Anatomy of upper airway (hyoid, thyroid cartilage, cricoid cartilage, trachea, thyroid). (Reproduced from Eur Arch Otorhinolaryngol (2011) 268:1357-1363-p. 1360)
Sonoanatomy

Sonoanatomy of upper airway

Tongue and floor of mouth – transverse scanning plane. (Courtesy of Srikar Adhikari, MD)

Tongue and floor of mouth – sagittal scanning plane. (Courtesy of Srikar Adhikari, MD)

B-mode image of tongue: (a) transverse view and (b) sagittal view using the curvilinear transducer. The mandible (M) contains the tongue bilaterally. The mentum is demarcated by blue, the hyoid bone in red, while the lower border of the muscles in the mouth floor is in dashed yellow. GH geniohyoid muscle,. HG hyoglossus muscles

Hyoid bone in transverse axis seen as an echogenic inverted U-shaped curvilinear structure with posterior acoustic shadowing. (Courtesy of Srikar Adhikari, MD)

Hyoid bone in long-axis narrow hyperechoic curved structure with an acoustic shadow. (Courtesy of Srikar Adhikari, MD)

B-mode image of epiglottis in transverse view seen as hypoechoic curvilinear structure (A-M interface-air-mucosa interface). (Courtesy of Srikar Adhikari, MD)

Thyroid cartilage in transverse plane-hypoechoic inverted V-shaped structure. (Courtesy of Srikar Adhikari, MD)

B-mode image of true vocal cords seen as hypoechoic structures outlined medially by echogenic vocal ligaments. (Courtesy of Srikar Adhikari, MD)

Hyperechoic false vocal cords (FC). (Courtesy of Srikar Adhikari, MD)

Cricothyroid membrane (CM) seen as a hyperechoic band between the hypoechoic thyroid cartilage (TC) and cricoid cartilage (CC) in sagittal plane (A-M interface-air-mucosa interface). (Courtesy of Srikar Adhikari, MD)

Hypoechoic cricoid cartilage in short axis with a bright air-mucosa interface (A-M interface) and reverberation artifacts. (Courtesy of Srikar Adhikari, MD)

Hypoechoic tracheal rings – inverted U appearance in transverse plane with hyperechoic air-mucosa interface and reverberation artifacts posteriorly. The isthmus of the thyroid gland is seen in this image. (Courtesy of Srikar Adhikari, MD)

Trachea, cricothyroid cartilage, and cricothyroid membrane scanning using a linear transducer (a) upper mid-sagittal plane, (b) lower mid-sagittal plane, and (c) transverse plane. The white hyperechoic line represents the tissue/air interface, while all echoes below it represent artifacts. The thyroid and cricoid cartilages are lined by yellow and red, respectively, and the arrow between them represents the cricothyroid membrane. T1, T2, and T3 indicate the first three tracheal rings; Lt TL and Rt TL indicate the left and right thyroid lobes

