Wash-in curves for volatile anaesthetic agents
Partial pressure of volatile agent in the pulmonary artery
The rate of uptake of volatile agent from each alveolus is dependent on the tension difference between the alveolus and the capillary blood. As the concentration and tension in the blood rises the rate of uptake is reduced and so the rate of tension rise decreases.
Pulmonary blood flow
As blood passes through the pulmonary capillaries the tension of volatile agent in the capillary will increase and so reduce the rate of transfer in the latter part of the capillary. Therefore, increasing the blood flow will increase uptake.
Ventilation/perfusion distribution
Ventilation/perfusion mismatch will reduce perfusion of well-ventilated areas of lung and increase perfusion of alveoli poorly supplied with volatile agent.
Concentration effect
The uptake of volatile agent from the alveolus, during a small part of the respiratory cycle, reduces the amount left in the alveolus for subsequent parts of the cycle. Rate of uptake is proportional to the tension, which is the direct result of the concentration. Assuming that a smaller proportion of the other constituents of alveolar gas (e.g. nitrogen and oxygen) is absorbed, then the concentration of the anaesthetic agent will fall. The degree of fall will be influenced by its concentration, agents in low concentrations suffering a greater proportional loss than those in high concentrations. This means that the uptake of agents inhaled in high concentrations will be better maintained over the respiratory cycle than those administered at lower concentrations.
Second gas effect
The second gas effect embodies the same principles as the concentration effect. However, in this case the administration of a rapidly absorbed gas given in high concentration (typically nitrous oxide), together with a volatile agent of lower solubility, produces an increasing alveolar concentration of the second agent, thus promoting its absorption.
Circulatory phase
The following factors influence the transport of the dissolved volatile agent to the brain:
Cardiac output
Cerebral blood flow
Distribution to other tissues
The distribution of blood to various tissues and compartments is described elsewhere (see Chapter 27, Distribution). While these principles apply to drugs that are intravenously administered, volatile agents behave in a similar fashion, with the driving force of partial pressure (tension) tending toward equilibrium. The uptake of agent by the tissues is proportional to tissue perfusion, solubility and arteriovenous tension difference. The formula is:
The formula produces time constants for the exponential function of tissue anaesthetic tension against time. High cardiac output and low tissue solubility produce a low time constant.
Cardiac output
Of cardiac output, 70–80% is distributed to the vessel-rich organs (brain, heart, liver, kidney) that constitute about 9% of body mass. Of the total, 14% goes to the brain (2.2% of body mass). A large proportion of the absorbed anaesthetic is thus directed to the brain. By virtue of their high lipid solubility, the brain has a relatively high affinity for anaesthetic agents.
Cerebral blood flow (CBF)
Factors affecting the proportion of cardiac output going to the brain will influence cerebral uptake of volatile agents. In shock, CBF is relatively well preserved, and therefore more agent will go to the brain and equilibrium will be reached more rapidly. Increasing depth of anaesthesia during spontaneous ventilation increases CO2 and secondarily CBF. Hyperventilation during induction of anaesthesia will reduce CO2 and CBF and delay equilibrium.
Distribution to other tissues
Distribution of volatile agent to other tissues (dependent on the factors above) slows the initial rate of uptake by the brain. Later, these depots of anaesthetic agent act to maintain the blood and so brain levels, and they act as a damper to any changes in alveolar and blood levels. This is something of an advantage, but slows recovery as much as it does induction.
Minimum alveolar concentration (MAC)
Minimum alveolar concentration may be defined as the concentration of anaesthetic agent which at equilibrium will prevent a reflex response to skin incision in 50% of subjects. MAC acts as a guide to the concentration required for anaesthesia. Altitude reduces atmospheric pressure, and therefore reduces the concentration of anaesthetic agent for a given percentage, so minimum alveolar pressure might be a more useful concept. It is a form of ED50 (effective dose). Other measures of potency include AD95 (anaesthetic dose), which is the dose required to prevent response to surgical stimulus in 95% of subjects. Various factors affect MAC for a particular agent, e.g. species, age and concomitant drugs. In clinical practice, it is important to remember that the displayed MAC value on an anaesthetic monitor is calculated from a standard MAC for the identified volatile(s), and does not measure neuronal tissue levels.
The mechanism of action of anaesthetic agents is described in Chapter 28.
The ideal volatile agent
Although the ideal volatile agent does not exist, nonetheless it remains a useful concept. The characteristics of an ideal agent are given in Figure 29.2. A comparison of the physical properties of the major agents in clinical practice is given in Figure 29.3.
