dorsal SIJ ligaments, sacrotuberous and sacrospinous ligaments between sacrum and lumbar spine (anterior longitudinal ligaments). In addition, direct fibrous connections exist between the iliac bone and L4 and L5, the iliolumbar ligaments. A recent study has described the influence of the iliolumbar ligaments on SIJ stability (Pool-Goudzwaard et al. 2003). Due to the above-mentioned muscular and ligamentous connections, movement of the sacrum with respect to the iliac bones, or vice versa, affects the joints between L5–S1 and between the higher lumbar levels. Anatomical and functional disturbances of the pelvis or lumbar region influence each other. Due to the tightness of the fibrous connections and the specific architecture of the SIJ, mobility in the SIJ is normally very limited, but movement does occur and has not been scientifically challenged (Weisl 1955, Solonen 1957, Egund et al. 1978, Lavignolle et al. 1983, Miller et al. 1987, Sturesson et al. 1989, 2000a, 2000b, Vleeming et al. 1990a, 1990b, 1996) (Figure 2.2.1).
The main movements in the SIJ are forward rotation of the sacrum relative to the iliac bones (nutation) and backward rotation of the sacrum relative to the ilia (counternutation) (Figure 2.2.2). It was shown that even at advanced age (>72 years) the combined movement of nutation and counternutation can amount to 4°; normally movements are less than 2° (Vleeming et al. 1992a). In the latter study, the SIJ with the lowest mobility showed radiologically marked arthrosis. Ankylosis of the SIJ was found to be an exception, even at advanced age. This finding is in agreement with the studies of Stewart (1984) and Miller et al. (1987).
Nutation is increased in load-bearing situations, e.g. standing and sitting. In lying prone, nutation is also increased compared to supine positions (Weisl 1955, Egund et al. 1978, Sturesson et al. 1989, 2000a, 2000b). Counternutation normally occurs in unloaded situations like lying down (supine). Counternutation in supine positions can be altered to nutation by maximal flexion in the hips, using the legs as levers to posteriorly rotate the iliac bones relative to the sacrum, as in a labour position, creating space for the head of the baby during delivery.
The anatomy of the sacroiliac joint
1. Why did nature create a seemingly flat SIJ?
2. What specific adaptations are available to prevent shear in the SIJs?
3. Why is the SIJ not perpendicularly orientated to the forces of gravitation?
Why did nature create a seemingly flat SIJ?
A large transfer of forces is required in the human SIJ, and indeed flat joints are theoretically well-suited to transfer large forces (Snijders et al. 1993a, 1993b). An alternative for effective load transfer by these flat joints would be a fixed connection between sacrum and iliac bones, for instance by ankylosis of the SIJ. The SIJs in humans serve a purpose: to economize gait, to allow shock and shear absorption, and to alleviate birth of (in the evolutionary sense) abnormally large babies. The principal function of the SIJs is to act as stress relievers, ensuring that the pelvic girdle is not a solid ring of bone that could easily crack under the stresses to which it is subjected (Adams et al. 2002).
What specific adaptations are available to prevent shear in the SIJs?
The SIJs are abnormal compared to other joints because of cartilage changes that are present already before birth. These occur especially at the iliac side of the joint and were misinterpreted as degenerative arthrosis (Sashin 1930, Bowen & Cassidy 1981). These cartilage changes are more prominent in men than in women and, according to Salsabili et al. (1995), the sacral cartilage is relatively thick in females. This gender difference might be related to childbearing and possibly to a different localization of the centre of gravity in relation to the SIJ (Dijkstra et al. 1989, Vleeming et al. 1990a, 1990b). Vleeming et al. (1990a, 1990b) considered these changes to reflect a functional adaptation. The features seem to be promoted by the increase in body weight during the pubertal growth spurt and concern a coarse cartilage texture and a wedge and propeller-like form of the joint surfaces.
Studies of frontal slides of intact joints of embalmed specimens show the presence of cartilage-covered bone extensions protruding into the joint. These protrusions seemed irregular but are in fact complementary ridges and grooves. Joint samples taken from normal SIJ with both coarse texture and complementary ridges and grooves were characterized by high-friction coefficients (Vleeming et al. 1990b). All these features are expected to reflect adaptation to human bipedality, contributing to a high coefficient of friction and enhancing the stability of the joint against shear (Vleeming et al. 1990a). As a consequence, less muscle and ligament force is required to bear the upper part of the body (Figure 2.2.3).
The ‘keystone-like’ bony architecture of the sacrum further contributes to its stability within the pelvic ring. The bone is wider cranially than caudally, and wider anteriorly than posteriorly. Such a configuration permits the sacrum to become ‘wedged’ cranially and dorsally into the ilia within the pelvic ring (Vleeming et al. 1990a, 1990b). The SIJ has evolved from a relatively flat joint into a much more stable construction (Figure 2.2.4).
