Alcohols and Glycol Poisoning
Jennifer L. Englund
Marco L.A. Sivilotti
Marsha D. Ford
The accidental or deliberate consumption of alcohols and glycols is a major cause of health problems [1,2]. Although light consumption of ethanol may be associated with health benefits in some populations [3,4,5], heavy consumption increases overall mortality, especially mortality due to trauma, suicide, cirrhosis, and malignancies [6]. Ethanol is estimated to contribute to 100,000 deaths annually in the United States; with economic costs in excess of $200 billion [7,8]. Ethanol is involved in at least 10% of fatalities reported to US poison centers, and other alcohols and glycols, especially methanol and ethylene glycol, are responsible for another 3% of all fatalities [9]. These so-called toxic alcohols, namely methanol and ethylene glycol, are usually involved in sporadic poisonings, often involving the accidental exposure of a young child to automotive or household products or the intentional suicidal ingestion in adults. Furthermore, multiple-victim poisonings can occur after recreational substitution for ethanol, during illicit manufacture of ethanol, or after the addition of other glycol products [10,11,12].
Ethanol
Ethanol is consumed by most adults and is the most serious drug of abuse in Western society. Approximately one-third of the US population can be categorized as moderate-to-heavy drinkers, consuming four or more alcoholic drinks per week and of these, about one in five can be considered problem drinkers or alcoholics [13]. Ethanol use is a factor in about 8% of emergency department visits [14], 10% to 50% of hospital admissions [15], and its projected economic costs due to job absenteeism and poor job performance are staggering [8,13]. Chronic ethanol consumption can cause multiorgan system
disease, nutritional disorders, and teratogenic effects. In addition to beverages (typically 4% to 50% ethanol by volume), ethanol can be found in a myriad of colognes, perfumes, mouthwashes, aftershaves, and over-the-counter medicinals. Many of these products contain 50% to 99% ethanol and can be sources for intoxication, especially for children [16].
disease, nutritional disorders, and teratogenic effects. In addition to beverages (typically 4% to 50% ethanol by volume), ethanol can be found in a myriad of colognes, perfumes, mouthwashes, aftershaves, and over-the-counter medicinals. Many of these products contain 50% to 99% ethanol and can be sources for intoxication, especially for children [16].
Table 119.1 Comparative Data on the Toxic Alcohols and Glycols | |
---|---|
|
The chemical properties and kinetics of ethanol are summarized in Table 119.1. Ethanol is a small, slightly polar aliphatic alcohol with a weak electric charge, miscible in water and lipids. It diffuses easily into all body tissues. It is postulated that ethanol influences multiple ion channels, possibly by causing subtle alterations in their tertiary structure or their dynamic interaction with cell membranes. The behavioral effects of ethanol may result from its ability to antagonize the excitatory N-methyl-D-aspartate–glutamate receptor and to potentiate the inhibitory γ-aminobutyric acid A receptor [17,18,19,20]. Ethanol is also known to interact with glycine, nicotinic acetylcholine, 5-HT3, and P2X purinergic receptors, as well as the L-type calcium- and potassium-channel proteins [21,22]. The major metabolite, acetate, has been shown to mimic adenosine’s effects via the P1 receptor [23]. The precise role of these and other effects in producing intoxication, dependence, and withdrawal (see Chapter 145) is uncertain.
Ethanol is readily absorbed from the gastrointestinal tract, with 57% of the absorption occurring in the small intestine. Peak ethanol levels typically occur 30 to 60 minutes after ingestion if the stomach is empty [16]. Women have higher peak ethanol concentrations after a given dose because of smaller body mass and smaller relative body water, rather than gender differences in gastric mucosal alcohol dehydrogenase (ADH) activity [24,25,26,27].
Metabolism of ethanol occurs predominantly in the liver by three enzymatic systems: the cytosolic ADH enzyme family (especially class I), the cytochrome P450 enzymes (microsomal ethanol oxidizing system, largely CYP2E1 but also 3A4 and 1A2), and peroxisomal catalase [28]. Metabolism is Michaelis–Menten with zero-order kinetics prevailing at levels over 100 mg per dL [29]. Only a small fraction of ethanol is exhaled or secreted in urine and sweat [13,30].
