Neuromuscular blockade, Chapter 38, page 286
2) Clinical uses and common agents
a) Reversal of neuromuscular blockade
i) Neostigmine and edrophonium
b) Treatment of myasthenia gravis (1)
i) Pyridostigmine (most common) and neostigmine
c) Treatment of Alzheimer dementia
i) Donepezil, rivastigmine, and galantamine (2)
d) Treatment of central cholinergic syndrome
i) Physostigmine
e) Treatment of glaucoma
i) Echothiophate
(1) Irreversible inhibitor of cholinesterase.
(2) Also inhibits plasma cholinesterase and can increase the duration of succinylcholine (3)
Myasthenia gravis Chapter 94, page 672
1) Pharmacology (4)
a) All three commonly used agents (neostigmine, edrophonium, and physostigmine) follow a two-compartment model after IV injection.
b) All are water-soluble, ionized compounds with excretion by the kidney
4) Reversal of neuromuscular blockade
a) Anticholinesterases are ineffective if such a profound neuromuscular blockade is present that all potential ACh receptors are occupied.
i) It is advisable to wait until as much spontaneous recovery as possible has occurred prior to administering the anticholinesterase (3).
ii) If three or four twitches are observed with train-of-four (TOF) stimulation, recovery with neostigmine will be much more effective and rapid than if one of four twitches is present (5).
b) Evidence of effect
i) Sustained head lift >5 seconds is a very sensitive clinical predictor of adequate reversal of blockade (4).
c) Excessive doses of neostigmine can cause paradoxical neuromuscular relaxation.
Excessive doses of neostigmine can cause paradoxical neuromuscular relaxation.
5) Adverse Effects/Toxicity
a) Predominantly due to the fact that ACh is the neurotransmitter for the entire parasympathetic nervous system.
b) Increased ACh due to cholinesterase inhibition allows ACh to bind parasympathetic muscarinic receptors.
i) Most adverse effects can be abated with the administration of an anticholinergic medication such as glycopyrolate or atropine.
(1) Bradycardia
(2) Bronchospasm
(3) Increased gastrointestinal peristaltic activity
(a) Neostigmine may cause increased nausea and vomiting.
(i) However, this is controversial, and a recent meta-analysis did not show a difference with neostigmine use (6).
(4) Increased bladder tone
(5) Constricted pupils
(6) Diffuse CNS excitation
(a) Seen only with physostigmine, an anticholinesterase that crosses that blood brain barrier
c) Cholinergic crisis
i) Patients present with all of the above muscarinic effects.
ii) Seen most commonly in patients with myasthenia gravis undergoing treatment with anticholinesterase treatment
d) Organophosphate poisoning (4)
i) Anticholinesterases are used as nerve gas agents and pesticides.
ii) Excessive muscarinic activity as described above is observed.
iii) Central nicotinic effects are also seen.
(1) Can result in respiratory depression
iv) The muscarinic effects can be reversed with large doses of atropine.
v) Nicotinic effects require supportive care.
Physostigmine crosses the blood brain barrier and can cause diffuse CNS excitation.
Myasthenia gravis, Chapter 94, page 672
6) Anticholinergic medications used with anticholinesterases (4)
a) Edrophonium has an onset of 1 to 2 minutes and is best used in conjunction with atropine (0.007 mg/kg).
b) Neostigmine’s peak onset is 7 to 11 minutes and is best used with glycopyrolate 0.007 mg/kg or atropine 0.015 mg/kg.
Chapter Summary for Anticholinesterases

References
1. Bhatt JR, Pascuzzi RM. Neuromuscular disorders in clinical practice: case studies. Neurol Clin 2006;24(2):233–265.
2. Masterman D. Cholinesterase inhibitors in the treatment of Alzheimer’s disease and related dementias. Clin Geriatr Med 2004;20(1):59–68.
3. Naguib M, Lien CA. Pharmacology of muscle relaxants and their antagonists. In: Miller RD, ed. Anesthesia. 6th ed. Philadelphia, PA: Churchill Livingstone; 2005:481–572.
4. Donati F, Bevan DR. Neuromuscular Blocking Agents. In: Barash PG, ed. Clinical Anesthesia. 6 ed. Philadelphia, PA: Lippincott Williams & Wilkins; 2009: 498–530.
5. Kirkegaard H, Heier T, Caldwell JE. Efficacy of tactile-guided reversal from cisatracurium-induced neuromuscular block. Anesthesiology 2002;96(1):45–50.
6. Cheng CR, Sessler DI, Apfel CC. Does neostigmine administration produce a clinically important increase in postoperative nausea and vomiting? Anesth Analg 2005;101(5):1349–1355.
Benzodiazapines
Andrea J. Fuller, MD
Benzodiazapines are sedative and hypnotic drugs that can be used for anxiolysis, sedation, and induction of anesthesia.
1) Overview
a) Benzodiazapines are widely used preoperative, intraoperative, and postoperative medications.
b) An overview of this class of drugs will be provided here. For more information on specific drugs, including dosing guidelines, refer to the Appendix of Drug Information, Chapters 193 and 205.
Appendix of drug information, Chapter 193, page 1276
2) Mechanism of action (1)
a) Benzodiazepines bind to specific receptor sites on the alpha subunits of the gamma-aminobutyric acid (GABA) receptor.
i) Binding of benzodiazapines enhances the affinity of the receptor for GABA.
ii) This causes increased chloride conductance and hyperpolarizes postsynaptic neurons, resulting in inhibition of synaptic transmission.
b) Structure/activity relationships
i) Chemical structure is a benzene ring fused to a seven-membered diazepine ring, hence the name, benzodiazepine.
