KEY POINTS
Anaphylaxis is an acute life-threatening systemic reaction that results from sudden systemic release of mediators from mast cells and basophils.
Degranulation of mast cell and basophils are commonly mediated by IgE antibody. Other nonimmunologic mechanisms including direct activation of these cells have been described.
The incidence of anaphylaxis appears to be rising, especially among young people.
Foods followed by medications (eg, antibiotics and NSAIDs) are the most common cause of anaphylaxis in the outpatient setting.
Medications, for example, antibiotics, muscle relaxants, blood products, and radiocontrast media, are common causes of anaphylaxis in the hospital.
Onset of symptoms of anaphylaxis is usually immediate but can be delayed by 2 to 10 hours.
Cutaneous symptoms are common but hemodynamic collapse and shock can occur in the absence of skin manifestations.
The hemodynamic symptoms of anaphylaxis are secondary to the widespread vasodilation and profound intravascular fluid loss.
Careful history and physical examination are most important in the diagnosis of anaphylaxis. Measurement of serum tryptase and histamine can be helpful.
Prompt recognition, administration of epinephrine, and intravascular volume replacement are key factors in the successful outcome of this potentially fatal event.
INTRODUCTION/DEFINITION
The traditional definition of anaphylaxis is “a systemic, immediate hypersensitivity reaction caused by immunoglobulin E (IgE)–mediated immunologic release of mediators from mast cells and basophils.” The term “anaphylactoid” reaction has been traditionally defined as a clinically similar event not mediated by IgE.1,2 More recently, the World Allergy Organization (WAO) has referred to anaphylaxis as a “severe, life-threatening, generalized or systemic hypersensitivity reaction.” It suggested that the term ‘‘anaphylactoid reaction’’ be eliminated, and that all episodes clinically similar to IgE-mediated reactions be called anaphylaxis.3
The difficulty in determining the clinical manifestations that define an anaphylactic event was highlighted in a symposium sponsored by the National Institute of Health and the Food Allergy and Asthma Network.4,5 This symposium was convened to define the clinical manifestations of anaphylaxis required to establish a diagnosis. No true definition, in the classic sense of the term, resulted from the deliberations of this group, but they did define a clear-cut constellation of signs and symptoms requiring the necessity for treatment with epinephrine. They formulated three clinical scenarios during which anaphylaxis was highly likely as a cause of the event and thus epinephrine therapy mandated. These scenarios can be summarized briefly as follows:
Acute onset of an illness (minutes to several hours) with involvement of the skin, mucosal tissue, or both and at least one of the following:
Respiratory compromise
Reduced BP or associated symptoms of end-organ dysfunction
Two or more of the following that occur rapidly after exposure to a likely allergen for that patient (minutes to several hours):
Involvement of the skin-mucosal tissue
Respiratory compromise
Reduced BP or associated symptoms
Persistent gastrointestinal symptoms
Reduced BP after exposure to known allergens for that patient (minutes to several hours).
EPIDEMIOLOGY
The true incidence and prevalence of anaphylaxis is currently not known because of a lack of any controlled studies. Underdiagnosis, underreporting, and miscoding are substantial obstacles preventing an accurate estimation.6-8 Table 128-1 summarizes the major studies of incidence and prevalence and their relevant findings. A recent expert panel concluded that the lifetime prevalence of anaphylaxis is about 0.05% to 2%.9 The same study reported that the incidence is approximately 50 to 2000 episodes per 100,000 person-years.
Incidence and Prevalence of Anaphylaxis.