Physical Liquid at room temperature Low latent heat of vaporisation Low specific heat capacity SVP sufficiently high to allow for easy vaporisation Stable in light Stable at room temperature Non-flammable Inexpensive Environmentally safe (locally and globally) |
Pharmacological Pleasant smell Low blood/gas solubility Potent enough to provide surgical anaesthesia without supplement (low MAC) High oil/water solubility Analgesic effect Non-epileptogenic No cardiac irritability No cardiovascular depression No respiratory depression Non-irritant to airways Muscle relaxation No increase in intracranial pressure (ICP) Unaffected by renal failure Unaffected by hepatic failure Minimal metabolism |
MW (daltons) | BP at 1 atm (°C) | SVP at 20 °C (kPa) | MAC (% v/v) | Ostwald solubility coefficients (dimensionless) a | ||||||
---|---|---|---|---|---|---|---|---|---|---|
O/W | BI/G | W/G | Br/G | O/G | Br/BI | |||||
Desflurane | 168 | 22.8 | 88.5 | 6.35 | N/A | 0.42 | N/A | 0.54 | 19 | 1.3 |
Enflurane | 184.5 | 56.5 | 22.9 | 1.68 | 120 | 1.9 | 0.78 | 2.6 | 98 | 96 |
Halothane | 197 | 50.2 | 32.5 | 0.75 | 220 | 2.3 | 0.8 | 4.8 | 224 | 1.9 |
Isoflurane | 184.5 | 48.5 | 31.9 | 1.15 | 174 | 1.43 | 0.62 | 21 | 91 | 1.6 |
Sevoflurane | 200 | 58.6 | 21.3 | 2.00 | N/A | 0.69 | N/A | 1.1 | 53 | 1.7 |
Nitrous oxide | 44 | – 88 | 5300 | 105 | 3.2 | 0.47 | 0.44 | 1.4 | 1.0 | |
Xenon | 131.2 | –108 | 71 | 19 | 0.12 | 0.075 | 1.8 | 0.17 | ||
Ethyl chlorideb | 64.5 | 13 | 132 |
Specific pharmacology
The following agents will be considered in more detail: desflurane (D), halothane (H), isoflurane (I) and sevoflurane (S). Chemical structures of these four, as well as enflurane and nitrous oxide, are shown in Figure 29.4. In the comparative lists for each agent, molecular weight (MW) is given in daltons, boiling point (BP) in degrees Celsius, MAC in volumes %, and the coefficients are dimensionless.
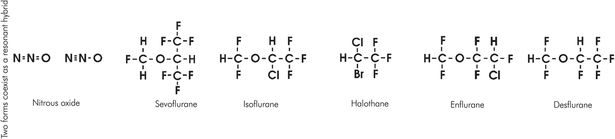
Chemical structures of volatile agents
General features
Physical
Physically, the volatile anaesthetic agents are relatively small, simple molecules, generally denser than water. They rely on physical as well as pharmacological features to achieve their effect. For a particular carbon skeleton, changes in halogenation alter both physical and pharmacological properties. This is best demonstrated by the methyl ethyl ether skeleton common to desflurane and isoflurane.
Solubility is reduced with lowering of molecular weight. Fluoride ions are lighter than chloride and bromide ones, and thus desflurane, having fluoride ions in place of chloride, is comparatively less soluble than isoflurane. Potency increases with increasing molecular weight, and the lighter desflurane is less potent than isoflurane. This may be related to the strong correlation of oil/water solubility with pharmacological potency (see Chapter 28).
Increasing fluorination of a carbon skeleton increases saturated vapour pressure and stability but reduces boiling point, flammability and toxicity. Methyl ethyl ether is flammable, but desflurane, enflurane and isoflurane are not.
Clinical
The comparative clinical properties of the major volatile agents are summarised in Figures 29.5 and 29.6.