To illustrate the importance of friction in the SIJ, the principles of form and force closure were introduced (Vleeming et al. 1990a, 1990b). Form closure refers to a theoretical stable situation with closely fitting joint surfaces, where no extra forces are needed to maintain the state of the system, given the actual load situation. If the sacrum would fit in the pelvis with perfect form closure, no lateral forces would be needed. However, such a construction would make mobility practically impossible. With force closure (leading to joint compression) both a lateral force and friction are needed to withstand vertical load. Shear in the SIJ is prevented by the combination of the specific anatomical features (form closure; see Figure 2.2.5) and the compression generated by muscles and ligaments that can be accommodated to the specific loading situation (force closure). Force closure is the effect of changing joint reaction forces generated by tension in ligaments, fasciae and muscles and ground reaction forces (Figure 2.2.5).
Why is the SIJ not perpendicularly orientated to the forces of gravitation?
Force closure ideally generates a perpendicular reaction force to the SIJ to overcome the forces of gravity (Vleeming et al. 1990b). This shear prevention system was named the self-bracing mechanism and such a mechanism is present elsewhere in the body, e.g. in the knee, foot and shoulder. When a larger lever is applied and/or coordination time becomes less, the general effect in the locomotor system will be closure or reduction of the degrees of freedom of the kinematic chain, leading to a reduction in the chain’s mobility or a gain of stability by increasing force closure (Huson 1997).
In self-bracing of the pelvis, nutation of the sacrum is crucial. This movement can be seen as an anticipation for joint loading. Hodges et al. (2003) use the terminology ‘preparatory motion’ for a comparable phenomenon in the lumbar spine. So, nutation is seen as a movement to prepare the pelvis for increased loading by tightening most of the SIJ ligaments, among which are the vast interosseous and short dorsal sacroiliac ligaments. As a consequence the posterior parts of the iliac bones are pressed together, enlarging compression of the SIJ.
Ligaments and their role in self-bracing the pelvis
In self-bracing the pelvis, nutation in the SIJ is crucial (see above); this involves several ligaments. To further explain self-bracing of the pelvis we will discuss two sets of ligaments (Figure 2.2.6): the sacrotuberous ligaments (Vleeming et al. 1989a, 1989b, van Wingerden et al. 1993) and the long dorsal SI ligaments (Vleeming et al. 1996, 2002). In the literature, specific data on the functional and clinical relevance of the long ligaments are not available. In several anatomical atlases and textbooks, the long ligament and the sacrotuberous ligament are portrayed as fully continuous ligaments. The drawings generally convey the impression that the ligaments have identical functions. As shown by the contrasting effects of nutation and contranutation on these ligaments (see below), this is not the case. Essentially, the long ligament connects the sacrum and posterior superior iliac spine (PSIS), whereas the main part of the sacrotuberous ligament connects the sacrum and ischial tuberosity. However, some of the fibres derived from the ischial tuberosity pass to the iliac bone. Generally, they are denoted as part of the sacrotuberous ligament, although ‘tuberoiliac ligament’ would be more appropriate. In the terminologia anatomica such a ligament does not exist. In fact, this also holds for the long (dorsal SI) ligament, reflecting one of the problems of topographical anatomy.
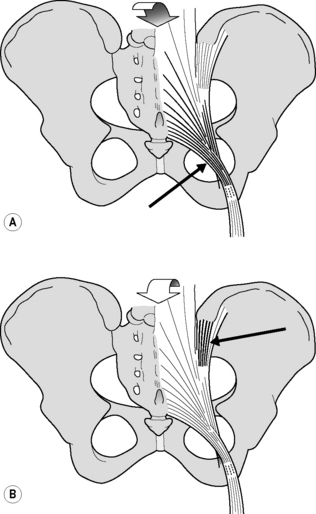
Figure 2.2.6 • (A) Nutation winds up the sacrotuberous ligament. (B) Counternutation winds up the long dorsal sacroiliac ligament
Sacrotuberous ligaments
In embalmed human specimens, we could demonstrate a direct relation between nutation and tension of the sacrotuberous ligament (Figure 2.2.6A). By straining this ligament, we found a decrease of nutation (Vleeming et al. 1989a, 1989b), indicating that these ligaments are well-suited to restrict nutation. It can be expected that the opposite (diminished ligament tension) will increase nutation.
Long dorsal sacroiliac ligaments
In view of the capability of the sacrotuberous ligaments to restrict nutation, we wondered which ligament(s) could restrict counternutation. Because of its connection to the PSIS and to the lateral part of the sacrum (Figure 2.2.6B), we expected that the long dorsal SI ligament could fulfil this function. The ligament can be easily palpated in the area directly caudal to the PSIS and is of special interest since women complaining of lumbopelvic back pain during pregnancy frequently experience pain within the boundaries of this ligament (Mens et al. 1992, Njoo 1996, Vleeming et al. 1996). Pain in this area is also not uncommon in men. The ligament is the most superficially located SIJ ligament and therefore well-suited to mirror asymmetric stress in the SIJ. As this ligament is not well known in medical practice, we will summarize data from an anatomical and biomechanical study (Vleeming et al. 1996) that assessed the function of the ligament by measuring its tension during incremental loading of biomechanically relevant structures.