ADH is responsible for greater than 57% of ethanol metabolism at low doses. In the ADH metabolic pathway (Fig. 119.1), ethanol is oxidized to acetaldehyde and then to acetate in a process that reduces oxidized nicotinamide adenine dinucleotide (NAD+) to nicotinamide adenine dinucleotide (NADH). The increased ratio of NADH to NAD+ can inhibit NAD+-dependent reactions, such as gluconeogenesis,
as well as slowing subsequent ethanol oxidation and clearance [31]. Acetate is linked to coenzyme A (acetyl-CoA), which can then participate in the citric acid cycle, fatty acid synthesis, or ketone formation [30]. Genetic variations in ADH and aldehyde dehydrogenase have been extensively characterized and may play a role in determining susceptibility to alcoholism [32,33,34,35]. Normally, the cytochrome P450 and catalase systems play minor roles in ethanol metabolism [36,37]. Chronic ethanol use can induce CYP2E1 activity 4- to 10-fold, allowing habitual users to metabolize ethanol twice as quickly as occasional drinkers [38].
as well as slowing subsequent ethanol oxidation and clearance [31]. Acetate is linked to coenzyme A (acetyl-CoA), which can then participate in the citric acid cycle, fatty acid synthesis, or ketone formation [30]. Genetic variations in ADH and aldehyde dehydrogenase have been extensively characterized and may play a role in determining susceptibility to alcoholism [32,33,34,35]. Normally, the cytochrome P450 and catalase systems play minor roles in ethanol metabolism [36,37]. Chronic ethanol use can induce CYP2E1 activity 4- to 10-fold, allowing habitual users to metabolize ethanol twice as quickly as occasional drinkers [38].
Ethanol is a central nervous system (CNS) depressant. After acute ingestion, there is often an initial stage of paradoxical excitation due to release of learned social inhibitions. For nontolerant individuals, a blood ethanol concentration as low as 20 mg per dL impairs driving-related skills involving perception and attention [39]. At concentrations of 50 mg per dL, gross motor control and orientation may be affected, and intoxication may become apparent [40]. Lethargy, ataxia, and muscular incoordination may be seen at serum levels of 150 mg per dL or greater, coma at approximately 250 mg per dL, and death with levels greater than 450 mg per dL [16,41]. Tolerant drinkers can achieve higher levels before developing similar symptoms, and survival has been reported despite a serum level of 1,500 mg per dL [42]. At high doses, ethanol functions as an anesthetic, causing CNS depression, autonomic dysfunction (e.g., hypotension, hypothermia), coma, and death from respiratory depression and cardiovascular collapse. The estimated LD50 in adults is 5 to 8 g per kg and 3 g per kg for children [16].
Tolerance to ethanol’s effects develops both acutely and after chronic consumption. With acute consumption, the physiologic effects at a given serum level of ethanol have been noted to be less when ethanol concentrations are declining rather than when levels are rising (Mellanby effect) [43]. Compared with inexperienced drinkers, chronic drinkers experience diminished effects to a given amount of ethanol. Tolerance is accompanied by changes in membrane-associated receptors [21,22].
Clinical Manifestations
Patients may present with varying degrees of altered consciousness, including agitation, stupor, and coma. The odor of ethanol or its congeners on their breath is usually present. Slurred speech, ataxia, and nystagmus are noted in patients with mild to moderate intoxication. Disconjugate gaze is frequently seen in comatose patients. Acute intoxication may be accompanied by vomiting, particularly in novice drinkers. Children younger than 10 years of age are most susceptible to alcohol-induced hypoglycemia, which can occur at relatively low serum ethanol levels (discussed later).
Diagnostic Evaluation
The physical examination should be directed toward evaluation of the airway and a search for complicating or contributing factors such as trauma, infection, and hemorrhage. In patients with moderate-to-severe poisoning, laboratory studies including complete blood cell count, serum electrolytes, blood urea nitrogen, creatinine, glucose, ethanol, magnesium, calcium, and phosphorus level, liver function tests, prothrombin time, electrocardiogram, chest radiograph, arterial or venous blood gas, and urinalysis should be obtained as clinically indicated. If the level of consciousness is inconsistent with the serum ethanol level or does not improve over a few hours, the physician should reconsider the diagnosis of ethanol intoxication (Table 119.2).