(1) Representative medications include midazolam, lorazepam, temazepam, alprazolam, diazepam, oxazepam
c) Pharmacokinetics
i) Absorption
(1) Rapidly absorbed from the gastrointestinal tract with oral dose
(2) Rapidly enter the CNS and other highly perfused organs with IV injection
ii) Distribution
(1) Highly lipid soluble
(2) Highly protein bound (to albumin)
(a) Disease states that cause hypoalbuminemia (cirrhosis, malnutrition, chronic renal failure) result in increased proportion of unbound drug and lower dosage required to achieve the desired effect.
d) Metabolism (1)
i) Drugs with active metabolites include diazepam, midazolam (metabolized by hepatic microsomal enzymes)
ii) Drugs without active metabolites include lorazepam, oxazepam, temazepam (metabolized by glucuronidation only)
Diazepam and midazolam are metabolized by hepatic microsomal enzymes and have active metabolites.
1) Special considerations
a) Benzodiazapines decrease cerebral metabolic oxygen requirements (CMRO2) and cerebral blood flow (CBF)
b) Unlike barbiturates and propofol, midazolam treatment does not result in an isoelectric EEG.
c) May cause anterograde amnesia (decreased ability to acquire new information)
4) Clinical uses
a) Preoperative anxiolysis (especially for pediatric patients)
b) Anticonvulsant
c) Sedation for procedures or critical care
d) Alcohol detoxification
e) Skeletal muscle relaxation (spinal cord mediated)
i) Not effective for surgical relaxation
f) Treatment of acute insomnia
5) Adverse effects/toxicity (1,2)
a) Dependence
b) Fatigue
c) Drowsiness
d) Decreased ventilation (dose dependent)
i) Most pronounced in patients with chronic pulmonary disease and in conjunction with other respiratory depressants
e) Potentiates the sedative effects of other medications (especially opiates)
f) Decreased systemic vascular resistance with large (i.e., anesthetic induction) doses
g) May inhibit platelet aggregation (midazolam)
Benzodiazepines potentiate the sedative effects of other medications (especially opioids).
6) Treatment of toxicity (1,2)
a) Flumazenil is a specific benzodiazepine antagonist. It reverses benzodiazepine effects, including ventilatory depression.
i) Titrate the drug for the individual, with an initial dose of 4 to 20 mg/kg IV (0.2 mg) and additional doses of 0.1 mg given at 1 minute intervals up to 1 mg. Typically effective doses are 0.3 to 0.6 mg.
ii) Duration of action is 30 to 60 minutes. May need to be repeated depending on the duration of action of the benzodiazepine administered. Alternatively, an infusion of 0.1 to 0.4 mg/h can be used.
iii) May precipitate withdrawal seizures in patients on antiepileptic medications
iv) May increase intracranial pressure in head-injured patients
Flumazenil is a specific benzodiazepine antagonist used to treat benzodiazepine overdose.
Chapter Summary: Benzodiazepines

References
1. Stoelting RK, Hiller SC. Pharmacology and Physiology in Anesthetic Practice. Philadelphia, PA. Lippincott Williams & Wilkins; 2006: 140–154.
2. Van Hemelrijck J, White PF. Nonopoid intravenous anesthesia. In: Barash PG, Cullen BF, Stoelting RK, eds. Clinical Anesthesia. 3rd ed. Philadelphia, PA: Lippincott Williams & Wilkins; 1997:311–328.
Opioid Analgesics and Antagonists
Andrea J. Fuller, MD
Opioids are medications that are used for pain management and adjuncts to anesthesia and critical care. Opioids are the cornerstone medication for the treatment of moderate to severe pain and are commonly used in the perioperative care of patients to provide analgesia. This chapter provides a brief overview of the mechanism of action and various routes of administration. For dosing guidelines, refer to the Appendix of Drug Information.
1) Mechanism of action (1)
a) Opioids bind to opioid receptors, which couple to G proteins and inhibit adenyl cyclase.
i) Leads to inhibition of voltage-gated calcium channels and opening of potassium channels
ii) Results in increased intracellular potassium, which leads to decreased neurotransmission
b) Receptor subtypes. The physiologic function of opioid receptors is to bind endogenous opioids and provide endogenous analgesia in response to pain
i) Mu receptors are in the brain, spinal cord, and periphery and are the primary receptors responsible for the analgesic and adverse effects of opioids, especially morphine.
(1) Mu1 subtype—analgesia
(2) Mu2 subtype—hypoventilation, bradycardia, and physical dependence
ii) Kappa receptors inhibit neurotransmission via type N calcium channels.
(1) Responsible for dysphoria and diuresis
iii) Delta receptors modulate mu receptor activity.
(1) Endogenous opioids include enkephalins, endorphins, and dynorphins.
Appendix B: Opioids, Chapter 194, page 1278
2) Structure/activity relationships
a) The term opiate is used to describe compounds derived from the poppy (Papaver somniferum).
i) The prototype opiate is morphine.
b) Opioid medications
i) Completely synthetic or semisynthetic modifications of morphine
c) Representative opioid medications
i) Hydromorphone, meperidine, fentanyl, alfentanil, sufentanil, and remifentanil
1) Administration of opioids
a) There are multiple routes of administration, including oral, parenteral, intramuscular, neuraxial, transdermal, and transmucosal.
b) Parenteral administration
i) Distribution
(1) Onset and duration of action depend on the lipid solubility and ionization of the drug.