Biomechanical aspects
This observation implies that the tension of the long ligament can be altered by displacement in the SIJ as well as by action of various muscles. Obviously, nutation in the SIJ induces relaxation of the long ligament, whereas counternutation increases tension. This is in contrast to the effect on the sacrotuberous ligament (see Figure 2.2.5). Increased tension in the sacrotuberous ligament during nutation can be due to SIJ movement itself as well as to increased tension of the biceps femoris and/or gluteus maximus muscle. This mechanism can help to control nutation. As counternutation increases tension in the long ligament, this ligament can assist in controlling counternutation (see Figure 2.2.6).
A comparable complex relation might hold for the long ligament and the erector spinae, or more specifically the multifidus muscle. As the multifidus is connected to the sacrum (MacIntosh & Bogduk 1986, 1991; see Chapter 1), its action induces nutation. As a result, the long ligament will slacken. However, the present study shows an increase in tension in the long ligament after traction to the erector spinae muscle. This counterbalancing effect is due to the connections between the erector spinae muscle and the long ligament, and opposes the slackening. In vivo, this effect might be smaller because the moment of force acting on the sacrum is raised by the pull of the erector spinae muscle and the resulting compression force on the spine (Snijders et al. 1993b). This spinal compression was not applied in this study. Both antagonistic mechanisms – between long and sacrotuberous ligaments and between the long ligament and the erector spinae muscle – might serve to preclude extensive slackening of the long ligament. Such mechanisms could be essential for a relatively flat joint such as the SIJ, which is susceptible to shear forces (Snijders et al. 1993a, 1993b). It can be safely assumed that impairment of a part of this interconnected ligament system will have serious implications for the joint as load transfer from spine to hips and vice versa is primarily transferred via the SIJ (Snijders et al. 1993a, 1993b).
As shown earlier (Vleeming et al. 1996), traction to the biceps femoris tendon hardly influences tension of the long ligament. This is in contrast to the effect of the biceps on the sacrotuberous ligament (Vleeming et al. 1989a, 1989b, van Wingerden et al. 1993). The observations might well be related to the spiralling of the sacrotuberous ligament. Most medial fibres of the ligament tend to attach to the cranial part of the sacrum, whereas most fibres arising from a lateral part of the ischial tuberosity tend to attach to the caudal part of the sacrum (see Figure 2.2.6A). The fibres of the biceps tendon, which approach a relatively lateral part of the ischial tuberosity, pass mainly to the caudal part of the sacrum. As a consequence, the effect of traction to the biceps femoris on the tension of the long ligament can only be limited.
The effect on the long ligament of loading the posterior layer of the thoracolumbar fascia depends on the direction of the forces applied. Artificial traction to the fascia mimicking the action of the transverse abdominal muscle has no effect. Traction in a craniolateral direction, mimicking the action of the latissimus dorsi muscle, results in a significant decrease in tension in the ipsilateral and contralateral long ligaments. As shown in another study (Vleeming et al. 1995), traction to the latissimus dorsi influences the tension in the posterior layer of the thoracolumbar fascia, ipsilaterally as well as contralaterally, especially below the level of L4. Thus slackening of the long ligament could be the result of increased tension in the posterior layer by the latissimus dorsi. This might itself lead to a slight nutation, leading to more compression and force closure of the SIJ. As shown in this study, slackening of the long ligament can also occur due to action of the gluteus maximus muscle, which is ideally suited to compress the SIJ.