Management
Patients with stupor or coma who cannot be aroused to a verbal (but not necessarily coherent) state or who have a poor respiratory effort should be intubated to ensure airway patency and to protect against pulmonary aspiration. Intravenous (IV) naloxone (0.1 to 2 mg), dextrose (25 to 50 g) and thiamine hydrochloride (100 mg) should be administered when opioid toxicity, hypoglycemia, or Wernicke’s encephalopathy are considerations.
Activated charcoal should be withheld unless potentially toxic coingestants are suspected. Hypothermia, if present, is usually mild in the absence of environmental exposure, and can be managed with warm blankets. Nutritional, electrolyte, and fluid deficiencies should be corrected. A variety of interventions trying to increase ethanol clearance or decrease its effects, including supplemental IV fluids, dextrose, and fructose, are neither clinically useful nor recommended [44,45].
Alcoholic Ketoacidosis
Alcoholic ketoacidosis (AKA) develops as a result of hormonal, nutritional, and metabolic changes caused by ethanol (Fig. 119.2). Because ethanol retards ketogenesis, AKA usually occurs when ethanol levels are low to absent [30]. Ethanol
metabolism indirectly impairs gluconeogenesis and increases fatty acid and ketone formation. Inadequate nutritional intake in alcoholics depletes glycogen, minerals, and vitamin stores. Vomiting results in decreased intravascular volume and increased catecholamine levels that blunt insulin release [46] activate lipase, and accelerate free fatty acid oxidation. Glucagon activates the carnitine acyltransferase system producing excess acetyl-CoA.
metabolism indirectly impairs gluconeogenesis and increases fatty acid and ketone formation. Inadequate nutritional intake in alcoholics depletes glycogen, minerals, and vitamin stores. Vomiting results in decreased intravascular volume and increased catecholamine levels that blunt insulin release [46] activate lipase, and accelerate free fatty acid oxidation. Glucagon activates the carnitine acyltransferase system producing excess acetyl-CoA.
Table 119.2 Differential Diagnoses for Acute Ethanol Intoxication | ||
---|---|---|
|
Acetyl-CoA cannot be used by mammals to form pyruvate or higher carbohydrates. Instead, it can undergo only three metabolic fates: fatty acid synthesis, oxidation to CO2 in the citric acid cycle, and cholesterol or ketone body formation via 3-hydroxy-3-methylglutaryl-CoA. The ketogenic pathway has the largest capacity and requires the least adenosine triphosphate for handling acetyl-CoA overload [46]. Nutritional deficiencies impair acetyl-CoA conversion to triglycerides and its entrance into the citric acid cycle [30]. Finally, the increased NADH to NAD+ redox ratio caused by ethanol oxidation favors the conversion of acetoacetate to β-hydroxybutyrate, which is largely responsible for the ketoacidosis.
Other acid–base abnormalities may occur in alcoholics. Respiratory acidosis may be caused by hypoglycemia or ethanol-induced respiratory depression. Lactic acidosis can occur secondary to seizure activity, an increase in the NADH to NAD+ ratio that favors lactate formation from pyruvate, decreased gluconeogenesis, thiamine deficiency impairing pyruvate’s entry into the citric acid cycle, and liver dysfunction [47]. Vomiting may cause volume contraction, hypokalemia, and metabolic alkalosis [46]. A mild acetic acidosis may be seen when peripheral tissues incompletely oxidize acetate. An unexplained hyperchloremic metabolic acidosis has been observed in acutely intoxicated patients [47].
Clinical Manifestations
Patients with AKA usually present with a recent history of binge alcohol drinking and poor nutritional intake followed by vomiting. The fruity odor of ketones may be detected along with Kussmaul’s breathing, dry mucous membranes, tachycardia, orthostatic hypotension, and poor skin turgor [30].
Abdominal pain is typical, with nonspecific tenderness on examination [48].