Transverse view of the anterior neck depicting the trachea (T), the thyroid lobes, the esophagus (E), the carotid artery (CA), and external jugular vein (JV)
Procedures
Percutaneous Dilation Tracheostomy (PDT)
Percutaneous dilation tracheostomy (PDT) is a commonly performed bedside surgical procedure. Refinement of equipment design, increased experience, and better training have led to enhanced safety of the procedure. However, complications such as perforation of the posterior wall of the trachea, puncture of the esophagus, tracheal ring injury, and vocal cord injury can still occur. Ultrasound can help avoid some of the aforementioned complications, improve safety, and increase the success rate of the procedure especially in the challenging patient [2, 3]. The optimum puncture level for PDT is usually guided by manual palpation of the anatomical landmarks of the anterior neck and endoscopic fiberoptic monitoring. However, this approach has several limitations. Ultrasound scanning of the neck prior to PDT and real-time guided penetration of the trachea may offset some of these shortfalls particularly when anatomical landmarks are not easily identifiable (e.g., in patients with previous tracheostomy, scar tissue, previous surgery or when neck mobility is limited during cervical spine precautions, and morbid obesity) [9, 10].
Abnormal cervical anatomy and obscure landmarks are associated with a higher incidence of peri- and post-procedural complications [11]. Even the use of endoscopic guidance could not avoid tracheostomy tube misplacement above or below the intended insertion site in a quarter of patients signaling poor correlation between actual insertion levels and palpated external landmarks [12].Obese patients undergoing PDT were five times more likely to suffer severe complications despite fiberoptic guidance [13].
Endoscopy offers direct and nearly continuous visualization of the advancing needle, guidewire, dilator, and tube position. However, it does not identify or reveal at-risk vascular structures, thyroid tissue, difficult cervical anatomy, or tracheal depth. Furthermore, endoscopy requires specialized equipment, training, and expertise which are all less likely to be available in any setting than a point-of-care ultrasound machine. Bleeding was observed in 4.8% of PDT patients in one series, and the authors suggested the use of ultrasound to minimize hemorrhagic complications [14]. Kollig et al. changed the planned puncture site in 24% of their patients following ultrasound assessment [15].
Real-time ultrasound-guided PDT was found to be safe in a small series of acute brain injury patient with cervical spine precautions [9]. Obese patients scheduled for PDT present various challenges. A short, thick neck and anatomical difficulties can render the ability to identify relevant external landmarks. Hence, PDT is relatively contraindicated in the morbidly obese patient. In contrast, ultrasound scanning of the obese patient requires considerable experience due to a short thyromental distance, thick pretracheal tissue, limited neck mobility, excessive subcutaneous fat, and poor anatomical landmarks. These factors make image acquisition and interpretation more challenging [10]. Nevertheless, in experienced hands scanning the anterior neck will provide the operator valuable information.
In a recent prospective study, Guinot et al. reported that real-time ultrasound-guided PDT in critically ill obese patients is safe and feasible. The incidence and severity of complications, as well as the time of completion of the procedure, did not differ significantly between obese and non-obese groups. The planned tracheal puncture point was changed in 50% of cases. Of note, ultrasound guidance and identification of cervical anatomy were described as being “difficult” in ten patients (20%), four of which were non-obese [10]. In the TARGET study, patients were randomized to undergo traditional landmark or real-time ultrasound-guided PDT. Ultrasound guidance was associated with significantly higher first needle pass success rate and more accurate tracheal puncture site placement. Fewer complications were observed in the ultrasound group [16].
Indications
The most common indication for PDT is respiratory failure and the need for continued mechanical ventilation. Other indications include upper airway obstruction from trauma, angioedema, malignancy, and obstructive sleep apnea, to facilitate weaning in difficult-to-wean patients and to aid in tracheobronchial toileting.
Contraindications
- 1.
Infection at the site of insertion
- 2.
Unstable patient
- 3.
Infants
- 4.
Unstable cervical spine injury
- 5.
Uncontrollable coagulopathy
Equipment/Probe Selection (Fig. 4.17)
High-frequency (12–5 MHz or higher) linear array probe
A low-frequency (5–1 MHz) probe
Percutaneous dilatational tracheostomy kit (22 gauge needle and syringe; 11 F short punch dilator; 1.32 mm guidewire; 8 F guiding catheter; 18 F, 21 F, 24 F, 28 F, 32 F, 36 F, and 38 F dilators; Shiley no. 8 double-cannula tracheostomy tube)

(a) Linear, (b) curvilinear, and (c) hockey-stick transducers
Preparation/Preprocedural Evaluation
- 1.
The operator stands on the right side of the patient, and the ultrasound monitor is positioned on the opposite side at the appropriate height.
- 2.
The operator should follow sterile precautions.
- 3.
The patient is positioned supine and the neck is extended when possible.
- 4.
Palpate the neck and identify landmark structures; the thyroid cartilage and sternal notch are palpable even in the morbidly obese.
- 5.
Place the transducer transversely in the midline over the thyroid cartilage, and move caudally toward the suprasternal notch. This will reveal the hypoechoic cartilaginous skeleton of the larynx and trachea with overlying thyroid isthmus (Fig. 4.14). Note that cartilages are uniformly hypoechoic, whereas striated muscles and connective tissue produce less homogeneous echoes. However, cartilages may become calcified and thus appear hyperechoic in the elderly. The thyroid gland and other glandular tissue usually appear as slightly hyperechoic (brighter) structures.
- 6.
Select the highest resolution and adjust the image depth, so that the trachea could be seen at the bottom of the screen. The operator should measure the depth of the trachea from the skin, with the neck in a neutral position. This is particularly important when an extended-length tracheostomy tube is to be considered. Tracheal diameter and axis are also evaluated.
- 7.
To obtain a sagittal/longitudinal view, the operator slides the transducer laterally on the neck (either side) while maintaining the edge of the transducer on the tracheal midline and then rotates the transducer 90° onto the sagittal plan. Subtle tilting of the transducer may be needed to optimize image acquisition. The resultant image is described as “pearls on a string” and represents the hypoechoic cricoid cartilage and individual tracheal rings anterior to the hyperechoic air-mucosa interface (Fig. 4.15). The operator should check for vascular structures with color Doppler within the area of interest at the midline to avoid those structures accordingly (Figs. 4.18 and 4.19). Then the optimum puncture site can be marked on the skin.

