TABLE 113.2 Severity of Breathlessness Assessment According to the Modified British Medical Research Council (mMRC) | |
![]() |
Lung Volume and Diffusing Capacity
Lung volumes can be measured using whole-body plethysmography or gas dilution (helium or nitrogen washout) techniques. In emphysema, both total lung capacity and residual volume may be increased because of loss of lung elastic recoil. The carbon monoxide diffusing capacity may be diminished with the progression of the disease, reflecting the impaired gas exchange due to loss of the functional parenchyma. Although not required for the diagnosis of COPD, both lung volumes and diffusing capacity can be used to characterize its severity. Both measurements are part of the COPD workup especially when techniques such as volume reduction surgery or placement of endobronchial valves are being considered.
Chest Radiographic Findings
Chest radiographic alterations usually occur late in the course of the disease, and there is no alteration pathognomonic of COPD. The radiograph is usually normal in mild disease; changes reflecting airway disease and hyperinflation may appear with disease progression. Sometimes it is possible to see enlarged bronchial walls reflected as an increase in bronchovascular markings. Emphysema is manifested by an increased lucency of the lungs. In smokers, these changes are more prominent in the upper lobes, while in α1-antitrypsin deficiency, they are more likely in basal zones. With hyperinflation, the chest becomes vertically elongated with low, flattened diaphragmatic domes. The heart shadow is also vertical and narrow. The retrosternal airspace is increased on the lateral view, and the sternal-diaphragmatic angle exceeds 90 degrees. Radiographic computerized tomography is more sensitive and specific for the presence of emphysema, but it is rarely required. It is most useful in the preoperative evaluation for lung volume reduction surgery or to plan the placement of endobronchial valves (11,12).
TABLE 113.3 COPD Assessment Test (CAT) | ||
![]() |
TABLE 113.4 Classification of Severity of Airflow Limitation According to GOLD | |
![]() |
Arterial Blood Gases
Both pulse oximetry and arterial blood gases can be used to determine the need of home oxygen therapy. If oxygen saturation is below 92% on pulse oximetry while breathing room air, an arterial blood sample should be obtained. If PaO2 is below 55 mmHg, long-term oxygen therapy is indicated. When between 55 and 59 mmHg, home oxygen is recommended only if there is polycythemia or signs of right heart failure.
EXACERBATION
COPD exacerbation can be defined as an acute event characterized by an increase in dyspnea, cough, or sputum production that requires therapy (13). The two most commonly identified precipitating factors are infection—viral, such as Rhinovirus spp or influenza, and bacterial, such as Haemophilus influenzae, Streptococcus pneumoniae, Moraxella catarrhalis, Enterobacteriaceae spp, or Pseudomonas spp—as well as environmental exposure to air pollutants. However, in about one-third of cases, no underlying cause is identified. Infectious agents can also be recovered from some patients with stable COPD, indicating that in some instances, their presence in decompensated COPD represents an epiphenomenon. On the other hand, the acquisition of a new strain of a bacterial species colonized with a pathogenic bacteria might lead to an exacerbation in stable COPD patients (14). All things considered, the best predictor of future exacerbations is having treated an exacerbation in the past, suggesting the existence of a frequent exacerbation phenotype (15). Other predictors of exacerbation include decreasing lung function, worsening quality of life, a history of gastroesophageal reflux, and an increased white-cell count (14). All exacerbations should be evaluated carefully for their potential to require hospitalization as a result of respiratory failure, which is associated with poor prognosis and increased risk of death. In the following section, we discuss the hospital treatment of COPD exacerbations.
TABLE 113.5 Combined Assessment Using Symptoms, Breathlessness, Spirometric Classification, and Risk of Exacerbations According to GOLD |
![]() |
Assessment and Treatment of Exacerbations
The goals of the treatment of COPD exacerbations are to eliminate or control the cause of the exacerbation, provide optimum bronchodilator therapy, assure adequate oxygenation, and correct respiratory acidemia, all the while avoiding tracheal intubation when possible. Most patients with mild exacerbations can be treated at home, but those with a more severe presentation require hospitalization. Signs of severity include use of accessory muscles, paradoxical breathing pattern, hemodynamic instability, and decreased level of consciousness (1). In-hospital mortality in those with severe exacerbations is approximately 10% to 24% and can reach 59% at 1 year (16,17).