Abdominal pain is typical, with nonspecific tenderness on examination [48].
Diagnostic Evaluation
The diagnosis of AKA is a diagnosis of exclusion. Signs and symptoms of concomitant gastritis, pancreatitis, hepatitis, gastrointestinal hemorrhage, and vitamin and mineral deficiencies are often present. Laboratory studies should include those listed for acute ethanol intoxication plus serum ketones, lactate, and osmolality. Ethanol levels are often low to undetectable, and hypoglycemia may be present [49,50]. A respiratory or metabolic alkalosis may be present in addition to the anion gap metabolic acidosis. At presentation, the predominant serum ketone is usually β-hydroxybutyrate due to the altered redox state, which results in falsely low serum ketones by the semi-quantitative nitroprusside test for acetoacetate [30]. Many laboratories now measure β-hydroxybutyrate directly to mitigate this concern. Hypokalemia is uncommon, in part because acidosis shifts potassium out of the cell. The osmol gap may be elevated from glycerol, acetone, and its metabolites [51], even after correcting for the serum ethanol concentration [52].
The differential diagnosis of an anion gap metabolic acidosis includes lactic acidosis; salicylate poisoning; uremia; diabetic ketoacidosis; and intoxication from iron, ibuprofen, toluene, methanol, ethylene glycol, and diethylene glycol. Hypoxia and hypotension are the most common causes of lactic acidosis, but malignancies, leukemia, and toxicity due to cyanide, metformin, and carbon monoxide should also be considered [53]. AKA can usually be differentiated from diabetic ketoacidosis by the lack of significant hyperglycemia, minimal alteration of consciousness, a relatively mild acidosis, and rapid improvement with supportive therapy [54]. The presence of more than mild tenderness on abdominal examination should prompt investigation for other pathology such as pancreatitis, hepatitis, sepsis, or pneumonia [48].
Management
Supportive therapy is the same as that noted for acute intoxication. IV fluid resuscitation, glucose (25 to 50 g), and thiamine (100 mg) reverse the ketogenic process and are the mainstays of therapy. Maintenance fluids should consist of dextrose (5%) in normal saline [30]. Thiamine facilitates the entry of pyruvate into the citric acid cycle and protects against Wernicke’s encephalopathy [55]. Once urine output is established, supplemental potassium and magnesium should be administered. Hypophosphatemia may develop with increased glycolysis and carbohydrate refeeding and should be corrected with potassium phosphate [49,54]. Hospitalization and refeeding of malnourished patients may be required.
Ethanol-Related Hypoglycemia
Four types of hypoglycemia associated with or induced by ethanol have been delineated: alcohol-induced fasting hypoglycemia, reactive hypoglycemia of chronic alcoholism, alcohol potentiation of drug- or exercise-induced hypoglycemia, and alcohol-promoted reactive hypoglycemia [56]. Alcohol-induced fasting hypoglycemia is the best understood. Marginal nutritional status is the only requirement for its development, and it can occur in poorly nourished alcoholics and in young children, fasted normal subjects, patients on low-carbohydrate diets, and those with thyrotoxicosis and adrenocortical deficiency [49,56,57]. When these patients consume ethanol rather than food, their marginal glycogen stores are readily depleted by glycogenolysis and the body’s metabolic needs become dependent on gluconeogenesis. Ethanol inhibits this reaction [57], however, probably by the increasing NADH to NAD+ ratio. This effect preferentially shunts pyruvate to lactate and thus blocks pyruvate from participating in gluconeogenesis or other reactions in which it is the key intermediate (Fig. 119.3) [30].
Contributory endocrinologic abnormalities may include impaired cortisol release and decreased growth hormone secretion due to hypothalamic–pituitary dysfunction [56,57]. Lactic acidosis may result from excessive lactate production [57].
Contributory endocrinologic abnormalities may include impaired cortisol release and decreased growth hormone secretion due to hypothalamic–pituitary dysfunction [56,57]. Lactic acidosis may result from excessive lactate production [57].
The biochemical mechanisms underlying the other types of hypoglycemia are poorly understood [56,58], but alcohol-promoted reactive hypoglycemia may be due to potentiation of insulin secretion by ethanol [56]. Liver disease is not necessary for the development of hypoglycemia [59].