Author | Year | Description of study | Findings |
---|---|---|---|
Yocum et al177 | 1999 | Rochester Epidemiology Project. | During the years 1983-1987, the average annual incidence rate was 21/100,000 person-years, and the most common triggers were foods, medications, and insect stings. |
Simons et al178 | 2002 | Dispensing data for all injectable epinephrine formulations over 5 consecutive years. | 0.95% had injectable epinephrine dispensed for out-of-hospital treatment. |
Bohlke et al179 | 2004 | Large HMO in the United States, 1991-1997. | The incidence rate was 10.5 anaphylactic episodes per 100,000 person-years. |
Helbling et al180 | 2004 | Investigated anaphylaxis with circulatory symptoms during a 3-year period, 1996-1998, in Bern, Switzerland. | Incidence rate of 7.9-9.6/100,000 person-years. |
Lieberman et al9 | 2006 | Panel convened to review major epidemiologic studies of anaphylaxis. | There was a frequency estimate of 50 to 2000 episodes/100,000 person-years or a lifetime prevalence of 0.05% to 2%. |
Poulos et al181 | 2007 | Data on hospital admissions for anaphylaxis were extracted for the periods 1993-1994 to 2004-2005, respectively. | There was a continuous increase by 8.8% per year in the incidence rate of ED visits/hospitalizations for anaphylaxis. |
Camargo et al12 | 2007 | State-by-state dispensing data (filled prescriptions, including refills) for epinephrine autoinjectors in 2004 in the United States. | Average was 5.71 Epi-pens per 1000 persons (range from 2.7 in Hawaii to 11.8 in Massachusetts). |
Decker et al182 | 2008 | Population-based incidence study from 1990-2000 in the Rochester Epidemiology Project. | Overall age- and sex-adjusted incidence rate of 49.8/100,000 persons; the annual incidence rate increased from 1990 to 2000. |
Lin et al183 | 2008 | Characterization of anaphylaxis hospitalizations in New York state in patients <20 years of age. | During the study period, 1990-2006, the anaphylaxis hospitalization rate increased by more than fourfold. |
Sheikh et al184 | 2008 | Recorded incidence and lifetime prevalence of anaphylaxis in England were investigated by using QRESEARCH, a national aggregated primary health care database containing the records of >9 million patients. | Age/sex standardized incidence of anaphylaxis was 6.7/100,000 person-years in 2001 and increased by 19% to 7.9/100,000 person-years in 2005; lifetime age/sex standardized prevalence of anaphylaxis was 50/ 100,000 in 2001 and increased by 51% to 71.5/100,000 in 2005. |
Geographic locations, age, gender, route of administration of antigen, and atopy have been found to influence the incidence of anaphylaxis.10-13
Anaphylaxis is more common in males until age 15 years and more common in females after age 15 years. This has been documented in several studies.13,14 The reason for such gender differences is not clear, but probably relates to hormonal influences. Anaphylaxis is more frequent in adults than children for some agents: radiocontrast media, plasma expanders, and anesthetics.
Camargo et al12 investigated the epidemiology of anaphylaxis using the number of autoinjector epinephrine prescription filled in 50 states and Washington, DC. A strong north-south gradient was observed for the prescription of autoinjectors in the United States, with the highest rates found in New England. This finding persisted after all other variables (eg, population demographic characteristics, number of health care providers, prescriptions for other medications) were adjusted. The authors concluded that regional differences in the number of automatic epinephrine injections prescribed may provide insight into the pathogenesis (eg, the potential role of vitamin D deficiency) of reactions experienced as an outpatient. In the United Kingdom, the incidence may be higher for those living in rural areas compared to urban areas.11
The relationship between atopy and anaphylaxis is complex. The incidence of latex anaphylaxis is clearly increased in atopic individuals15 but the same is not true for penicillin, insulin, insect stings, and muscle relaxants.10 Atopic subjects appear to be predisposed to anaphylaxis, but only a minority of such atopic individuals will experience an event.
ETIOLOGY
The various triggers of anaphylaxis are grouped according to the mechanism by which they cause anaphylaxis (Table 128-2). Overall drugs and foods are the most frequent causes of anaphylaxis.16
Mechanisms and Causes of Anaphylaxis
Immunologic mechanisms (IgE dependent) |
Foods: peanut, tree nut, shellfish, fish, milk, egg, sesame seed, and food additives |
Medications: β-lactam antibiotics, NSAIDs, and biological agents |
Venoms: stinging insects (Hymenoptera) |
Natural rubber latex |
Occupational allergens |
Seminal fluid (prostate-specific antigen) |
Inhalants: horse, hamster, and other animal danders and grass pollen (rare) |
Radiocontrast media |
Immunologic mechanisms (IgE independent) |
Dextran: high-molecular-weight iron dextran |
Infliximab |
Radiocontrast media |
Nonimmunologic mechanisms |
Physical factors: exercise, cold, heat, and sunlight/UV radiation |
Ethanol |
Medications, such as opioids |
Idiopathic anaphylaxis |
Foods are arguably the most common cause of anaphylaxis in the outpatient setting. Food allergens account for 30% of fatal cases.17,18 The prevalence of food-induced anaphylaxis is increasing. The most frequently incriminated foods are peanuts, tree nuts, fish, and shellfish, but other foods, such as sesame seeds, have become increasingly important as causes of food-induced anaphylaxis.18,19
Medications are the second most common overall cause of anaphylaxis, and perhaps the primary cause of anaphylaxis in adults.20 They are also the most common cause of anaphylaxis in the hospital setting. The most common classes of drugs producing anaphylaxis are antibiotics, especially β-lactam antibiotics, and nonsteroidal anti-inflammatory drugs (NSAIDs). β-Lactam antibiotics account for as many as 22% of all drug-related episodes.2 NSAIDs are the second most common medication offender.21 Depending on the country, anaphylactic reactions during the perioperative procedure represent 9% to 19% of complications occurring during anesthesia. The fatality rate approximates 5% to 7%. Muscle relaxants account for 62%, latex approximately 16%, and the remainder of reactions are due to hypnotics, antibiotics, plasma substitutes, and opioids.22 Other agents like antilymphocyte globulin and antivenom antisera have been mentioned as causes of anaphylaxis.