Admission criteria according to the American Thoracic Society/European Respiratory Society guidelines (18) include:
- High-risk comorbidities including pneumonia, cardiac arrhythmia, congestive heart failure, diabetes mellitus, renal failure, or liver failure
- Inadequate response of symptoms to outpatient management
- Marked increase in dyspnea
- Inability to eat or sleep because of symptoms
- Worsening hypoxemia
- Worsening hypercapnia
- Changes in mental status
- Inability to care for oneself (i.e., lack of home support)
- Uncertain diagnosis
Pharmacologic Treatment
The mainstay of pharmacologic treatment is the use of bronchodilators, corticosteroids, and antibiotics, all of which are discussed below.
Bronchodilators
- β2-Agonists: The bronchodilators most commonly used are the inhaled short-acting β2-agonists because of their rapid onset of action. They can be administered via a nebulizer or through metered dose inhalers (MDIs). Typically, two puffs of albuterol or salbutamol are given every 4 hours, or an equivalent dose via nebulizer. During mechanical ventilation, the use of a spacer interposed in the circuit between the tube and the Y-piece is recommended. An unresolved issue relates to dosage when MDIs are used with intubated patients. Fernandez et al. (19) used two puffs, Gay et al. (20) used three puffs, and Fuller et al. (21) used four puffs in their studies. Because the MDI dose deposited in the lungs of intubated patients is, at best, half of the dose deposited in the lungs of ambulatory patients, it seems reasonable to at least double the number of MDI puffs in intubated patients (i.e., at least four puffs). In some patients, this dose will be inadequate, and a greater number of puffs (e.g., 10–20) can be safe and effectively used. In such circumstances, tachycardia—a common and dose-dependent side effect of bronchodilators—should be monitored to avoid β2-agonists overdose. Other potential side effects should be closely followed, such as agitation, tremulousness, hyperlactatemia, hypokalemia, and hyperglycemia.
Long-acting β2-agonists can also be considered. Subcutaneous or intravenous administration should not be used unless there is contraindication for the inhaled route because of their increased systemic effects.
- Anticholinergics: Ipratropium bromide can be used in association with the β2-agonists as needed. It is available both via nebulization (500 μg) or MDI (two puffs every 2–4 hours). There are no clinical studies that have evaluated the use of the long-acting anticholinergic tiotropium bromide during COPD exacerbations.
- Methylxanthines: Methylxanthines are currently not indicated in the treatment of exacerbations of COPD.
Corticosteroids. Steroids are usually recommended for exacerbations of COPD. If feasible, prednisone can be given orally at a dose of 30 to 40 mg/day for 5 days (22). If the oral route is not an option, hydrocortisone or methylprednisolone can be substituted in equivalent doses. Some investigators advocate the use of much higher doses (methylprednisolone, 125 mg intravenously four times daily) (23), but as no studies have been designed to find the optimal dose, we favor the lower dose. The inhaled route can be an option (24,25). The combination of salmeterol, 50 μg, and fluticasone, 500 μg, given twice daily, has been compared with placebo and resulted in a reduction in mortality of 3 years (p = 0.052), fewer exacerbations, and improved health status and lung function. Nebulized budesonide, 1,500 μg four times daily, was compared with prednisolone 40 mg and demonstrated equal efficacy and potentially fewer side effects, especially less hyperglycemia.
Antibiotics. Antibiotics decrease mortality during exacerbations. These agents are indicated when there is increased production or change in the color of the sputum. For mild exacerbations, amoxicillin, sulfamethoxazole-trimethoprim, or doxycycline for 7 to 10 days is usually adequate. Patients requiring hospitalizations should receive penicillin/penicillinase (e.g., amoxicillin/clavulanate), a respiratory quinolone (levofloxacin, moxifloxacin), or a third-generation cephalosporin together with a macrolide (e.g., ceftriaxone plus clarithromycin). In addition to their antimicrobial activity, macrolides possess anti-inflammatory and mucoregulatory properties that may confer beneficial effects to patients with COPD (26).
