A 58-year-old Asian male with no significant past medical history presents with acute herpes zoster in the left T4 dermatome. Presenting signs and symptoms include severe localized pain and tactile allodynia associated with a vesicular eruption. Even after the rash resolves, 3 months later he continues to complain of severe burning pain in addition to allodynia and hyperalgesia in the left T4 dermatomal region, suggesting that he has developed post-herpetic neuralgia (PHN). Ten milligrams of nortriptyline, a tricyclic antidepressant (TCA), is prescribed with a plan to titrate up to a higher dose to achieve effective pain relief if the initial 10-mg dose is not helpful enough. Unfortunately, he is unable to tolerate the initial dose because he quickly develops intolerable adverse effects (sedation, orthostatic hypotension, and dry mouth) shortly after he begins taking it. Tramadol and then hydrocodone/acetaminophen are prescribed but these are also poorly tolerated. He is then prescribed gabapentin at an initial dose of 300 mg taken at night, which is titrated over several weeks to an effective analgesic dose for PHN: 1800 mg/day in three divided doses. Although the burning pain is lessened, it is still not sufficiently controlled and the patient returns for further treatment. From the time of presentation for treatment, it has taken over 6 weeks for him achieve any notable degree of pain reduction.
This patient was treated according to available evidence-based, current guidelines, yet he did not obtain adequate analgesic benefit from the prescribed medications. Clinicians recognize that individual variations in responses to pain itself, as well as in the response to pharmacotherapies for pain relief, are routinely observed; however, advances in pharmacogenomics may allow us to better understand why patients may have variable responses to various analgesic therapies and may soon allow an opportunity for clinicians to identify the most appropriate pharmacotherapies for a patient before prescribing and hence improve patient-specific outcomes. The knowledge and use of relevant pharmacogenomic information may indeed help a clinician to truly individualize a patient’s care, an example of personalized health care.
In this example, the patient’s treatment responses require a careful evaluation of possible reasons for them. Variability in patient response, including intolerable adverse effects, can be due to nonadherence with the prescribed medication or medication schedule, a drug interaction inhibiting the medication’s effectiveness, mechanisms of pain generation that fail to respond to the prescribed medication’s mechanism of action, or a combination of these. It is also possible that the patient’s genetic background has affected his ability to clinically respond to or metabolize the prescribed medications, and this is where the emerging field of pharmacogenomics becomes important to recognize. This patient example illustrates the variable analgesic response and adverse event profile when analgesics are prescribed. As noted, clinicians are only too well aware how often individuals may not obtain the expected therapeutic benefit from analgesic medications. The response rates in well-designed studies of various analgesic therapies are approximately 50% to 60%; this “treatment success” rate is similar to trials of therapies used to treat congestive heart failure and epilepsy. Many key areas of medicine are similarly affected by poor responder rates in clinical trials of diverse drug types. Knowledge of relevant pharmacogenomic factors that may influence the effect of a prescribed analgesic should allow clinicians to improve analgesic treatment outcomes.
The Impact of Pharmacogenomic Research on Clinical Medicine
Pharmacogenomics is the study of the impact of genetics on pharmacotherapeutic responses and tolerability, in an effort to improve drug safety and efficacy through genetically guided, individually tailored treatments. As noted previously, the same drug can be associated with different clinical responses among a group of individuals. This is because each individual’s unique genetics provides the genetic blueprint for the generation of singular protein expression profiles, which subsequently leads to functional variations observed as clinical responses. Indeed, taking into account such genetic predisposition and receptor subtype expression has the potential to result in a more satisfactory reduction in symptoms or resolution of disease. This approach is certainly at the core of so-called personalized medicine. When the clinician is aware of a patient’s genetic predisposition and receptor subtype expression, this knowledge may lead to more individually focused prescribing with improved efficacy and safety for the patient.