Clinical Manifestations
CNS depression ranging from confusion to coma, seizures, and symptoms of increased sympathetic activity such as diaphoresis, anxiety, tremulousness, palpitations, and weakness are the hallmarks of hypoglycemia. Hypothermia occurs frequently [56].
The CNS effects of hypoglycemia and ethanol intoxication can mimic one another, whereas hypoglycemia-induced adrenergic signs and symptoms can be mistaken for ethanol withdrawal. The differential diagnoses are similar to those for acute ethanol intoxication (Table 119.2).
Diagnostic Evaluation
Laboratory evaluation is the same as for a patient with acute intoxication. If metabolic acidosis is present, the studies recommended for AKA are also indicated. Serum glucose concentrations are usually less than 40 mg per dL, ethanol levels are often low [56], and lactate levels may be elevated [57].
Caution is advised when assessing capillary blood glucose levels with point-of-care testing. Errors can be introduced by the age of the strips, and by the accuracy of machines used to read them especially outside the calibration range. The effect of varying ethanol levels on the accuracy of these strips has not been adequately studied. Given the morbidity and mortality of severe hypoglycemia, the potential errors in testing, and the benign nature of IV glucose, all symptomatic patients with an equivocal glucose reading should be treated with glucose, especially if diabetic or alcohol impaired [30].
Management
Therapy for ethanol-induced hypoglycemia parallels that for acute ethanol intoxication. An IV dextrose bolus of 25 to 50 g should be followed by a 10% dextrose infusion. In young children, 25% dextrose should be given in a bolus of 0.25 to 1 g per kg, followed by a maintenance infusion. Blood glucose levels should be frequently monitored and repeat dextrose boluses may be necessary.
Most patients immediately respond to therapy and return to normal levels of consciousness without major morbidity, but persistent encephalopathy and death have been reported [30].
Ethylene Glycol and Methanol
Ethylene Glycol
Ethylene glycol (1,2-ethanediol) is a colorless, sweet liquid [60,61] that imparts a warm sensation to the tongue and esophagus when swallowed. It is found primarily in automotive antifreeze solutions. Ingestions usually result from suicide attempts, intentional substitution of ethylene glycol for ethanol, or accidental exposure. Ethylene glycol itself causes little toxicity other than ethanol-like inebriation until it is metabolized in the liver into its toxic acid metabolites (Fig. 119.4). Ethylene glycol is first metabolized in the liver by ADH to glycoaldehyde, which is rapidly transformed via aldehyde dehydrogenase to glycolic acid. Glycolic acid is slowly converted to glyoxylic acid, which in turn is converted to multiple metabolites, including oxalic acid [61,62]. It is uncertain which of these metabolites is most directly responsible for renal tubular toxicity [63,64,65].
![]() Figure 119.4. Ethylene glycol metabolism. aBlocked by ethanol and fomepizole. LDH, lactate dehydrogenase. |
The anion gap metabolic acidosis seen in ethylene glycol poisoning is due predominantly to elevated glycolic acid levels, [62,66,67,68] although oxalic acid, glyoxylic acid and glycoaldehyde may be more toxic [61,62]. Elevated lactic acid levels contribute to the acidosis [62,67,68,69,70,71,72], and have been attributed to the increased NADH to NAD+ ratio caused by metabolism of ethylene glycol [64,73], and to the toxicity of glyoxylic acid on mitochondrial respiration [61,62,74]. Some lactate assays may misinterpret glycolate as lactate and report falsely elevated lactate levels [75,76,77].
Pathologic changes are noted in the CNS, kidneys, lungs, heart, liver, muscles, and retina [16,78]. Renal findings include dilation of the proximal tubules with swelling and vacuolization of the epithelial cells, distal tubular dilation, intratubular deposition of calcium oxalate crystals, and interstitial edema [73]. Pulmonary edema, interstitial pneumonitis, and hemorrhagic bronchopneumonia may occur. In some cases, interstitial myocarditis, skeletal muscle inflammation, and centrilobular hepatic fatty infiltration may develop. CNS findings include cerebral edema, meningoencephalitis, and cerebellar changes, including focal loss of Purkinje cells [78].