Respiratory Support
The goal of respiratory support in patients with exacerbations of COPD is to correct hypoxemia/acidemia and reduce the respiratory work, thus avoiding respiratory muscle fatigue (27,28). In the acute setting, oxygen therapy alone is able to resolve hypoxemia, but not acidemia and respiratory distress. For this reason, invasive or noninvasive mechanical ventilation is frequently needed (2,28).
Oxygen Therapy. To improve the hypoxemia commonly present in exacerbations of COPD, controlled oxygen therapy is the cornerstone of hospital treatment (2). Long-term oxygen therapy is established as the standard of care for selected patients with advanced chronic stable hypoxemia due to COPD (29,30). However, in the acute setting, some patients have an impaired response to hypercapnia when treated with supplementary oxygen, leading to worsening of CO2 retention (31,32). The precise mechanism of this impairment is not well understood, but ventilation/perfusion (33–37) and respiratory drive (34,38) disturbances have been implicated. Some evidence suggests that blunting of the hypoxic vasoconstriction response due to the higher oxygen content in poorly ventilated areas may be the culprit of the acute CO2 retention. The increased perfusion of such poorly ventilated, previously hypoxic areas might suddenly increase the shunt effect, transferring a great part of the venous CO2 content directly to the arterial compartment, causing some worsening of hypercapnia and sometimes deterioration of mental status.
There is no individual risk factor that identifies patients with COPD who will evolve to hypercapnia after oxygen exposure (2,31,32); therefore, the National Heart, Lung, Blood Institute/World Health Organization Global Initiative for Chronic Obstructive Lung Disease (GOLD) Workshop summary has recommended controlled oxygen therapy for the exacerbations, where adequate levels of oxygenation—PaO2 of at least 60 mmHg or SaO2 of at least 90%—are easy to achieve in uncomplicated exacerbations. Notwithstanding, CO2 retention can occur insidiously with little change in symptoms; hence, measuring arterial blood gases 30 minutes after the start of oxygen therapy is recommended. Venturi masks are more accurate sources of oxygen than are nasal prongs, but are more likely to be removed by the patient (2). Controlled oxygen therapy must be started at a low inspiratory oxygen fraction—0.24 to 0.28—and titrated upward to reach a PaO2 of at least 60 mmHg or SaO2 of at least 90% without significant retention of CO2. A clinically significant increase in PaCO2 has been arbitrarily defined as a raise in CO2 of 6.5 mmHg, especially if mental status deteriorates (32).
One must always remember that most of these patients have some degree of chronic vascular disease associated with their smoking history, and cardiovascular complications may be frequent during prolonged hypoxic episodes; for example, acute coronary syndromes, atrial fibrillation, cerebral ischemia, and pulmonary congestion. Therefore, the quick reversal of severe hypoxemia is frequently a priority.
Noninvasive Mechanical Ventilation. Patients with COPD are prone to acute hypercapnic respiratory failure, often resulting in emergency admission to the hospital. Between 20% and 30% of patients admitted with hypercapnic respiratory failure secondary to acute exacerbation of COPD will die in the hospital (39–42). Traditionally, patients who do not respond to conventional treatment are given invasive mechanical ventilation despite its well-known risks. Tracheal intubation and assisted ventilation have been associated with high morbidity and mortality rates, in addition to the difficulties during the weaning process from the ventilator (43,44). Many clinical complications seem to arise from the intubation procedure itself, or during the course of mechanical ventilation. The most common complications have been nosocomial infections, aspiration, pulmonary embolism, muscle atrophy, polyneuropathies, electrolyte imbalances, and gastrointestinal bleeding, as well as prolonging the stay in the intensive care unit (45,46).