Worst | Worse | Better | Best | |
---|---|---|---|---|
Induction | D | I | H | S |
Cardiovascular stability | H | I | D | S |
Respiratory irritation | D | I | H & S | |
Ease of titration | H | I | S | D |
Emergence | H | I | S | D |
Metabolism/toxicity | H | S | I | D |
Halothane | Isoflurane | Desflurane | Sevoflurane | |
---|---|---|---|---|
Pungency | ●○○○ | ●●●○ | ●●○○ | ○○○○ |
Respiratory irritation | ○○○○ | ●●○○ | ●●●○ | ○○○○ |
Respiratory depression | ●●○○ | ●●●○ | ●●●● | ●●○○ |
Cardiovascular depression | ●●●○ | ●●○○ | ●○○○ | ●○○○ |
Coronary vasodilatation | ●○○○ | ●●○○ | ●○○○ | ●●○○ |
Muscle relaxation | ●●○○ | ●●●○ | ●●●○ | ●●●○ |
Intracranial pressure elevation | ●●●● | ●●○○ | ●●●○ | ●●○○ |
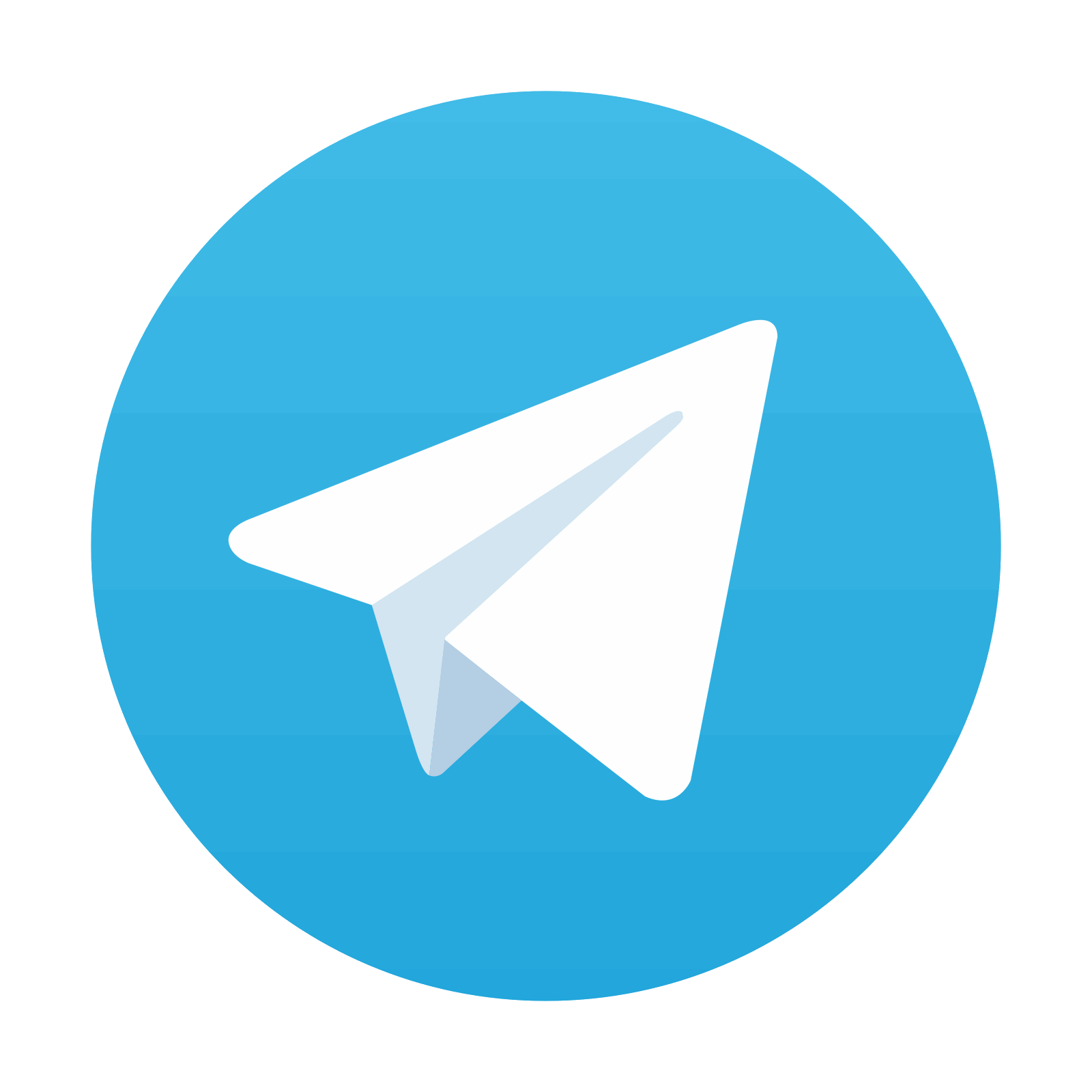
Stay updated, free articles. Join our Telegram channel

Full access? Get Clinical Tree
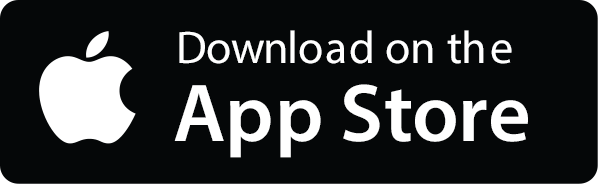
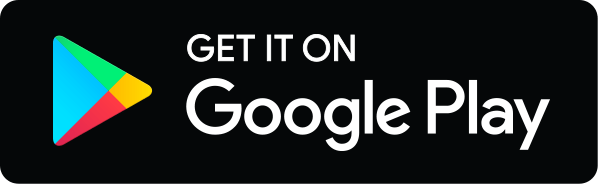