Anaphylaxis to anticancer chemotherapy drugs is increasing in incidence with the increasing use of these drugs. In particular, reactions to the platinum-containing drugs, such as cisplatinum and carboplatinum, are increasing in incidence.23 Anaphylactic events to biologic modifiers are also growing in importance. These include omalizumab (anti-IgE), an agent used to treat asthma,24 and cetuximab, a chimeric mouse/human IgG1 monoclonal antibody to epidermal growth factor receptor used in the treatment of colorectal cancer and squamous cell cancer of the head and neck.25
Radiographic contrast material (RCM) is used in more than 10 million radiologic examinations annually in the United States. The overall frequency of adverse reactions is 5% to 8%. Moderate reactions, such as severe vomiting, diffuse urticaria, or angioedema, that require therapy occur in about 1% of patients who receive RCM. However, life-threatening reactions occur with a frequency of less than 0.1% with conventional high-osmolality RCM.26,27 With the development of lower-osmolality RCM, the overall risk of anaphylactoid reactions has decreased.28
Systemic allergic reactions to insect stings are reported by up to 3% of adults, and almost 1% of children who have been stung.29,30 At least 50 fatal reactions to an insect sting occur each year in the United States. Half of these occur in individuals who had no history of a previous reaction to an insect sting.31
It has been estimated that the overall incidence of latex allergy in the United States ranges between 2.7 million and 16 million. Although the incidence of latex allergy has risen markedly over the last 15 years, with the reduction of the use of powdered gloves and the substitution of nonlatex gloves in hospitals, the incidence of latex allergy appears to have stabilized and perhaps declined.32 Populations at risk are those experiencing multiple mucosal exposures to latex such as subjects who have had multiple catheterizations, multiple surgeries, and of course health care workers.
Exercise-induced anaphylaxis (EIA) is a rare disorder. One study estimated the prevalence of EIA among Japanese adolescents to be approximately 0.03%, with no clear gender preference.33 Exercise-induced anaphylaxis exists in two forms. In one form the act of exercise alone is sufficient to produce an event, and in another form exercise plus a cofactor such as the ingestion of a food or drug is required. The prevalence of patients with purely exercise-triggered anaphylaxis, relative to those who require exercise plus a cofactor, is not known.34 Foods are the most frequently reported cofactor.35 NSAIDs and aspirin are the most frequently reported drug cofactor.36,37 Other cofactors include alcoholic beverages, menstruation, and exposure to pollen during exercise.36,38
The cause of anaphylactic events remains unidentified in as many as two-thirds of adults presenting to an allergist/immunologist for evaluation of anaphylaxis. A survey of 75 allergists in the United States found that these physicians had encountered 633 cases. The authors extrapolated these data to the population of the USA and estimated there are as many as 20,592 to 47,024 cases.14
CAUSES OF ANAPHYLAXIS IN THE CRITICAL CARE UNIT OR OPERATING ROOM
The incidence of anaphylaxis during anesthesia has been reported to range from 1 in 4000 to 1 in 25,000.20 Neuromuscular blocking agents are responsible for 60% to 70% of anaphylactic reactions during general anesthesia.39-41 In most series, succinylcholine is the most frequently cited agent but this may vary depending on the practice pattern, which determines the agent used. Most of the neuromuscular blocking agents cause direct mast cell degranulation and histamine release which are IgE independent. However, life-threatening reactions to these agents usually are IgE mediated.42 The tertiary or quaternary ammonium group, common to all muscle relaxants, is likely the immunodominant determinant recognized by IgE.43 The antigenicity of the shared ammonium structures may be responsible for cross-reactivity among the muscle relaxants. Cross-reactivity occurs most consistently between pancuronium and vecuronium.44 Cross-reactions also may occur between muscle relaxants and other classes of pharmaceuticals, based on in vitro inhibition of specific-IgE binding to the muscle relaxants. Agents that potentially cross-react with muscle relaxants include acetylcholine, choline, morphine, neostigmine, and pentolinium.45 Cross-inhibition studies suggest that previous exposure to these nonanesthetic drugs may sensitize individuals to muscle-relaxing agents, resulting in reactions among patients without prior anesthesia. Three out of four cases of anaphylaxis to muscle relaxants occur in females, suggesting cross-reactivity with ammonium compounds in personal care products.46 Skin testing may be useful to determine the safest alternative for subsequent anesthesia following a suspected reaction, recognizing that nonimmunologic reactions are not identified by this diagnostic method.47,48 Skin testing is not recommended for preanesthetic screening of subjects without a history of a previous reaction.49
The antibiotics most commonly implicated in reactions during this period are β-lactam antibiotics and vancomycin.50 Rapid vancomycin administration may result in life-threatening, non-IgE-mediated anaphylaxis.51-53 These nonimmunologic reactions to vancomycin can be reduced or eliminated by administering this drug as a dilute solution, dissolved in at least 200 mL, and infused over at least a 2-hour period. IgE-mediated anaphylaxis to vancomycin is much less common.