Ongoing research on the genetic determinants of various diseases have been derived from the Human Genome Project, including The SNP Consortium (TSC). Single nucleotide polymorphisms (SNPs; pronounced “snips”) are the most important contributors to the observed regular variation in the genetic code, which lead to the human population’s diversity of appearance and, at a deeper level, to our diversity in physiology. SNPs are defined as deoxyribonucleic acid (DNA) sequence variations resulting from a single nucleotide (adenine [A], thymine [T], cytosine [C], or guanine [G]) change in the genome sequence. As an example, the most commonly observed change is when a C is replaced by a T, as in when the sequence ACGGCTAA is changed to ATGGCTAA. Overall there are approximately 9 million to 10 million common SNPs. In many cases, this minimal change amid a background of the approximately 3.3 billion base pairs of DNA contained in one somatic cell does not lead to any significant observable change in an individual. Many of these DNA changes do not translate into differences at the protein level. However, there are SNPs that in fact do lead to phenotypic differences, and some single base pair changes do affect a noticeable, clinically relevant change in phenotype. Unique SNPs have been identified that lead to lower activity of various enzymes, red hair color and fair skin, and a lack of or diminished expression of one of the red blood cell antigens.
The most commonly observed DNA sequences of a gene are called “wild-type,” and the less common alleles are “variants” if they are prevalent in more than 1% of the population. The even less prevalent alleles have another name; DNA sequences in genes found in less than 1% of the population are called “mutations.” For example, within pain management, an autosomal dominantly inherited peripheral neuropathy, hereditary sensory, and autonomic neuropathy (HSAN) type I confers insensitivity to pain mediated through the progressive degeneration of the dorsal root ganglia (DRG) and motor neurons as the result of mutations within a specific location in chromosome 9. Individuals with this genetic background cannot sense pain, making them particularly prone to painless but potentially severe injuries, with detrimental complications, such as chronic skin ulcers and distal amputations. Additional types of changes to our DNA can occur, such as deletions and insertions, which also can result in catastrophic consequences. Large population studies have analyzed hundreds of thousands of SNPs in an attempt to establish the genetic linkages to a diversity of diseases. The Wellcome Trust Consortium published a particularly important study in the June 2007 issue of Nature . The authors assessed the genetic influence on seven major diseases by comparing the SNPs of 14,000 patients (2000 with each disease to those of 3000 healthy patients). Genomic hot spots (regions of DNA connected to biologic functions/diseases) were linked to a susceptibility to developing bipolar disorder, Crohn’s disease, coronary artery disease, diabetes (both type 1 and type 2), hypertension, and rheumatoid arthritis. Key terms used in pharmacogenomics can be viewed in Table 11.1 .
Genome | The entire collection of genetic information (or genes) that an organism possesses |
Genotype | The genetic constitution of an individual, either overall or at a specific gene |
Heterozygous | The presence of two different alleles (one on the maternal chromosome and one on the paternal chromosome) at a gene location |
Homozygous | The presence of two identical alleles at a gene location |
Isoform | A variant in the amino acid sequence of a protein |
Messenger RNA | An RNA-containing single-strand copy of a gene that migrates out of the cell nucleus to the ribosome, where it is translated into a protein |
Mutation | A rare variant in a gene, occurring in less than 1% of a population |
Pedigree | A diagram depicting heritable traits across two or more generations of a family |
Phenotype | The observable characteristics of a cell or organism, usually resulting from the product coded by a gene (genotype) |
Polymorphism | The existence of two or more variants of a gene in a population, with at least 1% frequency of the less common variant |
Prodrug | A medication that is inactive until it is converted enzymatically to its active metabolite |
Single-nucleotide polymorphism (SNP) | A single base-pair change in a DNA sequence compared with the “common” or “wild-type” sequence |
Variant allele | The allele at a particular SNP that is the least frequent in a population |
Wild-type allele | The allele at a particular SNP that is most frequent in a population, also called “common” allele |
The integration of genetic information into clinical practice has far-reaching implications. For example, the clinical integration of genetic information has been increasingly used in oncology to guide treatment. Other areas of medicine are also beginning to tailor pharmacotherapies based on an individual’s genetic information, and certain biomarkers are now known that can help to guide treatment in many areas of medicine including pain management. Pharmacogenomic testing has benefited patients and clinical medicine in general by improving the ability to stratify the risks of various treatments for individual patients by identifying groups of patients with a higher potential for poor efficacy or for treatment side effects ( Table 11.2 ). The beneficial effects of utilizing information derived from pharmacogenomics in the future of clinical medicine may lead to improved success rates, decreased side effect burdens, and reduced health care costs.