A transverse scan using a linear probe at the level of the second tracheal ring. An off-midline vein is not uncommon (a). Note the effect of transducer compression upon the vein (b and c)

Transverse scan of the neck with a linear transducer at the level of the third tracheal ring. Images (a) and (b) depict a number of “risk” vessels including a small vein at the possible needle penetration point (observe the probe compression in image b). (c) Color Doppler differentiating artery (pulsating flow) versus vein (orange shadow)
Procedure
- 1.
Dynamic ultrasound guidance enables the visualization of the advancing needle and guidewire, mimicking ultrasound-guided vascular access and locoregional anesthetic procedures.
- 2.
Static preprocedural ultrasound mapping of the neck is performed to provide preliminary information as discussed above.
- 3.
The patient is prepared in a standard method (positioning, anesthesia, local anesthetic, oxygenation and ventilation, monitoring, muscle relaxation, and drapes).
- 4.
The operator should follow full aseptic technique, including sterile transducer sheath and gel.
- 5.
An assistant adjusts the position of the tip of the endotracheal tube (ETT) under direct laryngoscopy, so that the tip is just inferior to the vocal cords. Alternatively, the ETT is replaced with a supraglottic airway device; however, this technique remains debatable as the airway is no longer protected or secured.
- 6.
Hold the transducer in the nondominant hand and obtain a transverse view of the trachea.
- 7.
Select optimum puncture level as discussed mentioned above, ideally between the first and fourth tracheal rings, avoiding vessels or a vascular isthmus in the path of the needle (Figs. 4.20).
- 8.
Insert the tracheostomy puncture needle attached to a saline-filled syringe in the midline perpendicular to the skin, in an out-of-plane approach, and observe the ultrasound monitor for the characteristic acoustic shadow and artifacts due to tissue displacement caused by the advancing needle (Figs. 4.21 and 4.22). As with any needle guidance by ultrasound, an in-plane approach is preferred for its greater precision and safety. However, in some cases space may be limited, and an out-of-plane approach may be necessary as it can be in central line placement on occasion. Anterior tracheal wall indentation is sometimes observed. Typically, with out-of-plane approach, identification and discerning of the needle tip from the shaft can be challenging due to low angle of insinuation.
- 9.
Stop advancing the needle when the needle is seen to penetrate the anterior tracheal wall. This is associated with an obvious change of resistance and a “give.” Aspiration of air confirms intraluminal tip position. Tilt the needle slightly caudally to avoid retrograde passage of the wire guide.
- 10.
Insert guidewire and remove the needle.
- 11.
The guidewire is visualized on transverse and particularly longitudinal views as a distinct hyperechoic signal (Fig. 4.14). Ideal puncture is as close as possible to the midline [17]. Endoscopic examination at this stage will confirm wire entry point and rule out tracheal ring fracture or posterior wall injury. This further enhances the safety of the procedure.
- 12.
Thereafter, proceed with PDT as per standard practice [17].