Dextran and hydroxyethyl starch (HES), large-molecular-weight polysaccharides, may be used as a nonblood, high oncotic fluid replacement during surgery. These agents are infrequently associated with adverse reactions and anaphylaxis. Estimates of reaction rates are 0.008% to 0.08% for dextran and 0.08% for HES.54
Intravenous drugs used for anesthetic induction can cause perioperative anaphylaxis. Barbiturates, especially thiopental, have been reported to cause anaphylaxis. Most of the adverse reactions with barbiturates, particularly thiopental, are caused by specific IgE antibody, though this agent can also cause direct mast cell degranulation.46,55-57 Propofol, a nonbarbiturate induction agent, has also been reported to cause perioperative anaphylaxis through both an IgE-mediated mechanism and direct histamine release.58-60
Opiates used in the perioperative period are a common cause of flushing and urticaria following intravenous administration. Anaphylaxis to these agents, in contrast, is very rare.61,62 Cutaneous flushing and hives often occur after intravenous morphine administration, but with rare exceptions, the amount of histamine release does not result in hypotension or bronchospasm.63 Reducing the rate of opioid administration usually limits the severity of these reactions. Fentanyl does not directly stimulate histamine release by way of the mast-cell opioid receptor.63 Intravenous protamine, an agent used to reverse heparin anticoagulation, may cause both anaphylactic and anaphylactoid reactions; the latter is characterized by increases in pulmonary artery pressure. Potential pathophysiologic mechanisms are numerous and varied.64-68 A case-controlled study showed that previous neutral protamine Hagedorn (NPH) insulin use, fish allergy, and other medication allergy are independent risk factors for anaphylaxis to protamine.67 It has been estimated that up to 39% of cardiopulmonary bypass patients have one or more of these risk factors. Latex has been reported to account for up to 17% of intraoperative anaphylaxis.69
The features of intraoperative anaphylaxis may differ considerably from anaphylaxis not associated with surgical procedures. While cutaneous, hypotensive and respiratory events occur in both, hypotensive cardiovascular collapse is a more predominant feature of reactions during surgery.70 Latex-induced anaphylaxis is due to IgE-mediated mechanisms. Unfortunately, no standardized skin test reagent for latex is available in the United States. For diagnostic purposes, in vitro tests for latex-specific IgE are available, but the sensitivity of these tests may vary. Due to the suboptimal diagnostic utility of these tests, results must be carefully correlated with the clinical history. Latex-induced anaphylaxis may occur in a variety of situations, all involving direct contact with latex, usually gloves, or instruments, or with aerosolization of latex antigen adherent to the cornstarch powder of latex gloves. Thus, latex reactions can occur during operative procedures when gloves are donned. Latex reactions may occur immediately with latex contact or may be delayed from 30 to 60 minutes. Intraoperative latex anaphylaxis may be related to the administration of drugs through a latex port prior to surgery.
PATHOPHYSIOLOGY
The mechanisms underlying anaphylactic reactions can be broadly divided into immunologic and nonimmunologic categories (Table 128-2). Immunologic reactions can be further subdivided into IgE-mediated and non–IgE–mediated events. There are agents that can cause anaphylaxis through several mechanisms (eg, radiocontrast agents can rarely trigger anaphylaxis through IgE-mediated mechanism as well as via direct mast cell degranulation.)