In view of such difficulties, noninvasive positive pressure ventilation is an alternative treatment for patients admitted to the hospital with hypercapnic respiratory failure secondary to acute exacerbations of COPD. With this ventilatory modality, the patient receives air, or a mixture of air and oxygen, from a flow generator or a special ventilator through a facial/nasal mask, thus avoiding the need for tracheal intubation (40–42,47–49). Many studies have shown that noninvasive positive pressure ventilation increases pH, reduces PaCO2, reduces the severity of breathlessness in the first 4 hours of treatment, and decreases the length of hospital stay (40–42). More importantly, mortality and the intubation rate are consistently reduced by this intervention (40–42). Some studies suggest that the use of proper noninvasive ventilation can reduce the chances of an eventual endotracheal intubation to less than half (0.42, 95% confidence interval [CI] of 0.31 to 0.59) when compared with the conventional treatment with oxygen mask. This alternative has been also associated to a reduced mortality rate (0.41, 95% CI 0.26 to 0.64). In clinical-physiological terms, the expected elevation of pH after 1 hour of treatment should be around 0.03 (95% CI 0.02 to 0.04) and the expected reduction in PaCO2 in the same interval around –3.0 mmHg (95% CI –5.1 to –0.2) (50).
Unfortunately, noninvasive ventilation is not appropriate for all patients (2). Failure rates between 9% and 50% have been reported (51,52). One important signal that this procedure is not working for a patient is the progression—even if slight—of hypercapnia or acidosis 30 to 60 minutes after the procedure, and deterioration of the mental status.
The classic indications for noninvasive mechanical ventilation in exacerbated COPD patients are (1) respiratory distress with respiratory rate above 35 breaths/min; (2) respiratory acidosis with a pH below 7.35, and with normal or high standard base excess; and (3) a PaO2 below 45 mmHg. These measurements are made after the patient has been breathing room air for at least 10 minutes (40,41,53). Noninvasive mechanical ventilation is contraindicated for patients with profound bradypnea, defined as a respiratory rate below 12 breaths/min, severe hypercapnic encephalopathy with Glasgow Coma Scale score below 10, cardiac and/or respiratory arrest, and hemodynamic instability (40,41,53). Some authors, however, have successfully applied noninvasive mechanical ventilation in comatose COPD patients with a Glasgow Coma Scale score below 8, with other causes of coma being ruled out (54). This latter use of noninvasive mechanical ventilation is not a consensus (53), but could be applied during a short-term trial (typically of 30–60 minutes) under continuous surveillance at the bedside. If the respiratory drive is blunted due to narcosis, the addition of mandatory breaths can be helpful.
Recommended settings of noninvasive ventilation vary among the studies. Few authors used an exclusive inspiratory pressure support (41) or inspiratory volume support (40). However, most physicians would recommend the use of positive end-expiratory pressure (PEEP) or continuous positive airway pressure (CPAP). This recommendations is based on the rationale that the use of PEEP/ CPAP further reduces the inspiratory work in patients with COPD exacerbation, especially the extra load generated by high levels of intrinsic PEEP (55–57).
There are many approaches to set the noninvasive ventilation. An easy way is to set the expiratory pressure at 5 cm H2O, the inspiratory pressure at 10 cm H2O—resulting in a “delta P” of 5 cm H2O—and to increase the delta P in increments of 5 cm H2O, up to 20 to 25 cm H2O or the maximum tolerated, over 1 hour (49). An alternative approach is to adjust the inspiratory pressure in order to obtain a tidal volume of 6 to 8 mL/kg and a respiratory rate of 25 to 30 breaths/min, setting the end-expiratory pressure to 5 cm H2O to offset the inspiratory threshold induced by intrinsic PEEP. Close observation of inspiratory time is very important for the success of this strategy, whatever the mode of ventilation. Too short, but especially too long inspiratory time causes great discomfort. Typically, inspiratory time should be set in the range of 0.7 to 1 second. In pressure support mode (PSV), inspiratory time can be optimized by adjusting the cycling-off criterion (automatically adjusted in some mechanical ventilators). Typical settings of this parameter lie within the range of 30% to 50% of peak inspiratory flow.
