Fig. 12.1
The glycolysis pathway
12.2 Pyruvic Acid
Pyruvic acid (CH3COCOOH) is an organic acid, the simplest of alpha-keto acids (obtained by pyrolysis of tartaric acid). The anionic form of pyruvic acid is pyruvate (CH3 CO CO O−): it represents the intermediate key factor in a lot of metabolic paths, particularly related to energetic metabolism (Fig. 12.2).


Fig. 12.2
The pyruvic acid molecule; black circles represent carbon atoms, red circles represent oxygen atoms, and white circles represent hydrogen atoms
Generated through glycolysis from glucose, pyruvate may:
1.
Be reconverted to carbohydrate through “gluconeogenesis”
2.
Be reconverted to fatty acids through acetyl-CoA
3.
Be used to synthesize the amino-acid alanine
4.
Be fermented to ethanol or lactate
Particularly, as an energy compound:
1.
It can enter the Krebs cycle (thereby entering the oxidative phosphorylation in aerobiosis conditions).
2.
It can undergo alcoholic (particularly in vegetables) or lactic fermentation.
12.3 From Pyruvate to Lactate
As glycolysis comes to an end, one molecule of glucose is split into two molecules of pyruvate (from a 6-carbon molecule to two molecules composed of 3 carbons each).
During this process, a small proportion of the whole available energy contained in one molecule of glucose is released. Indeed, since pyruvates are still relatively reduced, they already contain most of the energy.
Further energy can be released by pyruvate through two main paths.
In aerobic conditions, the pyruvate-dehydrogenase complex, a multienzymatic compound composed of three proteins located in both the mitochondria of eukaryotic cells and the cytosol of prokaryotic cells, oxidizes pyruvic acid to acetyl-CoA and CO2 [2].
The overall resultant reaction (actually three subunits catalyze three different reactions) is represented by an oxidative decarboxylation which also sets out the intervention of several coenzymes and prosthetic groups (namely, coenzyme A, lipoic acid, FAD, NAD).
The resulting acetyl-CoA enters the mitochondrial next phase of aerobic metabolism, which is alternatively named tricarboxylic acid cycle, or Krebs cycle.
As the cycle starts, acetyl-CoA is condensated with oxaloacetate to generate citrate.
From this point on, oxaloacetate will be cyclically regenerated (and here again condensated with acetyl-CoA). Each cycle will produce one molecule of ATP and, most importantly, NADH and FADH.
Those are reduced cofactors whose entrance into the electron transport chain – known as “oxidative phosphorylation” – catalyzes energy production of 36 molecules of ATP.
On the other hand, under conditions of low oxygen availability, pyruvate undertakes the path of lactic fermentation. This will produce L-lactate and oxidized NAD [3].
It is noteworthy that a true anaerobic state didn’t represent the unique circumstance characterized by the activation of lactic fermentation since it also takes place under different conditions like intense muscular activity, where insufficient local content of O2 is present.
12.4 What Is Lactate?
Lactic acid (whose IUPAC classification name is hydroxypropanoic acid) is a chemical compound that plays a significant role in many biochemical processes.
It is a large carboxylic acid characterized by the chemical formula C3H6O3. Its deprotonation (loss of a H+) gives rise to the lactate ion (Fig. 12.3) [4].


Fig. 12.3
The lactic acid molecule; black circles represent carbon atoms, red circles represent oxygen atoms, and white circles represent hydrogen atoms
Actually, the commonly called “lactate ion” is represented by just the L-lactate stereoisomer. D-lactate is in fact only present in patients affected by short bowel syndrome and requires special measures to be determined.
Given a pH of 7.4, the lactate/lactic acid ratio is in the order of 3,548:1.
Lactic acid is therefore a strong acid (at least in a biological context), which strongly dissociates into lactate ions and hydrogens when it is solved in a solution (pKa = 3.9 to 7.4). Lactate is therefore a strong ion as well.
It is noteworthy that, in clinical settings, one commonly speaks about lactate instead of lactic acid.
12.5 What Is Lactate Unit of Measurement? What Are Its Normal Values?
Lactate should be measured in mmol/L (millimoles per liter).
An easy method for converting mg/dl to mmol/L with regard to lactate is to divide the former by 9.01.
When lactate concentration is expressed in mmol/L, one can directly use it for the “anion gap” computation.
The normal plasmatic concentration of lactate in health individuals is traditionally considered to be 1 ± 0.5 mmol/L. In critically ill patients, values up to 2 mmol/L are considered normal.