(2) Drugs diffuse across the blood–brain barrier and redistribute to inactive tissue sites such as skeletal muscle and fat.
(3) High doses of lipid soluble drugs (i.e., fentanyl) lead to saturation of inactive sites and buildup of the drug in the plasma, resulting in a longer duration of action.
(1) Most opioids are metabolized in the liver and excreted by the kidney.
(2) An exception is remifentanil, which is rapidly metabolized by plasma esterases.
(3) Many opioids have long-acting, pharmacologically active metabolites.
(a) Normeperidine is the active metabolite of meperidine and has renal elimination.
(i) Accumulation can cause seizures and should be used with caution in patients with renal failure.
(b) Morphine-6-glucuronide is an active metabolite of morphine and can accumulate in neonates and those with renal failure.
c) Neuraxial Administration
i) Neuraxial opioids act on mu receptors in the substantia gelatinosa of the spinal cord.
ii) Epidural administration requires diffusion across the dura to bind receptors in the spinal cord.
iii) Spinal administration requires lower doses because diffusion is not necessary.
(1) The typical spinal dose to produce a desired effect is five to ten times less than the epidural dose.
iv) Pharmacokinetics
(1) Lipid-soluble opioids (fentanyl) have more IV absorption and shorter duration of action.
(2) Water-soluble opioids (morphine) have a longer onset but longer duration, presumably due to increased time needed to diffuse across the dura.
(3) The duration of action depends on diffusion of the drug out of the cerebrospinal fluid (CSF) CSF and into the blood and not on metabolism.
v) Effects of neuraxial opioids
(1) Analgesia
(2) Pruritus
(3) Urinary retention
(4) Hypoventilation
(a) May be delayed up to 12 hours, especially after neuraxial morphine administration
(5) Hypothermia
(6) Nausea and Vomiting
d) Transdermal/transmucosal administration
i) Both routes avoid first-pass metabolism
ii) Fentanyl can be administered via either route due to its high potency and solubility in both water and lipids.
iii) The transmucosal route offers the advantage of rapid onset.
iv) The transdermal route is frequently used for treatment of chronic pain
Obstetrical anesthesia Chapter 144, page 1047
Patients who have received neuraxial morphine should have respiratory monitoring due to the risk of delayed respiratory depression.
Hypothermia can occur after neuraxial opioids and may be treated with naloxone.
4) Special considerations and drug interactions
a) The combination of monoamine oxidase inhibitors and meperidine can result in a severe reaction including fever, convulsions, hemodynamic instability, and coma.
Meperidine should be avoided in patients with renal failure, history of seizures, and those taking monoamine oxidase inhibitors.
5) Clinical uses (1)
a) Pain management
b) Sedation for procedures or critical care
c) Anesthesia for cardiac surgery (high doses)
d) Cough suppression
e) Adjuncts to anesthesia
i) The use of opioids leads to decreased MAC requirements for volatile anesthetics.
6) Adverse effects/toxicity (1,2)
a) Physical dependence
b) Drowsiness
c) Decreased ventilation
i) Opioids produce a dose-dependent change in ventilatory response to increased PaCO2
d) Potentiation of sedative effects of other medications
e) Analgesic tolerance
i) May limit clinical utility
f) Bradycardia (dose dependent)
i) Meperidine is an exception. It has an atropine-like structure and tends to cause tachycardia.
g) Skeletal muscle rigidity
i) Especially seen with rapid IV administration
ii) Ventilation may be difficult and require administration of a muscle relaxant
h) Histamine Release
i) Most commonly associated with morphine
ii) Causes vasodilation and hypotension
i) Miosis
j) Decreased gastrointestinal (GI) motility
k) Decreased fetal heart rate variability when administered to pregnant patients
Acute pain management, Chapter 149, page 1085
7) Agonist/antagonists
a) Representative drugs in this class: nalbuphene, buprephenone, and butorphanol
b) Agonist/antagonist medications have complex interactions with receptors
c) Analgesic effects when used alone but with a ceiling effect, beyond which increased doses do not produce desired results or adverse effects
i) Can result in analgesia with minimal respiratory depression
d) When combined with a full agonist acts as an antagonist
i) Nalbuphene 2.5 to 10 mg IV is very effective in relieving pruritus induced by neuraxial opioids.
The opioid agonist/antagonist nalbuphene is very effective for the treatment of pruritus due to neuraxial opioids.
8) Treatment of toxicity
a) Naloxone is a specific opiate receptor antagonist, binding the receptor without causing activation.
b) The effective dose is 1 to 4 µg/kg IV, and the duration of action is 30 to 45 minutes.
i) Dose may need to be repeated or an infusion started (5 µg/kg/h) after administration of a long-acting opiate.
c) Side effects of naloxone
i) Reversal of analgesia, nausea, vomiting, and increased sympathetic nervous system activity, including tachycardia, hypertension, pulmonary edema, and cardiac dysrhythmias.
Patients who have received a long-acting opioid who require opioid antagonism may require repeat doses or an infusion of naloxone.
Chapter Summary for Opioid Analgesics and Antagonists

References
1. Stoelting RK, Hiller SC. Pharmacology and Physiology in Anesthetic Practice. Philadelphia, PA: Lippincott Williams & Wilkins; 2006.