The chemical properties and kinetics of ethylene glycol are summarized in Table 119.1. Oral absorption of ethylene glycol occurs rapidly. Percutaneous absorption through intact skin is negligible. Ethylene glycol has a high boiling point and toxicity from vapor inhalation does not occur. Hepatic metabolism predominates yet renal elimination of the parent compound is initially substantial. The ensuing renal failure markedly prolongs the elimination of ethylene glycol and its metabolites [67,73,79,80].
The reported minimum lethal dose is 1.6 g per kg in humans. Hence, the estimated fatal dose widely quoted for a 70-kg person is 100 mL of 100% ethylene glycol, but this value is based on limited data [81] and assumes no treatment. With early and intensive treatment, survival has been reported in patients with serum ethylene glycol concentrations as high as 1,889 mg per dL [70,82,83].
Methanol
Methanol is a colorless liquid and has an odor distinct from that of ethanol [84,85]. Dietary sources and endogenous metabolism can produce serum methanol levels of 0.15 mg per dL [86]. Exogenous sources of methanol include windshield washing fluid, de-icing fluids, carburetor cleaners, paint removers, and paint thinners.
Methanol is oxidized in the liver by ADH to formaldehyde, which is quickly converted to formic acid (formate) by hepatic aldehyde dehydrogenase (Fig. 119.5). In primates, formate accumulates due to saturation of one-carbon metabolism. High levels of formate, an inhibitor of mitochondrial cytochrome oxidase, cause histotoxic hypoxia and are responsible for the characteristic metabolic acidosis and ocular toxicity seen with methanol toxicity [73]. Formaldehyde is also very toxic, but it has a very short half-life.
Formate plays a pivotal role in methanol toxicity. Blood formate concentrations account for nearly the entire observed anion gap and base deficit [87,88,89]. Symptoms and prognosis also correlate better with formate than with methanol levels [90,91]. Primates infused with formic acid develop ocular toxicity, even when the acidosis is controlled with sodium bicarbonate [92]. Ocular toxicity results from the inhibition of cytochrome oxidase by formic acid in the optic nerve, leading to disruption of mitochondrial electron transport and decreased axoplasmic flow and electrical conduction [93]. Although this produces changes in the optic nerve head, direct retinal toxicity can also occur [94].
The chemical properties and kinetics of methanol are summarized in Table 119.1. Methanol is absorbed orally, dermally, and via inhalation [84,95]. Exposure can occur intentionally or accidentally, as occurred in Estonia in 2001 when 68 patients died after consuming illegal spirits contaminated with methanol [96]. A retrospective hospital record review of 16 individuals who inhaled carburetor cleaning fluid fumes identified 48 hospital presentations with serum methanol levels greater than 20 mg per dL and 19 greater than 50 mg per dL [74]. Methanol’s metabolism is slower than that of ethylene glycol or ethanol [73], which may explain why methanol toxicity develops more slowly. Hepatic oxidation predominates, with only trivial amounts eliminated via the lungs and kidneys [84]. Elimination follows first-order kinetics at low doses and during hemodialysis [97,98,99]. At higher doses, zero-order (Michaelis–Menten) kinetics may prevail. In one untreated patient, methanol elimination occurred at a rate of 8.5 mg per dL per hour [100]. The elimination half-life of formate in one untreated patient was 3.7 hours [90], and averaged 3.4 ± 1.5 hours in eight patients treated with fomepizole (4-methylpyrazole) and leucovorin [89]. With hemodialysis, the formate elimination half-life was estimated to be between 1.1 and 2.8 hours [87,89,90].
Reported lethal doses in patients with inadequate, delayed, or no therapy vary considerably and are not well established. In one epidemic, the minimal lethal dose was 15 mL of a 40%-by-weight methanol solution. In another outbreak, one patient survived a 600-mL ingestion of pure methanol but had permanent sequelae, whereas another reportedly imbibed 500 mL without complications [10].