If inspiratory comfort is not achieved, asynchrony with the ventilator is often observed, with the patient often not able to trigger the assisted breath. Trials of 2 cm H2O elevations in the PEEP/CPAP levels should be performed in order to further reduce the extra load imposed by the intrinsic PEEP. During these trials of augmentation of external PEEP, the minimum inspiratory pressure should be provided to maintain a stable tidal volume (58). Oxygen should be offered to keep oxygen saturation above 85% to 90% (2,49,58).
Theoretically, pure CPAP support in these patients might be of some help, offsetting part of the inspiratory threshold load imposed on COPD patients, caused by intrinsic PEEP. The appeal of such a strategy is the possibility of using low-cost CPAP systems. This approach, however, has not been tested systematically and should be reserved for very special conditions under close supervision. Whenever possible, some level of inspiratory support should always be added to a CPAP strategy, unloading also part of the resistive workload.
Success rates of noninvasive mechanical ventilation for COPD exacerbations can be in the order of 80% to 85% (53). Close monitoring after the start of noninvasive ventilation is very important to recognize early the minority of patients who will fail. Confalonieri et al. (59) evaluated the risk of failure of noninvasive ventilation in 1,033 consecutive patients with exacerbation of COPD admitted to experienced hospital units. In that study, some factors found on admission were associated with a failed attempt at noninvasive ventilation. These risk factors included a Glasgow Coma Scale score <11, an Acute Physiology and Chronic Health Evaluation (APACHE) II score >28, respiratory rate >30 breaths/min, and arterial pH <7.25. The presence of all these risk factors resulted in a predicted risk of failure greater than 70%. An arterial pH below 7.25 after 2 hours of ventilation greatly increased the risk of failure to over 90%. All these numbers and thresholds should be taken as relative reference points, because the success of noninvasive mechanical ventilation depends on a learning curve of the whole staff. The less experienced the staff, the more conservative they should be with these limits, not waiting for further deterioration of the patient before deciding to intubate.
After hospital admission, the correct timing for starting noninvasive ventilatory support is either immediately or at any time the patient shows worsening of the respiratory distress, a fall in PaO2, or an increase in PaCO2 (49,58). Noninvasive ventilatory support can be applied in any area of the hospital where close monitoring of the patient by trained personnel is available, such as intensive care units, emergency departments, high-dependency units, and respiratory wards. The duration of the noninvasive ventilation and the number of possible interruptions for oral and facial cleaning varies according to the patient need. Ventilatory periods lasting at least 40 minutes are warranted (41), and some patients will require uninterrupted use (49,58).
The choice for an appropriate mask is an important aspect of noninvasive mechanical ventilation. In general, patients benefit from a facial mask that covers the mouth and the nose; this is more efficient than the nasal type to deliver effective inspiratory pressures. Leaks directed at the eyes, sores in the nasal area, and dry mouth are frequent causes of extreme discomfort to patients. The total face mask may be better tolerated by some patients, but not all, and greatly reduces skin sores. However, one has to be aware that the anatomic dead space increases with this type of mask, which also imposes some challenges to the mechanical ventilator in terms of synchrony and PEEP maintenance.
High-Flow Oxygen Therapy. By delivering a high flow (typically 20 to 60 L/min) of heated and humidified air through a special nasal prong, at controlled inspiratory fractions (typically from 30% to 80% oxygen), recent studies in patients with acute lung injury (60) have shown benefits that may exceed the benefits of conventional oxygen therapy, or even the benefits of noninvasive ventilation. Studies are under way to prove the specific benefits of this strategy in patients with hypercapnic respiratory failure, and there is a good rationale to expect future benefits also extended to this population of patients with COPD exacerbation. Among the proven physiologic effects of this strategy, which forces some fresh air to flow from the nasal cavity toward the mouth, passing through the pharynx, studies demonstrated: (a) a decrease in the dead space, due to the washout of CO2 accumulated in the nasal cavity, retropalatal cavity, pharynx, and mouth; (b) a CPAP effect that amounts to 3 to 7 cm H2O depending on the flow settings, anatomy of airways, and the maintenance (or not) of a closed mouth; (c) a decrease in minute ventilation demands, which may be related to central effects or just to a decrease in dead space; and (d) an improvement in patient comfort (when compared to pure oxygen or to mask ventilation). Although promising, future trials will provide us with more information about safety and benefits of this new strategy, when compared to the traditional noninvasive ventilation, as described above.