Gene or Enzyme | Examples of Drugs Metabolized by This Enzyme | Phenotype and Frequency by Groups | Clinical Effect |
---|---|---|---|
CYP1A2 | Anticonvulsants: phenytoin, carbamazepine substrates Antidepressants: clomipramine, fluoxetine, fluvoxamine, imipramine, maprotiline, nortriptyline Muscle relaxant: cyclobenzaprine NSAIDs: acetaminophen, naproxen | PM: Caucasian, 12% | Weak metabolism of enzyme |
CYP2C9 | Warfarin substrates Phenytoin Angiotensin II blockers: irbesartan, losartan NSAIDs: celecoxib, diclofenac, ibuprofen, meloxicam, naproxen Oral antidiabetics: glipizide, tolbutamide | PM: Caucasian, 2%-6% | Weak metabolism of enzyme |
CYP2C19 | Anticonvulsants: diazepam, phenytoin substrates Proton pump inhibitors: omeprazole, pantoprazole | PM: Caucasian, 2%-6%; Chinese,15%-17%; Japanese, 18%-23% | Weak metabolism of enzyme |
CYP2D6 | Analgesics: codeine, dextromethorphan, oxycodone, tramadol substrates Antiarrhythmic drugs: ajmaline, flecainide, mexiletine, propafenone Antiemetics: metoclopramide Antipsychotics + SSRIs: fluoxetine, haloperidol, paroxetine β-blockers: metoprolol, propranolol, timolol 5-HT3 antagonists: ondansetron, tropisetron TCAs: amitriptyline, clomipramine, desipramine, imipramine | PM: Caucasian, 3%-10%; Chinese/Japanese/African American, <2% UR: Ethiopian, 20%; Hispanic, 7%; Scandinavian, 1.5% | Weak metabolism of enzyme Enhanced metabolism of enzyme substrates |
The Impact of Pharmacogenomic Research on Pain Medicine
Individuals have differential responses to pain and different likelihoods for developing chronic pain. In 2006, Tegeder and colleagues reported that GTP cyclohydrolase (GCH1), the rate-limiting enzyme for tetrahydrobiopterin (BH4) synthesis, is a key modulator of peripheral neuropathic and inflammatory pain, tracing the predisposition for high pain tolerance to this enzyme cofactor, which is upregulated in primary sensory neurons of the dorsal root ganglion following nerve injury. This cofactor modulates both inflammatory and neuropathic pain. These investigators identified a “pain protective” polymorphism in 15% of the population within the gene that encodes for this essential cofactor involved in this specific pain pathway, which yields reduced pain sensitivity. Individuals with this “pain protective” genotype could potentially confound analgesic trial results, unless they were identified and randomized. Polymorphisms in other genes have also been linked to pain sensitivity.
Genetic predisposition also affects an individual’s susceptibility to specific complications of common disease states such as diabetes-associated nephropathy. A genome-wide scan identified two novel candidate genetic loci associated with diabetic nephropathy, implicating new pathways in the pathogenesis of this debilitating complication of diabetes. Pharmacogenomic research has the potential to lead to great strides in the optimization of analgesics as well. Genetic variance can impact the therapeutic response of specific pain medications or predispose an individual to adverse effects. Differences in clinical response to pain medications, in terms of efficacy, toxicity, pharmacokinetics, metabolism, and drug transport, have been linked in part to genetic variations. In 2004, Fishbain and colleagues concluded that “genomic testing for enzymes of drug metabolism has significant potential for improving the efficacy of drug treatment and reducing adverse drug reactions,” notably for the pain therapies: tricyclic antidepressants, anti-inflammatory drugs, and opioids, among others.
The Impact of Pharmacogenomic Research on Pain Medicine
Individuals have differential responses to pain and different likelihoods for developing chronic pain. In 2006, Tegeder and colleagues reported that GTP cyclohydrolase (GCH1), the rate-limiting enzyme for tetrahydrobiopterin (BH4) synthesis, is a key modulator of peripheral neuropathic and inflammatory pain, tracing the predisposition for high pain tolerance to this enzyme cofactor, which is upregulated in primary sensory neurons of the dorsal root ganglion following nerve injury. This cofactor modulates both inflammatory and neuropathic pain. These investigators identified a “pain protective” polymorphism in 15% of the population within the gene that encodes for this essential cofactor involved in this specific pain pathway, which yields reduced pain sensitivity. Individuals with this “pain protective” genotype could potentially confound analgesic trial results, unless they were identified and randomized. Polymorphisms in other genes have also been linked to pain sensitivity.