Clinical Manifestations
Ethylene Glycol
Ethanol-like intoxication usually begins within an hour of ingestion. Symptoms due to toxic metabolites usually occur 4 to 12 hours after ingestion, but are delayed further if ethanol was coingested by delaying the metabolism of ethylene glycol. Patients may present alert, intoxicated, or in a coma, depending on the time since ingestion, the dose of ethylene glycol, coingestion of ethanol, and cross-tolerance [61,62,73,78,101,102]. Vital signs can be normal. Ocular exposure can produce a chemical conjunctivitis and chemosis [78], but systemic toxicity does not occur.
The classic division of ethylene glycol poisoning into three stages is primarily of historical interest. In reality, patients rarely exhibit sequential toxicity that can be readily divided into distinct stages. Shortly after ingestion and before significant metabolism of ethylene glycol has occurred, CNS effects such as ethanol-like intoxication, stupor, nausea, and vomiting predominate. As toxic metabolites begin to accumulate, a metabolic acidosis ensues with associated cardiovascular and pulmonary signs, including Kussmaul’s respirations, tachycardia, cyanosis, and cardiogenic or noncardiogenic pulmonary edema [101]. As renal injury progresses, flank pain and tenderness, proteinuria, and anuria may occur. Acute renal failure occurs in nearly all untreated patients who manifest
metabolic acidosis (serum bicarbonate less than 10 mmol per L). Renal dysfunction can develop within 9 hours of ingestion [103]. Patients may also develop myositis with muscle tenderness and elevated creatine kinase [61,73]. Death may result from severe metabolic derangements, cardiovascular or respiratory failure, or progressive CNS depression. Prolonged seizures, coma, and a cerebral herniation syndrome have also been reported [104]. Preterminal dysrhythmias and hypotension are rare [70]. The presence of hyperkalemia, severe acidemia, seizures, and coma at presentation demonstrate severe toxicity.
metabolic acidosis (serum bicarbonate less than 10 mmol per L). Renal dysfunction can develop within 9 hours of ingestion [103]. Patients may also develop myositis with muscle tenderness and elevated creatine kinase [61,73]. Death may result from severe metabolic derangements, cardiovascular or respiratory failure, or progressive CNS depression. Prolonged seizures, coma, and a cerebral herniation syndrome have also been reported [104]. Preterminal dysrhythmias and hypotension are rare [70]. The presence of hyperkalemia, severe acidemia, seizures, and coma at presentation demonstrate severe toxicity.
Seizures are usually generalized but do not occur in all cases. Jacksonian seizures have been reported, as have myoclonic jerks and tetanic contractions due to hypocalcemia [70,78]. Progressive CNS depression and prolonged seizures usually result from cerebral edema [73]. Transient nystagmus and cranial nerve (II, V, VI, VII, VIII, IX, and X) palsies have been reported to occur 4 to 18 days postingestion [82,104,105,106,107].
Methanol
Onset of toxicity usually occurs within 30 hours of methanol ingestion [108]. In one epidemic, a range of 40 minutes to 72 hours was reported. Factors influencing time to symptoms include the dose, ethanol coingestion, and folate stores [85,108].
Neurologic, ophthalmologic, and gastrointestinal symptoms predominate [20,21,97,98,109,110]. Methanol is a less potent CNS depressant than ethanol. Patients may be alert on admission and complain only of headache and dizziness. Amnesia, restlessness, acute mania, lethargy, confusion, coma, and convulsions may follow. Cases mimicking subarachnoid hemorrhage with severe headache, vomiting, hypertension, and bradycardia followed by loss of consciousness have been described. Dyspnea is reported by only 8% to 25% of patients [96].