Since the 1970s, clinicians made a distinction between “hyperlactacidemic states,” conditions characterized by lactate values ranging from 2 to 5 mmol/L, and “lactic acidosis,” more alarming circumstance characterized by higher-than-5 mmol/L values and usually associated with decrements of pH [5].
Both venous and arterial blood samples are accepted for clinical purposes. In almost every ICU worldwide, lactate is measured through complex instrumentations, the same used for the determination of blood gases, hemoglobin (Hb), and electrolytes.
Specifically, lactate concentration is measured within an amperometric cell. Here, after the enzymatic scission of lactate into hydrogen peroxide, an electric current, whose intensity is proportional to the peroxide concentration, is quantified.
The normal arterial and/or venous lactate value strictly represents the balance between lactate production and lactate disposal, for consumption or removal (clearance).
Notably, although this concentration is less than 2 mmol/L, the daily production of lactate is around 1,500 mmol/day (0.8 mmol/kg/h−1).
12.6 Which Tissues Are Responsible for Lactate Production?
In physiological conditions, lactate is produced by:
1.
Muscular cells (25 %)
2.
Skin (25 %)
3.
Brain (20 %)
4.
Erythrocyte (20 %)
5.
Intestinal cells (10 %)
and, actually, by all the cells whose main energetic source is glycolysis, especially in its low efficient form, characterized by the shift of pyruvate to lactic fermentation [4].
We must notice that erythrocytes, that can be considered as a 2,500 g “organ”, are forced to anaerobic metabolism since they don’t have mitochondria: their cellular respiration thereby relies exclusively on their lactate production. Lactate is then converted into fatty acids by the liver.
12.7 Which Tissues Can Use Lactate as an Energy Source?
The energy demand of the heart is high and continuous at the same time. The maintenance of an energetic homeostasis relies on a continuous supply of ADP, inorganic phosphate, oxygen, and reducing equivalents (NADH). Unlike the brain, the heart may utilize different energy substrates depending on different conditions (e.g., rest or exercise). Traditionally, only glucose and long-chain fatty acid were thought to play a pivotal role. Lactate, thus, seems to be crucial as well.
The uptake of lactate by the heart depends, in vivo, on its concentration in serum.
Significant interactions occur between lactate and glucose and between lactate and fatty-acid beta-oxidation, giving lactate the role of a potential energy substrate.
The human brain contains 1,000 billions of cells, 90 % of them being represented by glial cells serving multiple functions (trophic, energetic, and immune functions; regulation of ionic concentration; and nervous impulse conduction support). The remaining 10 % is represented by neurons. The brain has an elevated energy consumption: it accounts for only 2 % of body weight but consumes 50 and 10 % of blood oxygen and glucose, respectively. It exclusively employs glucose as energy substrate. Energy is consumed mainly by neurons, mostly for the Na/K active antiport (50 % of energy) necessary for their activity (i.e., to generate electrical signals). Glucose is therefore stored in the form of glycogen into glial cells (namely, astrocytes). Astrocytes can recognize neurons’ activity identifying the presence of neurotransmitters into the extracellular spaces.
The brain contains an average amount of 115 mg of glycogen per 100 g of tissue; its needs are instead approximately 120 g/day and are all provided by blood circulation.
Glucose is absorbed by the astrocytes, whose “feet” overlay cerebral capillaries surface. The absorbed glucose is turned into astrocytes and converted to lactate. This represents the actual main substrate used by neurons. Astrocytes are sensitive to glutamate, the main neurotransmitter of the human brain. Being an indicator of neuronal activity, it can induce an increased glucose uptake in astrocytes. The more neurons are active, the more glucose is absorbed.
Glutamate is removed from extracellular spaces by entering into astrocytes and being turned into glutamine. It will then be provided to neurons, where it will be transformed back to glutamate. Glutamine is carried into the astrocyte by a symport system together with Na, while the Na/K pump will proceed to the extrusion of the latter. This causes an energy consumption supported by the 2 ATPs obtained through the anaerobic glycolysis. Glucose thereby begins its metabolic pathway in astrocytes (anaerobic glycolysis = 2 ATPs), and it is carried as lactate into neurons and then turned again into pyruvate and consequently into acetyl-CoA, which enters the tricarboxylic acid cycle, thus producing 34 molecules of ATP.
12.8 What’s the Relationship Between Pyruvate and Lactate?
The LDH-mediated reaction that turns pyruvate into lactate, with NADH transformed into NAD+, tends largely towards the lactate side, with a lactate/pyruvate ratio = 10. Thus, when the pyruvate follows the way of lactic fermentation, the conversion of pyruvate into lactate is really favorable.