Mast cell and basophil degranulation are the primary events in anaphylaxis. Anaphylaxis commonly involves an immunologic mechanism in which IgE is synthesized in response to allergen exposure and becomes fixed to high affinity receptors for IgE (FcERI receptors) on the surface membranes of mast cells and basophils. Aggregation of receptor-bound IgE molecules occurs on reexposure to the allergen and results in cell activation and mediator release.71-73 IgE also contributes to the intensity of anaphylaxis by enhancing the expression of FcERI on mast cells and basophils.71-73 Other immunologic mechanisms that do not involve IgE can cause anaphylaxis.74 IgG-mediated anaphylaxis has been reported due to high-molecular-weight iron dextran and the infusion of chimeric, humanized, or human therapeutic mAbs, such as infliximab.75,76 Complement-mediated anaphylaxis occurs in association with hemodialysis, the use of oversulfated chondroitin sulfate (OSCS)–contaminated heparin, protamine neutralization of heparin, and the administration of liposomal drugs and polyethylene glycols.77 OSCS-contaminated heparin triggers anaphylaxis through activation of the complement system as well as via activation of the contact system. This results in the formation of anaphylatoxins C3a and C5a and kinin.77,78 Direct activation of the innate immune system might also produce anaphylactic events.79 In addition, as noted previously, nonimmune activation of mast cells and basophils occurs.16,80,81 A trigger can lead to anaphylaxis through more than one mechanism; for example, radiocontrast media can rarely trigger anaphylaxis through an immunologic IgE-dependent mechanism as well as through direct mast cell activation.81,82 Regardless of the underlying mechanisms, mast cells and basophils may play an important role in initiating and amplifying the acute allergic response. Calcium influx is the essential proximal intracellular event leading to mast cell degranulation and is controlled by both positive and negative regulation through calcium channels.71,83 Mast cells and basophils release preformed chemical mediators of inflammation, including histamine, tryptase, carboxypeptidase A, and proteoglycans.71,72,84-86 They also release newly generated mediators, such as leukotrienes, prostaglandins, platelet-activating factor, and cytokines, such as IL-6, IL-33, and TNF-α.71,72,84,87-90 Sphingosine-1-phosphate is now recognized as a circulating mediator in anaphylaxis, and in addition, it acts as a signaling component within the mast cell.91
The clinical manifestations are the result of the activities of the mediators released from the mast cell and basophils (Table 128-3). These mediators not only exert direct effects on the target organs, but also recruit other inflammatory cascades including the complement system, the contact system, and the clotting cascade. These recruited pathways can amplify the severity of the event and change the nature of the pathophysiology (Table 128-4).
Anaphylactic Mediators and the Resultant Pathophysiologic Activities
Mediator | Pathophysiologic Event | Clinical Correlate |
---|---|---|
Histamine and products of arachidonic acid metabolism (leukotrienes, thromboxane, prostaglandins, and platelet-activating factor) | Smooth muscle spasm, mucus secretion, vasodilatation, increased vascular permeability, activation of nociceptive neurons, platelet adherence, eosinophil activation, eosinophil chemotaxis | Wheeze, urticaria, angioedema, flush, itch, diarrhea and abdominal pain, hypotension, rhinorrhea, and bronchorrhea |
Neutral proteases: tryptase, chymase, carboxypeptidase, cathepsin G | Cleavage of complement components, chemoattractants for eosinophils and neutrophils, further activation and degranulation of mast cells, cleavage of neuropeptides, conversion of angiotensin I to angiotensin II | May recruit complement by cleaving C3, may ameliorate symptoms by invoking a hypertensive response through the conversion of angiotensin I to angiotensin II and by inactivating neuropeptides. Also, can magnify response due to further mast cell activation |
Proteoglycans: heparin, chondroitin sulphate | Anticoagulation, inhibition of complement, binding phospholipase A2, chemoattractant for eosinophils, inhibition of cytokine function, activation of kinin pathway | Can prevent intravascular coagulation and the recruitment of complement. Also can recruit kinins increasing the severity of the reaction |