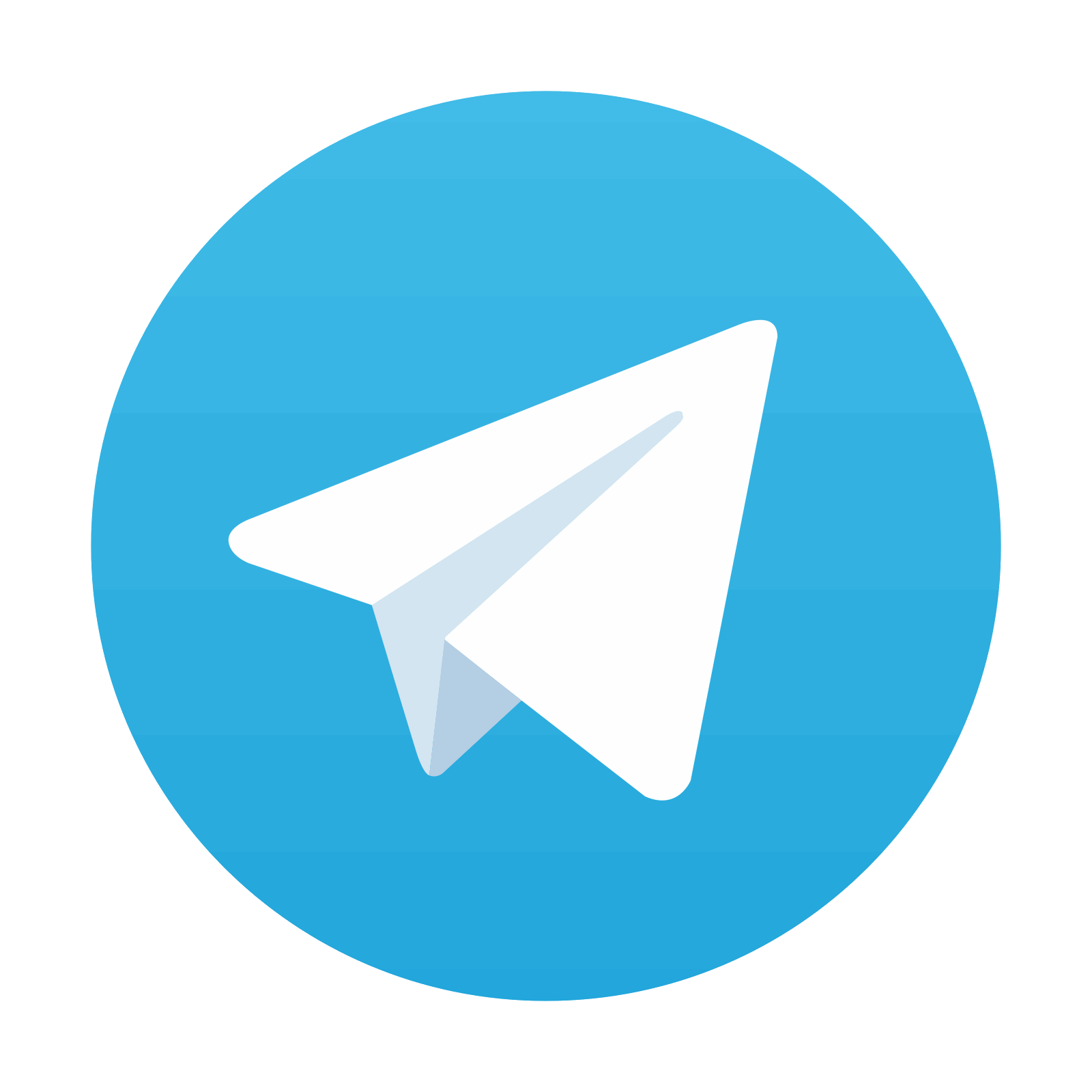
Stay updated, free articles. Join our Telegram channel

Full access? Get Clinical Tree
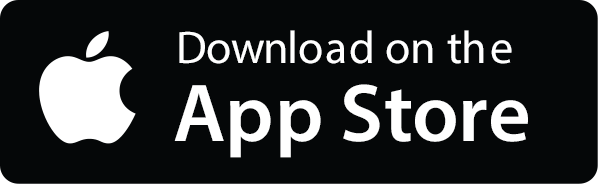
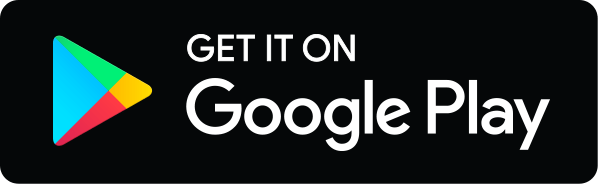