2. Coda BA. Opioids. In: Barash PG, Cullen BF, Stoelting RK, Cahalan MK, Stock MC, eds. Clinical Anesthesia. 6th ed. Philadelphia, PA: Lippincott Williams & Wilkins; 2009:465–497.
3. Fukuda K. Intravenous opioid anesthetics. In: Miller RD, ed. Miller’s Anesthesia. 6th ed. Philadelphia, PA: Churchill Livingstone; 2005.
Nonsteroidal Anti-inflammatory Drugs and Acetaminophen
Andrea J. Fuller, MD
Nonsteroidal anti-inflammatory drugs (NSAIDs) are very commonly used medications and are widely available to patients without prescription as over-the-counter formulations. NSAIDs can be useful adjuvant drugs when given in conjunction with other anesthetic agents but have specific renal, gastrointestinal (GI), hematologic, and hepatic toxicity that can limit their use.
1) Overview
a) NSAIDs are used for pain management and treatment of inflammatory conditions such as arthritis.
b) An overview of this class of drugs is provided here. For more information on specific drugs, refer to the Appendix of drug information, Chapter 196.
NSAIDs inhibit COX, the enzyme responsible for converting arachidonic acid to prostaglandins.
a) Prostaglandins are compounds that mediate a wide variety of physiologic processes, including platelet aggregation and inflammation.
b) NSAIDs inhibit cyclooxygenase (COX), the enzyme responsible for converting arachidonic acid to prostaglandins.
c) There are two types of COX: one that is always functional, or constitutive (COX-1), and one that is inducible (COX-2).
1) Structure/Activity Relationships
a) Two types of NSAIDs
i) Nonspecific COX inhibitors (i.e., aspirin, ketorlac, ibuprofen, acetaminophen)
ii) COX-2 inhibitors such as rofecoxib, valdecoxib, celecoxib
b) Chemical structure
4) Pharmacokinetics
a) All are well absorbed and have low first-pass metabolism.
b) All are highly protein bound and have small volumes of distribution.
c) NSAIDs are metabolized in the liver and excreted by the kidney
5) Special considerations
a) COX-2 inhibitors(1)
i) Since COX-2 is inducible mainly at inflammatory sites and not involved with platelet aggregation, COX-2 inhibitors were developed to treat inflammation and pain. COX-2 inhibitors are as effective as conventional (nonselective) COX inhibitors at treating pain.
ii) Reports of increased incidence of myocardial infarction and cerebral vascular accidents with long-term use prompted rofecoxib to be withdrawn from the market.
iii) Valdecoxib was also withdrawn from the market because of its association with life-threatening skin reactions and possibly increased cardiovascular events.
iv) Celecoxib is still in use and does not appear to have a higher incidence of cardiovascular events compared with traditional NSAIDs (3).
b) Acetaminophen
i) Acetaminophen is an effective analgesic and antipyretic that lacks anti-inflammatory properties.
ii) Does not cause gastric irritation or inhibition of platelet aggregation
iii) May contribute to analgesic induced nephropathy
iv) Large doses (single dose > 15 g or > 4 g/d) can cause hepatic necrosis and death.
(1) Hepatotoxicity is possible at lower doses in the presence of alcohol abuse.
(2) N-Acetyl cystine antagonizes the hepatotoxic effects and is an effective antidote when given within 8 hours of overdose.
Large doses of acetaminophen can cause hepatic necrosis and death.
a) Postoperative pain management
b) Analgesia
c) Closure of patent ductus arteriosis in neonates (indomethacin)
d) Long-term treatment of various forms of arthritis, including osteoarthritis, rheumatoid arthritis, and ankylosing spondylitis
e) Treatment of fever
f) Treatment of primary dysmenorrhea
g) Treatment of familial adenomatous polyposis (celecoxib)
7) Adverse effects/toxicity
a) Renal impairment
b) Bleeding
i) Due to platelet inhibition
ii) Aspirin causes irreversible platelet inhibition
c) Gastrointestinal irritation
i) May lead to gastric bleeding
d) Asthma (in susceptible individuals)—possibly due to blocking synthesis of prostaglandin E2
e) Hypertension (small effect)
Chapter Summary for NSAIDS

References
1. Stoelting RK, Hiller SC. Pharmacology and Physiology in Anesthetic Practice. Philadelphia, PA: Lippincott Williams & Wilkins; 2006: 140–154.
2. Van Hemelrijck J, White PF. Nonopoid intravenous anesthesia. In: Barash PG, Cullen BF, Stoelting RK, eds. Clinical Anesthesia. 3rd ed. Philadelphia, PA: Lippincott Williams & Wilkins; 1997:311–328.
3. Daiani EZ, Islam K. Cardiovascular and gastrointestinal toxicity of selective COX-2 inhibitors in man. J Physiol Pharmacol 2008; 58 (Sup 2)117–33.
Intravenous Induction Agents
Jerry Ingrande, MD, MS
Intravenous (IV) induction agents are medications given at the beginning of an anesthetic to induce loss of consciousness. Each has its advantages and disadvantages. The choice of agent is determined by the procedure and the patient’s coexisting diseases.
1) Propofol
a) Propofol (2,6,-diisopropylphenol) is a water-insoluble IV anesthetic clinically available as a 1% emulsion containing 10% soybean oil, 2.25% glycerol, and 1.2% egg phosphatide. It is widely used, especially for ambulatory surgery, due to its fast onset and rapid recovery pharmacokinetic profile.
b) Mechanism of action
i) Selective GABA receptor agonist
c) Clinical use
i) Induction
(1) Propofol is given as an IV bolus (1.5 to 2.5 mg/kg) for induction of general anesthesia (1).