In any time that pyruvate tends to accumulate in cells, it undergoes this easy and immediate metabolic path.
12.9 What Makes It Easier for Pyruvate to Accumulate?
The pyruvate accumulates every time that its production, especially through glycolysis, exceeds its usage by the mitochondria.
Therefore, a quantitative increase in glycolysis production and an obstacle in entering the oxidative phosphorylation through the Krebs cycle (the aerobic way) are either cause of pyruvate overload: at this point, pyruvate is necessarily converted into lactate through fermentation.
12.10 What Is Lactate Metabolic Destiny?
Although lactate is an electrically charged molecule, it can easily cross the membranes through a transmembrane transport system (monocarboxylate transporter). This couples lactate to H+ during the transfer.
This active transport outward the cell is vital because it avoids an undesired alteration of intracellular pH.
After its production, lactate can:
1.
Be transformed into oxaloacetic acid after reconversion to pyruvate (carboxylation)
2.
Be converted into alanine after reconversion to pyruvate (transamination)
3.
Be used untransformed directly by the periportal hepatocytes, in order to produce glucose (gluconeogenesis) or glycogen (glycogenesis).
The liver manages 60 % of the total lactate and most of the aforementioned metabolic pathways.
Kidneys are the second most important organs involved in lactate metabolism: their cortex can produce glucose through the gluconeogenic pathway.
Kidneys don’t excrete lactate unless their plasmatic levels reach a warning threshold. This is due to its important role as an energy substrate.
When hyperlactatemia occurs, we are unavoidably facing an increased production of lactate or a reduced clearance or both.
In case of a severe systemic hypoperfusion (shock), the regional blood flows are the main determinant of each organ’s relative contribution to lactate production and extraction.
In experimental hemorrhagic models, with moderate hypoperfusion, low splanchnic flow is not associated with low hepatic flow, whose perfusion is essentially preserved. In case of worsening of hemorrhage and hypoperfusion, the liver itself becomes a lactate producer.
12.11 What Is “Cori Cycle”?
Cori cycle is named after the spouses Gerty and Carl Cori, who won the Nobel Prize in 1947 for this discovery.
In an anaerobiotic environment, a muscle in activity would inevitably develop lactic acidosis and local decrease of pH, if a prompt transfer of lactate to the liver through the circulation didn’t take place.
The hepatocytes move lactate to gluconeogenesis (which consist in a reverse fermentation and glycolysis: from lactate to pyruvate and from pyruvate to glucose): the glucose produced is addressed again to the working muscle by the systemic circulation.
The cost of each molecule of lactate transformed into glucose through the Cori cycle is 6 ATP molecules, instead of the 2 molecules normally produced in the glycolytic cycle. It follows that, given its high energy cost, the cycle cannot be indefinitely sustained.
Indeed, this is the reason why a prolonged effort has to be performed through the much cheaper aerobic pathway.
When muscular effort ceases, the regenerated glucose follows the path of glycogen regeneration (glycogenosynthesis) in order to compensate for the amount of glycogen used in the first phases of muscular work.
12.12 NADH and NAD
To fully understand the energetic metabolism and the role played by lactate within it, one needs to understand the role of NAD and its corresponding reduced form NADH.
NAD is an acronym for redox coenzyme “nicotinamide adenine dinucleotide.”
It is a biomolecule whose biological role is to transfer electrons, thus realizing oxidation–reduction reactions, and move hydrogen atoms.
The term “NAD” stands for the oxidized form of the molecule, while “NADH” indicates its reduced form (sometimes referred to as NADH2).
Since it takes part in a redox (reduction–oxidation) reaction, the reduction of the coenzyme (NAD to NADH) promotes the oxidation of a substrate.
It has already been reported that glycolysis of one glucose molecule consumes 2 NAD, thereby generating 2 NADH molecules. On the other hand, lactic fermentation reoxidizes NADH to NAD.
The physiological mechanism of glycolysis towards aerobic energy production and oxidative phosphorylation requires a balance between the levels of NAD and NADH: a correct NADH/NAD ratio ensures a correct pyruvate usage which, as we have seen before, is a paramount turning point for energy metabolism.
The rate of conversion from pyruvate to lactate is in fact controlled by the NADH availability: NADH excess facilitates the lactic fermentation that produces NAD.
Actually, when an excess of NADH (and therefore lack of NAD) occurs, alternative mechanisms (shuttles) are activated in order to force the conversion of NADH to NAD at a mitochondrial level (namely, malate–aspartate shuttle and glycerol-3-phosphate shuttle).