Genetic predisposition also affects an individual’s susceptibility to specific complications of common disease states such as diabetes-associated nephropathy. A genome-wide scan identified two novel candidate genetic loci associated with diabetic nephropathy, implicating new pathways in the pathogenesis of this debilitating complication of diabetes. Pharmacogenomic research has the potential to lead to great strides in the optimization of analgesics as well. Genetic variance can impact the therapeutic response of specific pain medications or predispose an individual to adverse effects. Differences in clinical response to pain medications, in terms of efficacy, toxicity, pharmacokinetics, metabolism, and drug transport, have been linked in part to genetic variations. In 2004, Fishbain and colleagues concluded that “genomic testing for enzymes of drug metabolism has significant potential for improving the efficacy of drug treatment and reducing adverse drug reactions,” notably for the pain therapies: tricyclic antidepressants, anti-inflammatory drugs, and opioids, among others.
Tricyclic Antidepressants
Consider the patient example, in which the TCA administered to the patient presenting with PHN led to intolerable adverse effects. TCAs are metabolized in the liver by the cytochrome P450 (CYP450) system, which is responsible for the breakdown of 40% to 50% of all commonly prescribed medications. CYP450 is the most intensely studied gene family, and polymorphisms within this class of enzymes can lead to reduced or accelerated metabolism of their substrates, including specific medications. As such, two individuals having the same weight and given the same drug dosage can have more than a 1000-fold difference in their plasma drug levels. This can be extremely important to recognize from a clinical viewpoint. In studies, individuals have been grouped by their phenotype: poor metabolizers, who have two nonfunctional enzyme alleles; intermediate metabolizers, who have at least one reduced functional allele of an enzyme; extensive metabolizers, who have at least one functional allele; and ultra-rapid metabolizers, who have multiple copies of a functional allele or an allele with a promoter mutation that confers increased transcription of that gene. Extensive metabolizers obtain the expected therapeutic benefit from standard doses of a drug, whereas individuals who are poor metabolizers are at risk of poor efficacy or adverse effects if given a prodrug that is incompletely metabolized due to genetic differences in their liver enzyme systems. On the other hand, ultra-rapid metabolizers can be prone to adverse effects because of higher than expected drug concentrations from enhanced metabolism. The plasma drug concentrations in individuals given the same dose of antidepressants have been found to vary—primarily based on the polymorphisms present in the CYP2D6 enzyme (as well as CYP1A2, CYP2C19, and CYP3A4/5). Relevant to the patient presented at the beginning of this chapter, individuals who are poor metabolizers of TCAs (approximately 7% of the Caucasian population) tend to accumulate drug concentrations outside of the narrow therapeutic range of the drug classes, leading to adverse TCA effects at lower than expected doses. In contrast, patients who are CYP2D6 ultra-rapid metabolizers may require higher doses to achieve analgesia. Potentially, the patient presented is a CYP2D6 poor metabolizer.
The value of genotyping before prescribing antidepressants was considered in a large population-based study of 1198 elderly Dutch patients. Perhaps not surprisingly, poor metabolizers of TCA’s more than extensive metabolizers required either lower maintenance doses or discontinuation of TCAs altogether. Although research does not provide clear evidence to support the routine clinical use of liver enzyme genotyping before analgesic treatment, an increasing number of commercial entities have developed the capabilities to perform these assays and thus they are now available for the clinician to consider using. Clinically, the recognition that an individual patient could be a poor metabolizer, with or without confirmatory testing, can assist the prescriber to consider medication changes (dose adjustment or frank discontinuation) when poor efficacy or adverse events are observed.