Chemoattractants: chemokines, eosinophil chemotactic factors | Calls forth cells to the site | May be partly responsible for recrudescence of symptoms in late phase reaction or extension and protraction of reaction |
Nitric oxide | Smooth muscle relaxation causing vasodilatation of peripheral vascular bed, bronchodilatation, and coronary artery vasodilatation. In addition, nitric oxide causes increased vascular permeability | Perhaps relief of bronchospasm, but most important effect appears to be the production of hypotension and shock |
Tumor necrosis factor-α activates NFK-β | Production of platelet-activating factor | Vascular permeability and vasodilatation. Also since it is synthesized and released “late,” has been incriminated in production of late phase reactions |
Interleukins 2, 6, 10 | These are usually found later in the course of an event than histamine and tryptase, and persist longer. The effects they cause have not been determined. IL-10 may be active in recovery from events | Unknown |
Tumor necrosis factor receptor-1 | Unknown | Elevated levels have been associated with more profound hypotension |
Recruitment of Other Inflammatory Cascades During Anaphylaxis
Mediator | Pathophysiologic Event | Clinical Correlate |
---|---|---|
Activation of the contact system (kinins) | Vasodilatation and vasopermeability | Hypotension and angioedema |
Activation of the complement system | C3a/C5a can cause vasopermeability | Possible urticaria/angioedema |
Activation of the clotting system (Factor XI, plasmin) | Intravascular coagulation | Disseminated intravascular coagulation |
Histamine is one of the important mediators. Histamine acts on the smooth muscle cells of the bronchi, coronary arteries, and the GI tract. It leads to smooth muscle spasm, increased vascular permeability, vasodilation, stimulation of sensory nerve endings, and myocardial depression. The clinical effects present as wheezing, hypotension, nausea, vomiting, diarrhea, and myocardial ischemia, as well urticaria and angioedema. Histamine binds to four different receptors. Anaphylactic events are mostly mediated through activation of the H1 and H2 receptors. Vasodilation is the primary event of histamine stimulation and is mediated through both H1 and H2 receptors. Vasodilation is the direct effect of H2 receptor stimulation on the vascular bed, whereas H1 stimulation causes stimulation of endothelial cells and the production of nitric oxide (NO), which in turn leads to vasodilation. Vasodilation produces flushing and lowers peripheral resistance and blood pressure. Smooth muscle contraction in the bronchial tree and GI tract is mediated primarily through the H1 receptor and causes wheezing, cramping abdominal pain, and diarrhea. Cardiac effects and the increase in glandular secretions are mediated through both the H1 and H2 receptors. H3 receptors are present in presynaptic terminals of sympathetic nerves innervating the heart and other systemic vasculature. Their stimulation leads to inhibition of norepinephrine release. Blockade of the H3 receptor would be potentially beneficial by correcting hypotension through restoration of the release of norepinephrine.92
In addition, many of these mediators are capable of activating other inflammatory pathways (Fig. 128-1). Mast cell kininogenase and basophil kallikrein can activate the kinin system. Tryptase also has kallikrein activity and can activate the complement cascade and cleave fibrinogen. Platelet-activating factor induces clotting and disseminated intravascular coagulation. In addition, chemotactic agents, by recruiting eosinophils and other cells, have the capacity to prolong and intensify reactions. Heparin can inhibit clotting, plasmin, and kallikrein. It also modulates the effects of tryptase and has anticomplementary activity. Chymase is capable of converting angiotensin I into angiotensin II and therefore theoretically could enhance the compensatory response to hypotension. Cells, especially eosinophils, called forth to the site by chemotactic agents originally released from mast cells and basophils, can be responsible for protracted episodes of anaphylaxis and for a recrudescence in symptoms after an initial improvement (late-phase response).