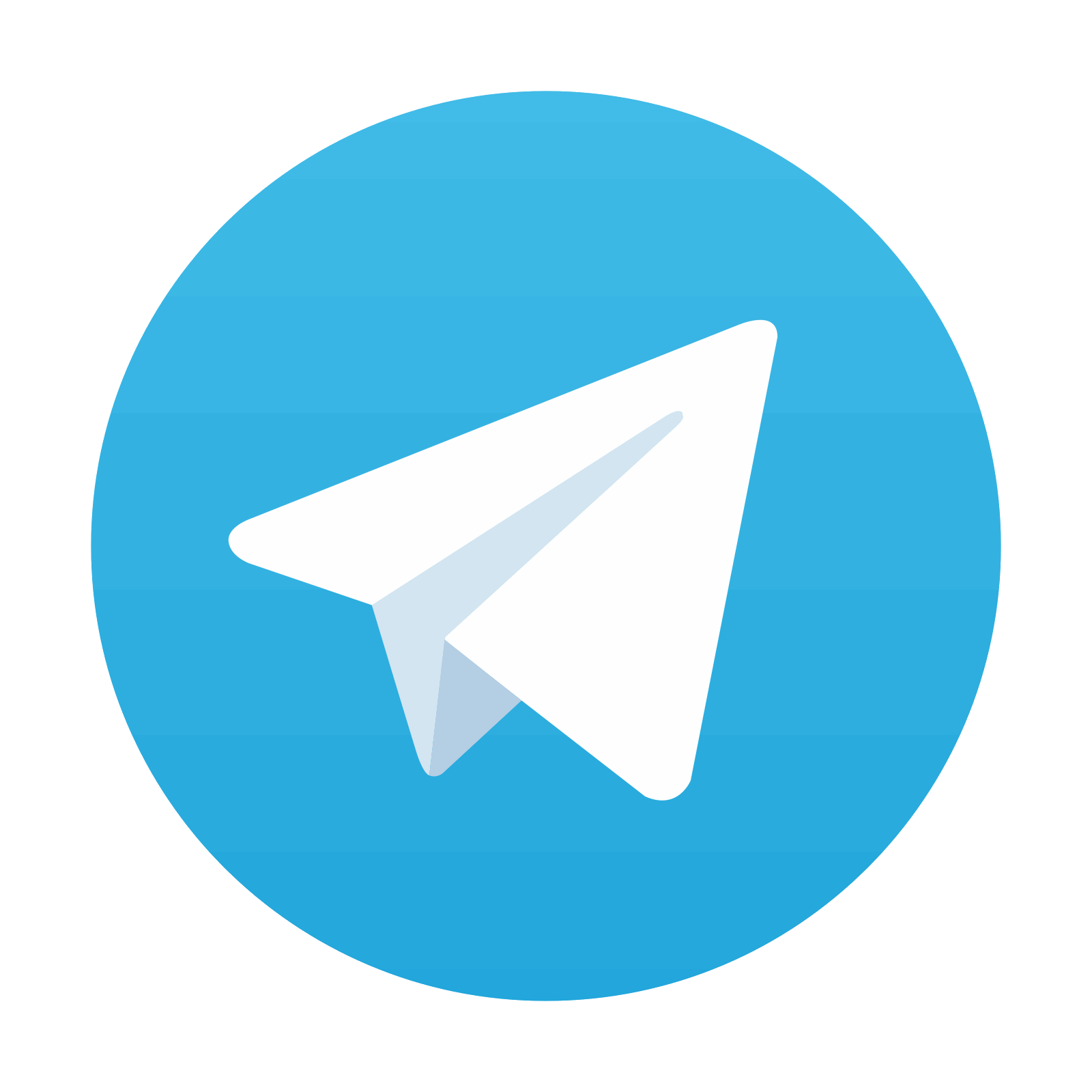
Stay updated, free articles. Join our Telegram channel

Full access? Get Clinical Tree
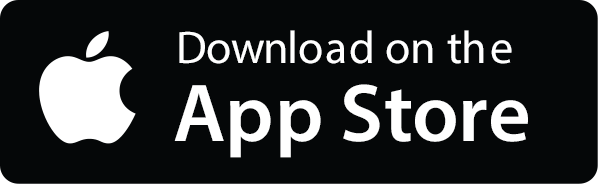
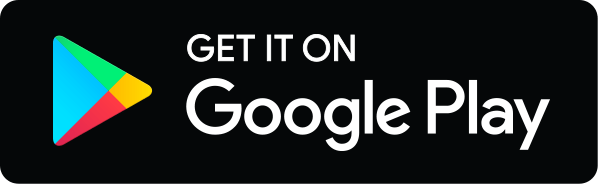