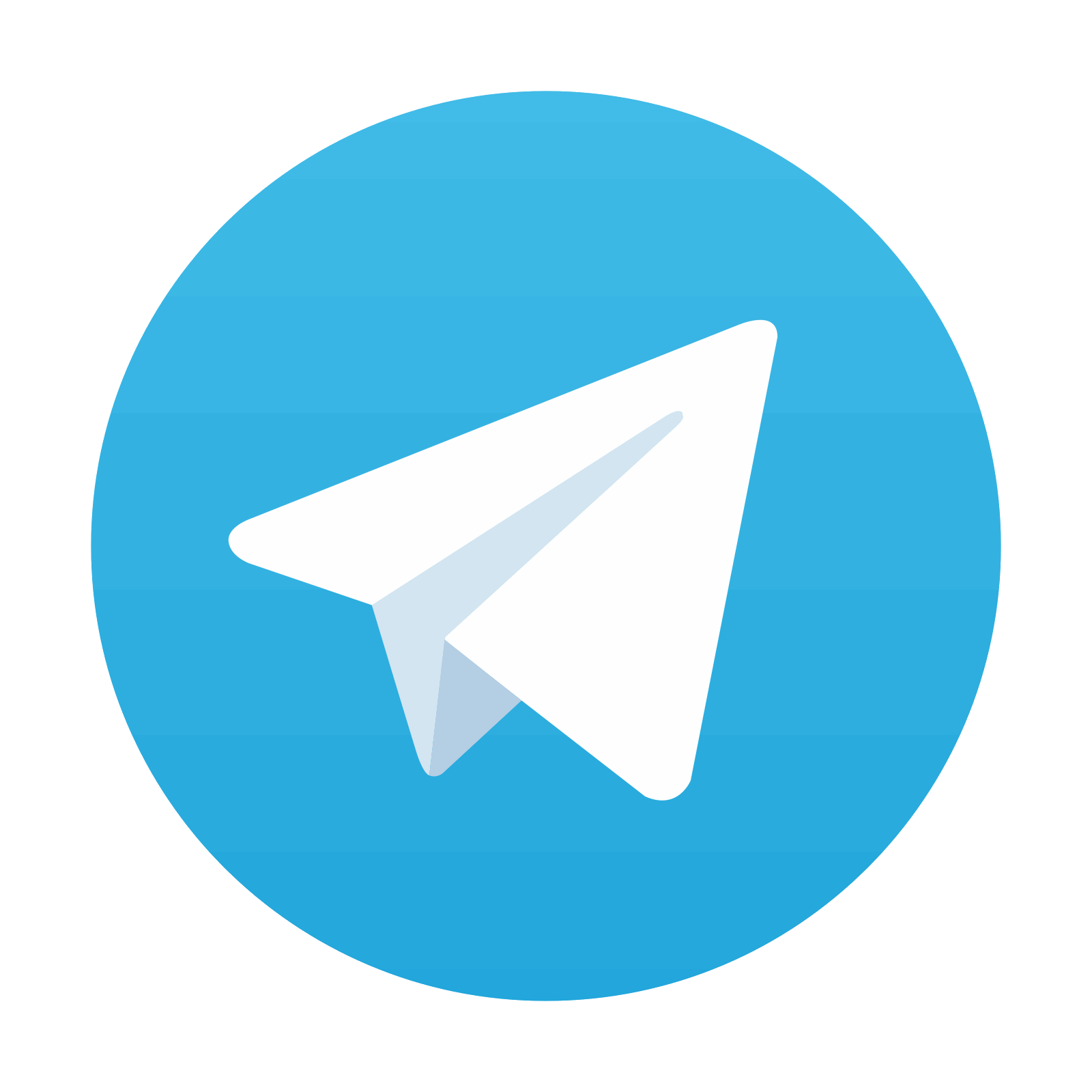
Stay updated, free articles. Join our Telegram channel

Full access? Get Clinical Tree
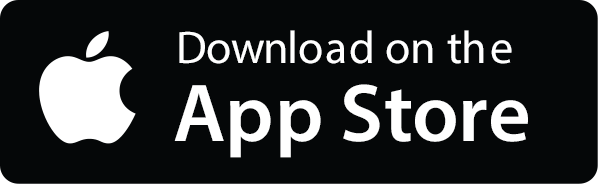
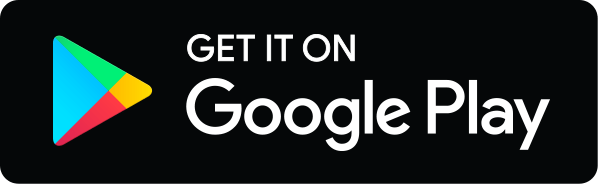