Invasive Mechanical Ventilation. Invasive mechanical ventilation can be either the initial choice in patients with COPD exacerbation or the strategy to be applied after failure of a trial of noninvasive ventilation (2). Mechanical ventilation can reduce or eliminate the work of breathing and improve gas exchange, while allowing the respiratory function to return to baseline through the treatment of the precipitating causes of the acute decompensation (4).
Assuming that all appropriate measures to improve airflow obstruction have already been taken, minimization of dynamic hyperinflation is a key objective of the ventilatory support of these COPD patients. At the bedside, dynamic hyperinflation is typically detected by the presence of nonzero end-expiratory flow in the flow–time curve, or by effectively measuring the end-expiratory pressure (auto-PEEP) after an expiratory pause. Precise quantification of the auto-PEEP, however, is problematic in patients with spontaneous breathing efforts (61).
In some patients, especially in those with predominant emphysema, the airway obstruction in the expiratory phase is disproportionally higher than in the inspiration. In these patients, the measured auto-PEEP is higher than expected when considering the calculated inspiratory airway resistance. This situation can be anticipated by looking at the flow–volume curve available on most ventilators. During pressure-controlled ventilation, the slopes of the inspiratory and expiratory curves are proportional to the time constant of the respiratory system (a higher slope meaning lower resistance), and the differences between inspiratory and expiratory airway resistances can thus be determined (Fig. 113.1) (62).
To reduce the hyperinflation, several concepts should be employed alone or in combination (4,63). The most effective strategy is controlled hypoventilation (64), which decreases dynamic hyperinflation through the reduction of the minute volume. Hypoventilation, with a fixed inspiratory time, decreases the expiratory flow requirement and consequently reduces air trapping and plateau pressures (64–66). An appropriate clinical goal at present is to keep the plateau pressures no higher than 30 cm H2O, a strategy associated with lower rates (4%) of barotrauma (67,68). Adequate sedation and analgesia help by lowering the production of CO2 and allowing further reduction of the minute volume (4). At the bedside, the general rules to minimize hyperinflation are to keep the minute volume 8 L/min or less and to keep the expiratory time at at least 4 seconds; low respiratory rates should be used, for example, 8 to 10 breaths/min, with 5 to 8 mL/kg of tidal volume. Once these goals have been achieved, there is probably little gain from further adjusting the ventilator. For example, Leatherman et al. (69) showed that halving minute ventilation from 7.4 to 3.7 L/min, and more than doubling expiratory time from 4.5 to 9.5 seconds had no significant effect on auto-PEEP and plateau pressure (63). Although controlled hypoventilation is the most effective measure to decrease hyperinflation, this ventilatory strategy frequently worsens CO2 retention. The hypercapnia and acidosis are generally well tolerated and considered acceptable by most clinicians (4,63,64), provided that such levels of hypoventilation are essential to keep plateau pressures below 30 cm H2O.

FIGURE 113.1 Example of a flow–volume curve of a patient mechanically ventilated with severe and equivalent inspiratory and expiratory flow limitation. The dashed lines represent the slope of the curves and are proportional to the inspiratory and expiratory time constants.