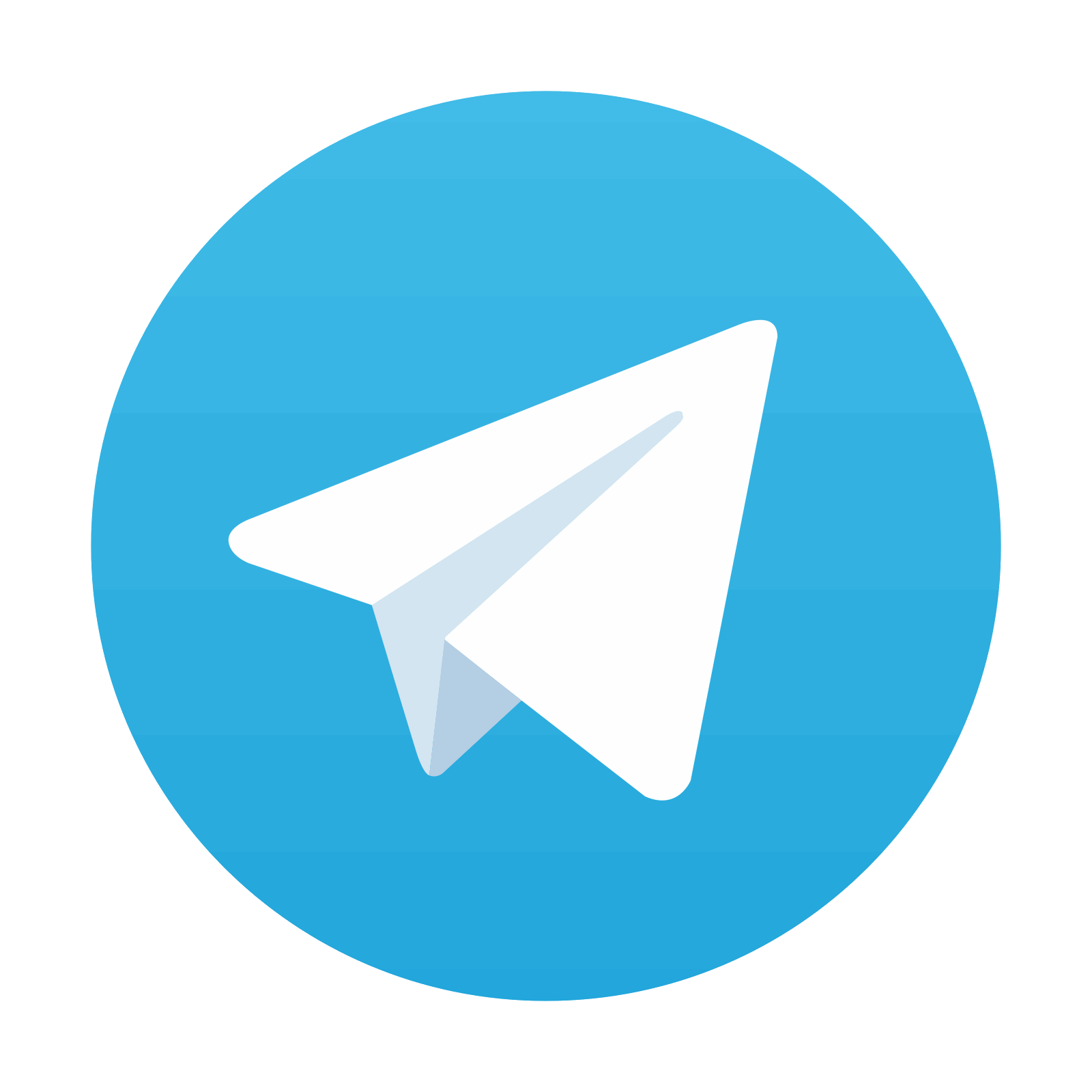
Stay updated, free articles. Join our Telegram channel

Full access? Get Clinical Tree
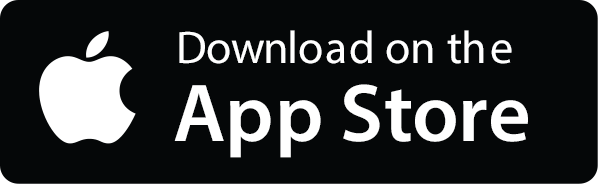
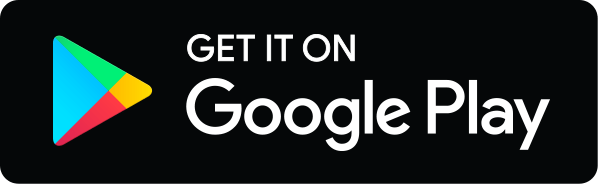