Nitric oxide (NO), synthesized from L-arginine by nitric oxide synthase, has an important role in the pathophysiological changes associated with anaphylaxis.93 NO production can be increased in anaphylaxis. This has been demonstrated in a rabbit model of anaphylaxis and human anaphylactic events.93,94 NO can play a dual role in anaphylaxis. While it prevents mast cell mediator release and dilates bronchial smooth muscle, it simultaneously can cause vasodilation and enhance vascular permeability. Nitric oxide synthase inhibitor attenuates hypotension and hemoconcentration and decreases venous return but does not improve cardiac depression. In animals pretreated with a NO synthase inhibitor, the cardiac output falls significantly, although venous return is increased. However, the role of NO during anaphylaxis has been questioned as well. In one study, there was no correlation between nitric oxide level, plasma histamine and serum tryptase levels. There was also no correlation between nitric oxide levels and urticaria or erythema, and the levels were not higher in patients with bronchospasm and hypotension.95
The hemodynamic abnormalities during anaphylaxis result from the loss of intravascular fluid and vasodilation and are often followed by vasoconstriction and then myocardial depression. Up to 50% of vascular volume can be lost into the extravascular space within 10 minutes secondary to increased vascular permeability.96,97 The intravascular fluid loss triggers compensatory mechanisms (Fig. 128-2)which includes the endogenous production of various vasoconstrictors, for example, epinephrine, norepinephrine, angiotensin, and endothelin-1.61,98-100
The early fall in arterial pressure (vasodilation) may lead to a brief and temporary increase in cardiac output due to left ventricular unloading or an increase in cardiac contractility (effects of epinephrine, norepinephrine, and histamine). Subsequently, the loss of plasma volume, the decrease in venous return, and the pooling of blood in the splanchnic circulation will decrease cardiac output, causing shock. Transient pulmonary hypertension and increased pulmonary vascular resistance has been observed in animal models of anaphylaxis. The high albumin concentration in pulmonary edema fluid and the low pulmonary artery wedge pressures indicate that the pulmonary edema in anaphylaxis is noncardiogenic and likely due to increased microvascular permeability.101,102
CLINICAL PRESENTATION
The signs and symptoms of anaphylaxis are summarized in Table 128-5. This table is based on a compilation of 1865 patients and includes patients with idiopathic anaphylaxis, exercise-induced anaphylaxis, and other causes of anaphylaxis.20 Cutaneous manifestations are most common and occur in more than 90% of cases.20 The cutaneous symptoms include urticaria, angioedema, flushing, and pruritus. Over 90% of adults experience cutaneous manifestations. However, the incidence of cutaneous manifestations in children may be lower.103,104 Cardiovascular symptoms, for example, dizziness, syncope, arrhythmia, and hypotension occur in about 30% to 35% of cases. Severe episodes characterized by rapid cardiovascular collapse