(2) Although not reported to induce histamine release, it should be used cautiously in persons with egg allergy.
(a) Pain after injection has been reported to occur in 28% to 90% of subjects (2).
(b) The mechanism of venous pain is unclear; however, its incidence is greater when propofol is given through small veins on the dorsum of the hand as opposed to large veins in the antecubital fossa.
ii) Maintenance
(1) Propofol is commonly used as a continuous infusion for maintenance of both general anesthesia (100 to 200 μg/kg/min) and conscious sedation (25 to 75 μg/kg/min) (1).
(2) Supplementation with nitrous oxide can reduce maintenance requirements
d) Effects on major organ systems
i) Central nervous system
(1) Decreases cerebral blood flow (CBF), cerebral metabolic activity, and intracranial pressure (ICP) (3)
(2) Large doses may decrease cerebral perfusion pressure secondary to decreases in CBF.
ii) Cardiovascular system
(1) Causes direct myocardial depression and decreased systemic vascular resistance (SVR) that are both dose and concentration dependent (1)
(a) Doses should be reduced for the elderly or those who are volume-depleted.
(2) Attenuates the baroreflex mechanism (4)
iii) Respiratory System
(1) Produces respiratory depression, causing apnea in approximately 25% of subjects after an induction dose.
(2) There is a decreased ventilatory response to hypercarbia and hypoxia, which is exaggerated in the presence of opioids.
iv) Other effects
(1) Propofol has antiemetic properties that popularize its use for outpatient procedures, or for maintenance of anesthesia in subjects who are prone to postoperative nausea and vomiting.
(2) It does not trigger malignant hyperthermia and is considered the induction and maintenance agent of choice in susceptible patients (1).
e) Pharmacokinetics
i) Propofol’s rapid recovery profile after a bolus dose is attributed to its rapid distribution from the vessel-rich group (i.e., brain), to inactive tissues (muscle and fat) (5).
(1) Initial distribution half-life is 1 to 8 minutes with an elimination half-life of 2 to 24 hours (1).
(2) Although propofol has a high hepatic extraction ratio, changes in hepatic blood flow have little effect on its pharmacokinetics (6).
(3) Metabolites are cleared via the kidneys.
The addition of local anesthetics (i.e., 1% to 2% lidocaine) to propofol may decrease pain upon injection.
Because propofol causes direct myocardial depression and decreases SVR, doses should be significantly reduced in the elderly or volume depleted.
The incidence of pain on injection of propofol is greater when given through the small veins on the dorsum of the hand.
2) Thiopental
a) Thiopental [5-ethyl-5-(1-methyl-butyl)-2-thiobarbituric acid] is a highly alkaline derivative of barbituric acid with a pKa of 7.6 and pH of 9 (1).
i) Reconstitution of thiopental with acidic solutions (i.e., lactated Ringers; nondepolarizing neuromuscular blocking drugs) may result in precipitation of the compound.
b) Mechanism of action
i) Like propofol, the sedative-hypnotic actions of thiopental are due to its agonism of the GABA receptor.
c) Clinical use
i) Induction
(1) The induction dose of thiopental is 3 to 5 mg/kg in adults and 5 to 6 mg/kg in children (1).
(2) Not commonly given in multiple doses or via infusion because of the potential for saturation of inactive tissues (muscle, fat), resulting in delayed awakening. Pain upon injection is rare.
d) Effects on major organ systems
i) Central nervous system
(1) Thiopental reduces cerebral metabolic oxygen requirements, CBF, electroencephalogram (EEG) activity, and ICP (3).
(2) The reduction of cerebral metabolic oxygen requirement exceeds CBF.
(a) Thiopental is used for neuroprotection during periods of incomplete ischemia (carotid endarterectomy, cerebral aneurysm clipping, cardiopulmonary bypass) (7).
(b) There is no benefit in the setting of global cerebral ischemia (8).
ii) Cardiovascular system
(1) Decreases cardiac output, SVR, and causes direct myocardial depression (1)
(a) The decreased cardiac output is a function of decreased venous return secondary to peripheral vasodilatation.
(b) Dose should be reduced in patients with hemodynamic compromise.
iii) Respiratory system
(1) Causes dose-dependent respiratory depression, with depression of central ventilatory centers (3)
(2) Decreases the ventilatory response to hypercarbia
e) Pharmacokinetics
i) The rapid induction of anesthesia after a bolus dose of thiopental is attributed to its rapid distribution into the effect site (9).
ii) Redistribution of drug from the central compartment into a large peripheral compartment reservoir accounts for the rapid awakening after a single induction dose (9).
iii) Thiopental has a low hepatic extraction ratio, and hepatic clearance is dependent on the intrinsic metabolic activity of the liver (1).
Because thiopental is highly basic, it can cause tissue necrosis when administered through an arterial catheter or subcutaneously (e.g., an infiltrated IV catheter).
1) Etomidate
a) Etomidate (R-1-ethyl-1-[a-methylbenzyl] is an imidazole-containing IV induction agent that undergoes intramolecular rearrangement at physiologic pH (1).
i) Because of its instability at physiologic pH, etomidate is formulated in a 0.2% aqueous solution containing 35% propylene glycol.
b) Mechanism of action
i) The mechanism for induction of hypnosis by etomidate is not fully understood but thought to be largely related to GABA and may be similar to the mechanism of action of propofol.
c) Clinical use
i) Induction
(1) Etomidate is given as an IV bolus (0.2 to 0.4 mg/kg) for induction of general anesthesia (1).