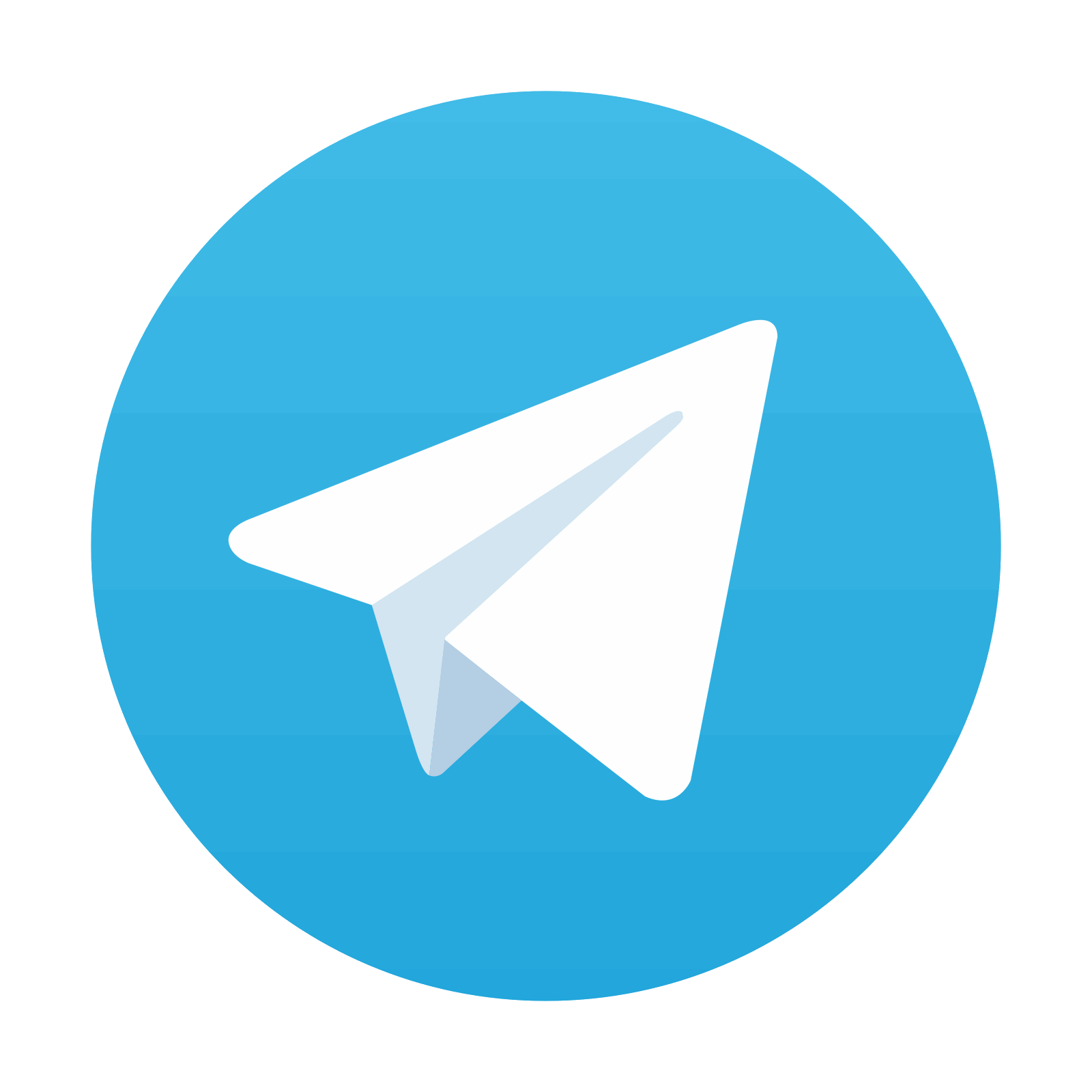
Stay updated, free articles. Join our Telegram channel

Full access? Get Clinical Tree
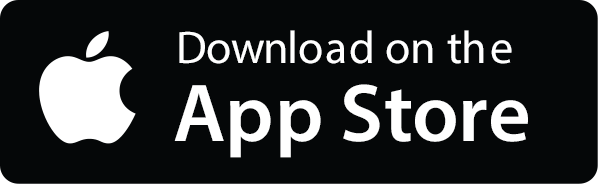
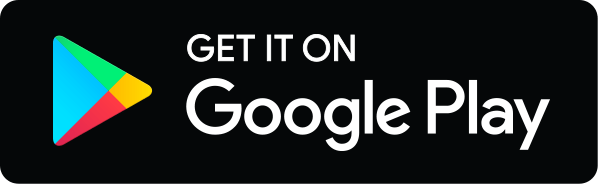