and shock can occur without cutaneous manifestations.105,106 In fact, in a series of 27 severe episodes, only 70% of patients with circulatory and/or cardiovascular collapse had cutaneous manifestations.107 Reflex tachycardia occurs commonly secondary to hypovolemia during anaphylaxis. This is a useful differentiating feature to distinguish anaphylaxis from a vasovagal reaction. However, bradycardia has also been described during anaphylaxis. It can occur secondary to increased vagal reactivity mediated through unmyelinated vagal C-fibers which are activated by ischemia. Brown et al studied 21 adults with history of systemic insect sting allergy who were challenged with stings in controlled settings. Eighteen individuals developed systemic reactions. Hypotension accompanied by bradycardia developed in two individuals.108 Allergic reactions can trigger not only anginal episodes, but also acute myocardial infarction.109 This was first reported in the American Heart Journal in 1950 with a case of a prolonged allergic reaction to penicillin.110 In 1991, Kounis and Zavras described the Kounis syndrome112 as chest pain during an anaphylactic reaction. The chest pain can present as classical angina pectoris. Myocardial infarction with normal coronary arteries can occur due to this phenomenon.111-113 The Kounis syndrome has been divided into two subtypes. In type I, chest pain occurs without coronary artery disease during an acute allergic reaction in patients without predisposing factors for coronary artery disease. These cases have a normal myocardial perfusion scan and normal coronary angiogram.114 These cases are thought to be due to endothelial dysfunction or microvascular angina.115 In type II there is preexisting coronary artery disease.113,114 This syndrome has been mostly reported in adults but can be seen in children as well.116 The clinical presentation of KS includes a mixture of symptoms and signs of an allergic reaction and acute coronary syndrome, with chest pain, dyspnea, faintness, nausea, vomiting, syncope, pruritus, urticaria, diaphoresis, pallor, palpitations, hypotension, bradycardia.117 Respiratory complaints, for example, wheeze, dyspnea, stridor, and rhinitis are seen in 40% to 60% of cases. Arterial blood gas abnormalities usually consist of a fall in [Math Processing Error] and [Math Processing Error] early in the course. If severe respiratory difficulty supervenes, the hypoxia worsens and an elevation of [Math Processing Error] may occur, along with a fall in pH that is probably due to a combination of carbon dioxide retention and metabolic acidosis. Other unusual presentations include syncope which can occur alone or associated with seizures. Syncope has been reported with anaphylaxis resulting from insect sting, fire ant, and mastocytosis.10 Profound anaphylaxis with hypotension can result in fibrinolysis and disseminated intravascular coagulation. Tranexamic acid can rapidly reverse the coagulopathy.118
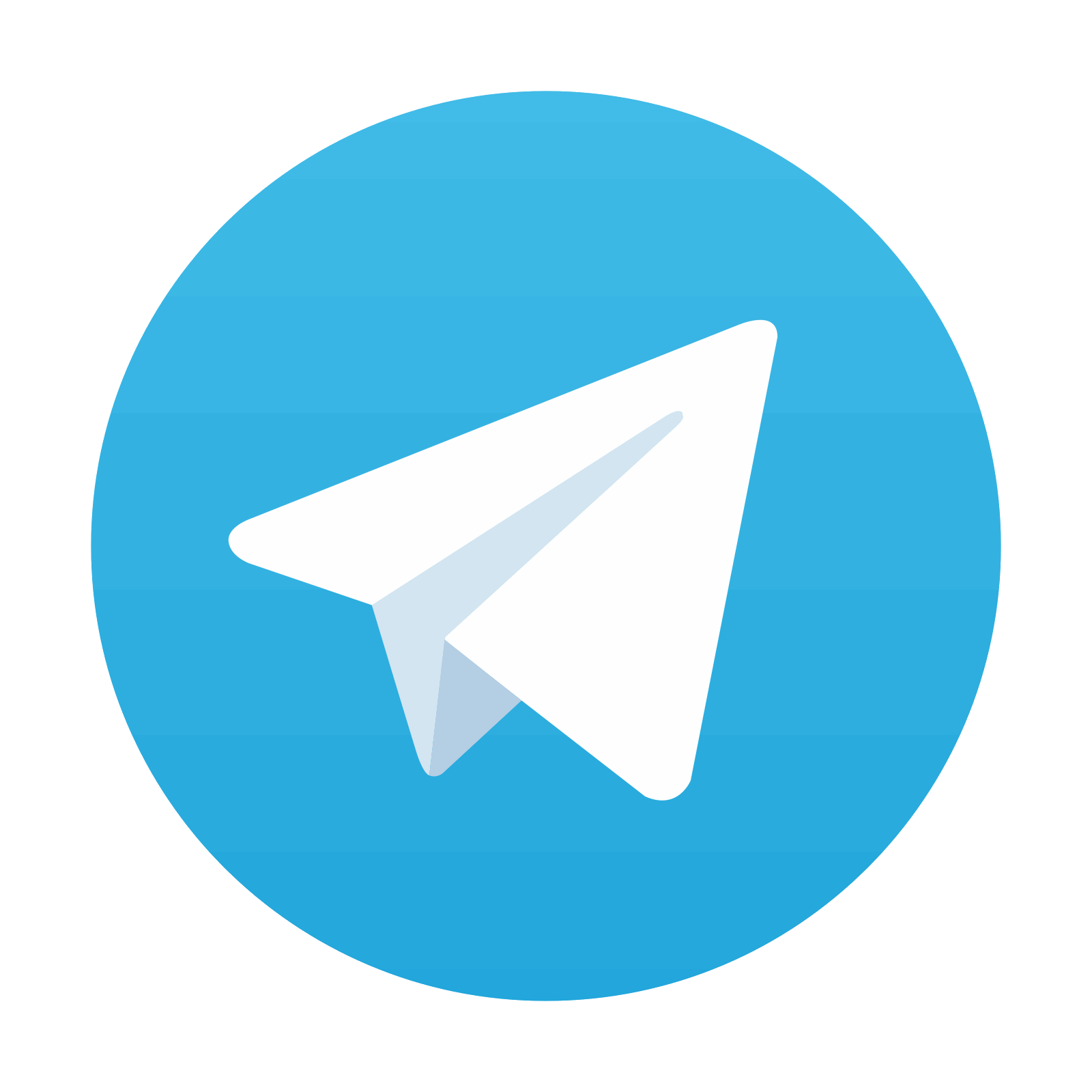
Stay updated, free articles. Join our Telegram channel

Full access? Get Clinical Tree
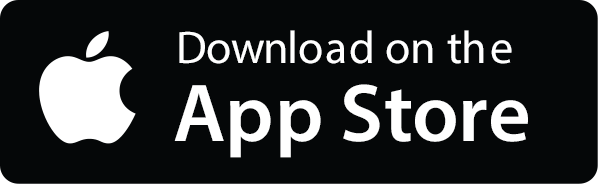
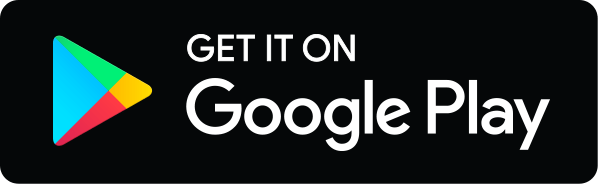