(2) Not commonly given in multiple doses or as a maintenance infusion secondary to adrenal suppression
d) Effects on major organ systems
i) Central nervous system
(1) Reduces cerebral metabolic oxygen requirement, CBF, and ICP (3)
(2) Cerebral perfusion pressure is maintained because of the hemodynamic stability after etomidate administration (1).
ii) Cardiovascular system
(1) Minimal changes in BP, SVR, HR, and cardiac output after administration (10)
(2) For these reasons, etomidate is often administered to patients with cardiopulmonary compromise.
iii) Endocrine System
(1) Etomidate causes inhibition of 11-beta-hydroxylase, resulting in adrenocortical suppression after its administration (1).
(a) Adrenal suppression occurs after administration of etomidate and may persist for 5 to 8 hours after a single bolus dose (11).
(2) This must be taken into consideration in patients dependent on an intact stress response (i.e., septic shock or hemorrhage).
iv) Other effects
(1) Associated with a high incidence of postoperative nausea and vomiting
(2) The propylene glycol formulation results in significant pain upon injection.
(3) Associated with myoclonus after bolus doses, which can be attenuated with prior administration of opioids
e) Pharmacokinetics
i) Moderately lipid soluble and almost entirely unionized at physiologic pH, allowing for rapid effect site distribution and subsequent redistribution to peripheral tissues.
(1) This accounts for the rapid induction and rapid awakening after a single IV bolus (3).
ii) Metabolism occurs via hydrolysis of the ester side chain in the liver.
4) Ketamine
a) Ketamine is a water-soluble compound with a pKa of 7.5. It is structurally related to phencyclidine.
b) Mechanism of action
i) Interacts with the NMDA receptor, in addition to opioid, and nicotinic and muscarinic acetylcholine receptors
ii) Ketamine’s analgesic, amnestic, and psychomimetic effects are thought to be due to antagonism of the NMDA receptor.
c) Clinical use
i) Induction
(1) Ketamine is given as an IV bolus dose of 1 to 2 mg/kg for induction of general anesthesia.
(2) For patients without IV access, 4 to 8 mg/kg may be given intramuscularly (1).
ii) Other uses
(1) Ketamine is also used in low doses as an adjunct to narcotics for pain relief, especially for chronic pain (1). Doses of 0.1 to 0.5 mg/kg are often given for this purpose.
d) Effects on major organ systems
i) Central nervous system
(1) Produces a dissociative anesthetic state characterized by analgesia and amnesia while consciousness is maintained.
(2) High incidence of psychomimetic reactions after awakening from ketamine.
(3) Unlike the other induction agents, ketamine increases cerebral metabolic oxygen requirement, CBF, and ICP (1).
(a) It is therefore contraindicated in patients with intracranial pathology.
ii) Cardiovascular system
(a) Produces a sympathomimetic effect with an associated increase in HR, BP, and cardiac index (1)
(b) Can cause direct myocardial depression and in the absence of autonomic control, these effects may be unmasked (12)
iii) Respiratory system
(1) Ketamine has direct bronchodilator properties.
(a) The induction agent of choice in the setting of acute bronchospasm (1)
(2) In contrast to the other IV induction agents, ketamine is most likely to preserve protective airway reflexes.
(3) An increase in oral secretions may require concomitant use of an antisialogogue.
e) Pharmacokinetics
i) Highly lipid-soluble, which facilitates its rapid transfer into the brain (3). This, combined with the drug-induced increase in CBF, is responsible for its rapid onset of action.
ii) Rapidly redistributed to peripheral tissues.
iii) Duration of ketamine-induced anesthesia is approximately 10 to 20 minutes after a single bolus dose (1).
iv) Metabolized by the cytochrome P-450 system to form norketamine, an active metabolite
v) It has a high hepatic extraction ratio, and alterations in hepatic blood flow may affect the metabolism of ketamine.
Ketamine can increase CMRO2, CBF, and ICP and is contraindicated in patients with intracranial pathology.
5) Dexmedetomidine
a) Dexmedetomidine is a selective alpha-2 adrenoreceptor agonist with an alpha-2 to alpha-1 ratio of 1,600:1.
b) The anxiolytic and hypnotic responses are associated with its effects in the locus ceruleus, and are mediated by the alpha-2A receptor (13).
c) Clinical use
i) Sedation
(1) Dexmedetomidine is approved for use as a continuous infusion (0.2 to 1 μg/ kg/h) for sedation of mechanically ventilated patients in the ICU (1).
(2) Dexmedetomidine is not used for induction of general anesthesia.
(3) It is used “off-label” for sedation during diagnostic and therapeutic procedures such as fiberoptic intubations and as an IV adjuvant during regional anesthesia.
d) Effects on major organ systems
i) Central nervous system
(1) Dexmedetomidine has sedative, anxiolytic, and analgesic effects.
(a) The level of sedation is comparable to that of midazolam (14).
(2) The analgesic effects of dexmedetomidine reduce postsurgical opioid requirements.
ii) Cardiovascular system
(1) Increases the potential for hypotension and bradycardia because of its high alpha-2 adrenoreceptor selectivity (1).