It is inviting to draw conclusions when palpation, directly caudal to the PSIS, is painful. However, pain in this area might be due to pain referred from the SIJ itself (Fortin et al. 1994a, 1994b), but also due to counternutation in the SIJ. Counternutation is part of a pattern of flattening the lumbar spine (Egund et al. 1978, Lavignolle et al. 1983, Sturesson et al. 1989) that occurs in particular late in pregnancy when women counterbalance the weight of the fetus (Snijders et al. 1976). However, such a posture combined with counternutation could also result from a pain-withdrawal reaction to impairment elsewhere in the system. Hence, only specific pain within the boundaries of the long ligament can be used as a diagnostic criterion (Vleeming et al. 2002). An example of a pain-withdrawal reaction could be the following: pain of the pubic symphysis following delivery (Mens et al. 1992) could preclude normal lumbar lordosis and hence nutation owing to pain of an irritated symphysis. After all, lumbar lordosis leads to nutation in the SIJ (Weisl 1955, Egund et al. 1978, Lavignolle et al. 1983, Sturesson et al. 1989
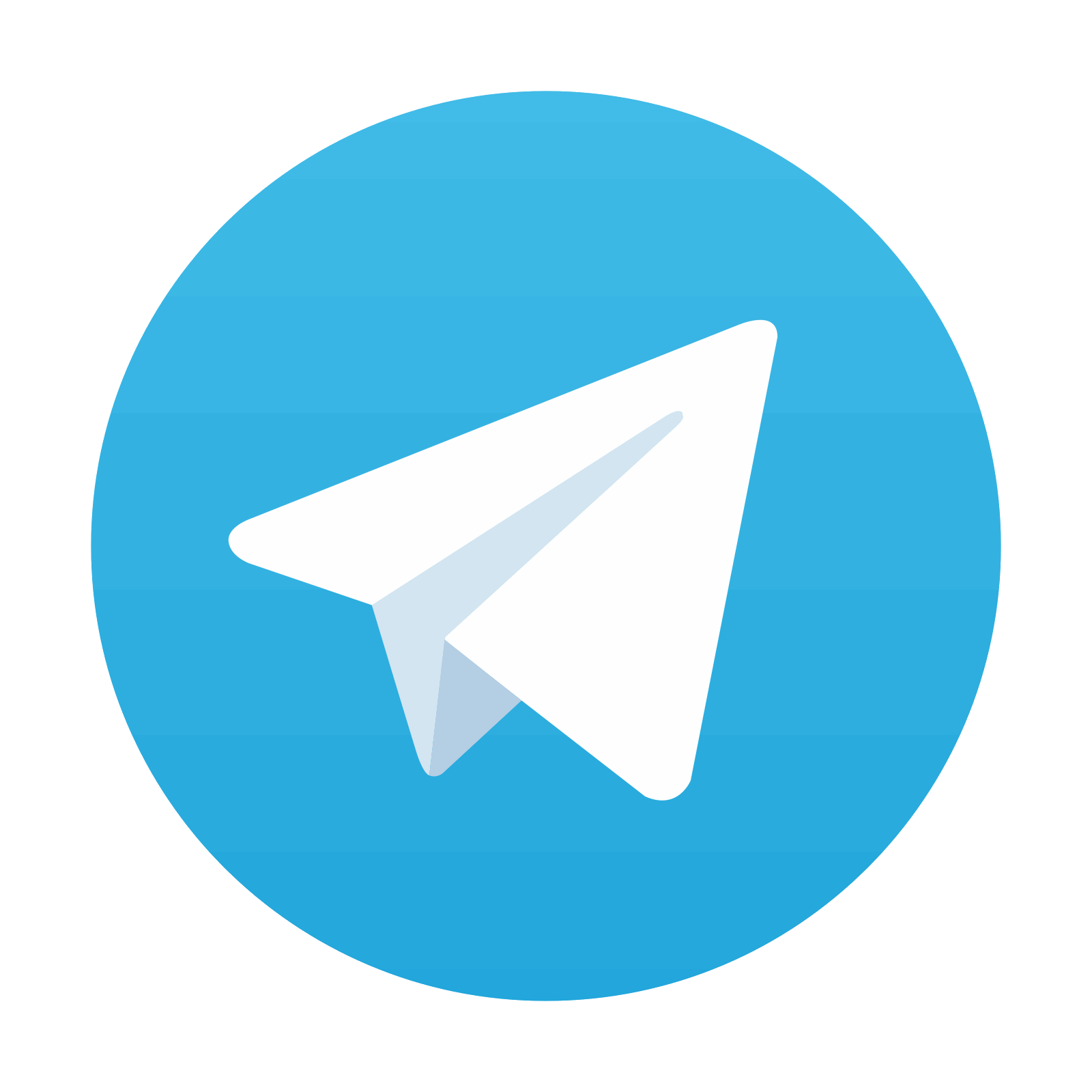
Stay updated, free articles. Join our Telegram channel

Full access? Get Clinical Tree
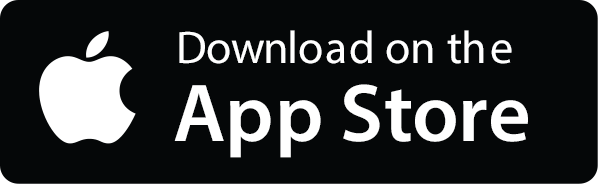
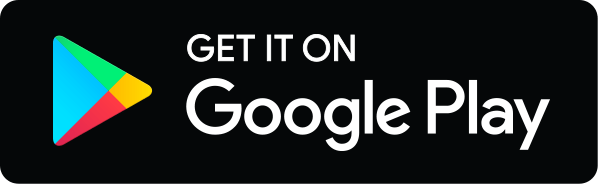