(2) Dexmedetomidine blunts the hemodynamic effects of direct laryngoscopy and improves hemodynamic stability when given as an anesthetic adjunct during neurosurgical procedures.
iii) Respiratory system
(1) Produces less ventilatory depression compared to other sedative-hypnotic and opioid anesthetics (15).
(2) Bolus administration does reduce minute ventilation (16).
e) Pharmacokinetics
i) Short distribution half-life (6 minutes)
ii) Short elimination half-life (2 hours) (13)
iii) Metabolized in the liver and eliminated by the kidneys
(1) Clearance is decreased with hepatic failure (13).
iv) Age and sex do not affect pharmacokinetic parameters
Induction Doses and Physiologic Effects of IV Induction Agents
↑ increase; ↓ decrease; ↔ no change/minimal change. CBF, cerebral blood flow; CMRO2, cerebral metabolic rate for oxygen; ICP, intracranial pressure; SVR, systemic vascular resistance; HR, heart rate; MV, minute ventilation; RR, respiratory rate
Chapter Summary for IV Induction Agents

References
1. Barash PG. Clinical Anesthesia. 6th ed. Philadelphia, PA: Wolters Kluwer/Lippincott Williams & Wilkins; 2009.
2. Smith I, White PF, Nathanson M, et al. Propofol. An update on its clinical use. Anesthesiology 1994;81:1005–1043.
3. Stoelting RK, Hillier S. Pharmacology & Physiology in Anesthetic Practice [print]. 4th ed. Philadelphia, PA: Lippincott Williams & Wilkins; 2006.
4. Sellgren J, Ejnell H, Elam M, et al. Sympathetic muscle nerve activity, peripheral blood flows, and baroreceptor reflexes in humans during propofol anesthesia and surgery. Anesthesiology 1994;80:534–544.
5. Shafer A, Doze VA, Shafer SL, et al. Pharmacokinetics and pharmacodynamics of propofol infusions during general anesthesia. Anesthesiology 1988;69:348–356.
6. Servin F, Desmonts JM, Haberer JP, et al. Pharmacokinetics and protein binding of propofol in patients with cirrhosis. Anesthesiology 1988;69:887–891.
7. Todd MM, Chadwick HS, Shapiro HM, et al. The neurologic effects of thiopental therapy following experimental cardiac arrest in cats. Anesthesiology 1982;57:76–86.
8. Randomized clinical study of thiopental loading in comatose survivors of cardiac arrest. Brain Resuscitation Clinical Trial I Study Group. N Engl J Med 1986;314:397–403.
9. Russo H, Bressolle F. Pharmacodynamics and pharmacokinetics of thiopental. Clin Pharmacokinet 1998;35:95–134.
10. Gooding JM, Corssen G. Effect of etomidate on the cardiovascular system. Anesth Analg 1977;56:717–719.
11. Wagner RL, White PF. Etomidate inhibits adrenocortical function in surgical patients. Anesthesiology 1984;61:647–651.
12. Schwartz DA, Horwitz LD. Effects of ketamine on left ventricular performance. J Pharmacol Exp Ther 1975;194:410–414.
13. Maze M, Scarfini C, Cavaliere F. New agents for sedation in the intensive care unit. Crit Care Clin 2001;17:881–897.
14. Alhashemi JA. Dexmedetomidine vs. midazolam for monitored anaesthesia care during cataract surgery. Br J Anaesth 2006;96:722–726.
15. Venn RM, Hell J, Grounds RM. Respiratory effects of dexmedetomidine in the surgical patient requiring intensive care. Crit Care 2000;4: 302–308.
16. Bloor BC, Ward DS, Belleville JP, et al. Effects of intravenous dexmedetomidine in humans. II. Hemodynamic changes. Anesthesiology 1992;77:1134–1142.
Inhaled Anesthetic Agents
Ellen Choi, MD
Inhaled anesthetic agents are used to provide general anesthesia for patients. They provide analgesia, amnesia, and skeletal muscle relaxation, which allow ideal surgical conditions. The exact mechanism of action is unknown. Nitrous oxide (N2O) and halogenated volatile anesthetic compounds are the most commonly used.
1) Pharmacokinetics
a) The goal of inhalation anesthesia is to achieve optimal and constant partial pressure of anesthetic in the brain (Pbr).
i) In order to achieve this goal, the anesthetic must go from the anesthesia machine to the tissue (brain).
ii) This creates a gradient that eventually leads to equilibrium between the alveolar partial pressure of the anesthetic (PA) and the partial pressure of the anesthetic in the arterial blood (Pa).
The goal of inhalational anesthesia
iii) Alveolar concentration (FA)
(1) Measure of anesthetic concentration and uptake
(2) FA quickly equilibrates with the concentrations in the blood and brain.
(3) Fi is the concentration of inspired anesthetic.
(4) The rate of rise of FA/Fi determines the speed of inhalational induction.
(a) If FA/Fi increases, then speed of inhalational induction increases.
iv) Factors affecting delivery/input
(1) Concentration of inspired gas (Fi)
(a) Amount of gas that is delivered
(i) Controlled with the anesthetic vaporizer
(2) Fresh gas flow (FGF) rates
(a) Increasing the FGF rates increases the amount of anesthetic agent delivered to the patient.
(3) Breathing circuit
(a) Material of the circuit can cause absorption, decreasing the alveolar concentration of the anesthetic (FA).
(b) The volume of the circuit can affect the amount of gas delivered to the patient (1).
(4) Concentration effect
(a) Increasing the inspired gas concentration (Fi) will cause a more rapid rate of rise of FA/Fi.
(5) Second gas effect
(a) A high concentration of one gas/anesthetic (usually N2O) will accelerate the increase in the FA of another administered gas (volatile anesthetic or O2).
b) Factors affecting uptake
i) Solubility
(1) The solubility of an anesthetic agent can be depicted through the blood: gas coefficient.
(a) The blood:gas coefficient is the ratio of concentration of an agent in blood versus the alveoli (Table 44-1).
Table 44-1
Physiochemical Properties of Volatile Anestheticsa
aAdapted from Barash PG, Cullen BF, Stoelting RK. Clinical Anesthesia. 5th ed. Philadelphia, PA: Lippincott Williams & Wilkins; 2006:386.
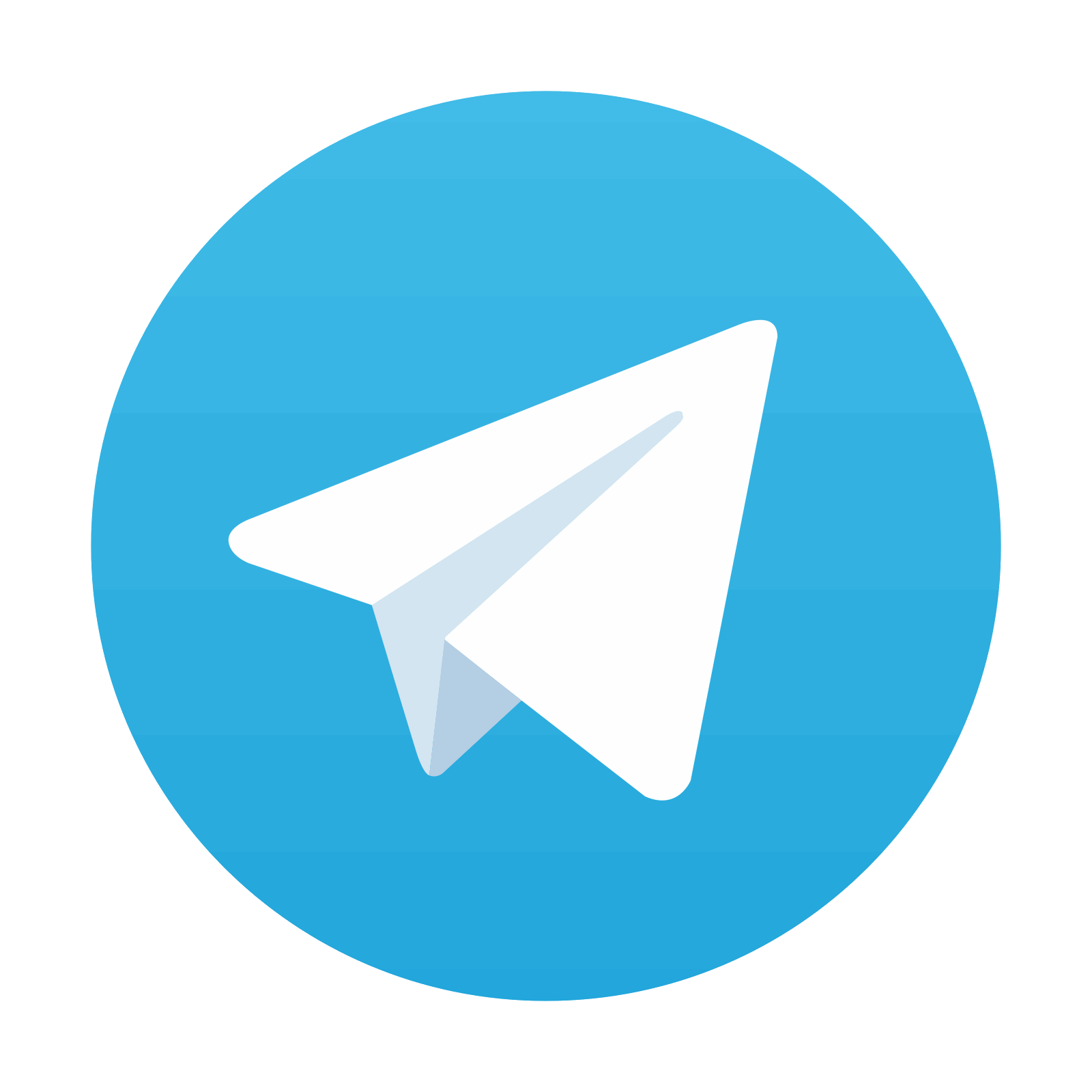
Stay updated, free articles. Join our Telegram channel

Full access? Get Clinical Tree
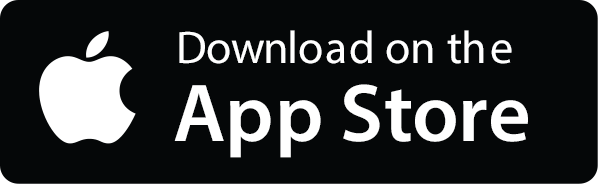
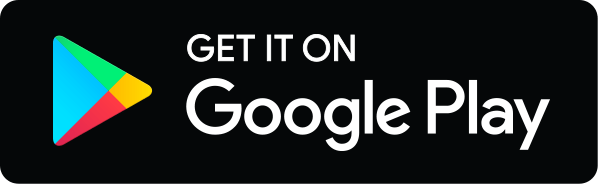