EPIDEMIOLOGY
In a recent systematic review of 312 studies including 49 million patients, the syndrome of AKI (according to KDIGO definition) was found to affect 1 in 5 adults and 1 in 3 children during hospital admission (17). A degree of acute renal injury (manifested by release of NGAL, albuminuria, loss of small tubular proteins, or inability to excrete a water load, sodium load or an amino acid load, or any combination of the above) can be demonstrated in most ICU patients. Using the RIFLE classification, the incidence of at least some degree of AKI has been reported as high as 67% in a study of more than 5,000 critically ill patients (3). The development of AKI with a maximum RIFLE category Failure has been reported in up to 28% of critically ill patients and is associated with a several fold increased risk of in-hospital death (2,3). Recent trends have suggested that the incidence of AKI may be increasing, in particular in critically ill patients. This may be attributable to improved recognition and reporting with the introduction of consensus definitions; however, this is also likely attributable to changes in population demographics, baseline susceptibilities, and comorbid diseases, and greater exposure to more complex and technologically advanced procedures and treatments (i.e., immune suppression; chemotherapy; organ transplantation; cardiac surgery).

FIGURE 132.1 Histogram showing the effect of experimental sepsis in sheep on the fractional excretion of sodium (FeNa). FeNa decreased in sepsis as would be expected during decreased perfusion. In fact, all experimental animals had a twofold to threefold increase in renal blood flow, providing proof of the concept that FeNa cannot be used to infer renal hypoperfusion.
TABLE 132.2 Laboratory Tests to Differentiate Prerenal AKI from Established AKI | ||
![]() |
Several risk factors for AKI in ICU patients have been identified including baseline (nonmodifiable) susceptibilities and potentially modifiable events or factors. These risk factors include older age, male sex, preexisting comorbid illness, a diagnosis of sepsis, cardiogenic shock, major surgery (specifically cardiac surgery), hypovolemia, and exposure to nephrotoxic drugs (18,19). In addition, multiorgan dysfunction, specifically concomitant acute circulatory, pulmonary, and hepatic organ dysfunction, is commonly associated with AKI (Table 132.4) (20–22).
PRESENTATION AND CLINICAL CLASSIFICATION
The clinical presentation of AKI may vary according to the etiology and the severity. AKI may be clinically silent until there are overt changes to serum creatinine (which is dependent on serum creatinine being routinely monitored in “at-risk” patients) and/or urine output. Urine output is often, but not always, decreased. As kidney function worsens, electrolyte disturbances such as hyperkalemia, hyperphosphatemia, metabolic acidosis due to diminished clearance of acid, and fluid accumulation occur. In those patients not carefully monitored or untreated, disruption of metabolic and fluid balance homeostasis can lead to life-threatening complications such as ventricular dysrhythmias and pulmonary edema.
TABLE 132.3 Urinary Sediment Scoring System (16) | |
![]() |
The contributing factors for AKI can largely be categorized into conditions that alter renal hemodynamics, conditions that cause direct kidney injury, or conditions that contribute to the obstruction of urine flow. Often patients will present with multiple concurrent and temporally associated contributing factors for AKI. The early identification of precipitating causes is essential for limiting the extent of ongoing injury and promoting repair and recovery.
Alternation to kidney hemodynamics, due to either or both systemic and regional factors, is likely the most common etiology for AKI in the ICU. These include events that affect systemic hemodynamics by causing decrease of extracellular volume (i.e., hypovolemia, dehydration, hemorrhage, burns), redistribution of that volume (i.e., capillary leak in sepsis, pancreatitis, or hepatic failure), events associated with decreased cardiac output (i.e., myocardial infarction, heart failure, septic cardiomyopathy), or those associated with low perfusion pressure (i.e., anaphylaxis). Kidney function can also be impacted by alterations to regional kidney hemodynamics. Processes that alter afferent (i.e., nonsteroidal anti-inflammatory drugs) and/or efferent arteriolar tone (i.e., ACE inhibitors/ARB) can adversely impact glomerular filtration.
Less commonly, AKI can present where the principal source of damage is within the kidney and where typical structural changes can be seen on microscopy. Numerous disorders, which affect the glomerulus or the tubule, may be responsible. Among these, nephrotoxins are particularly important, especially in hospitalized patients (23). The most common nephrotoxic drugs affecting ICU patients are listed in Table 132.5. Many cases of drug-induced AKI rapidly improve upon removal of the offending agent. Accordingly, a careful history of drug administration is essential in all patients with AKI.
TABLE 132.4 AKI Risk Factors |
![]() |
TABLE 132.5 List of Medications Known to Be Associated with Nephrotoxicity (26) | ||
![]() |
More than a third of patients who develop AKI in ICU have chronic kidney disease (CKD) due to factors such as age-related changes in nephron mass, longstanding hypertension, diabetes mellitus, or atheromatous disease of the renal vessels. Such CKD may be manifest by a prehospital elevation in serum creatinine or evidence of proteinuria; however, this may not be universally evident or known. Often, what may seem to the clinician as a relatively trivial kidney insult may unmask more significant AKI due to a lack of renal functional reserve related to subclinical pre-existing CKD.
Obstruction to urine outflow is a common cause of functional AKI in the community (24), but is uncommon in the ICU. The pathogenesis of obstructive AKI involves several humoral responses as well as mechanical factors. Typical causes of obstructive AKI include bladder neck obstruction from an enlarged prostate, ureteric obstruction from pelvic tumors or retroperitoneal fibrosis, papillary necrosis, or large calculi. The clinical presentation of obstruction may be acute or acute-on-chronic in patients with longstanding renal calculi. It may not always be associated with oliguria. If obstruction is suspected, ultrasonography can be easily performed at the bedside. However, not all cases of acute obstruction have an abnormal ultrasound and, in many cases, obstruction occurs in conjunction with other renal insults (e.g., staghorn calculi and severe sepsis of renal origin). The use of medications that lead to urinary retention in those without an indwelling urinary catheter may exacerbate the presentation. Assessment of the role of each factor and overall management should be conducted in conjunction with an urologist. Finally, the sudden and unexpected development of anuria in an ICU patient should always suggest obstruction of the urinary catheter as the cause. Appropriate flushing or changing of the catheter should be implemented in this setting.
Clinical risk prediction tools can be utilized to identify patients at greater risk of AKI. Those at high-risk patients can be monitored closely for development of AKI including frequent assessments of serum creatinine, continuous urine output monitoring, and strict avoidance of nephrotoxins. For example, Kheterpal et al. (25) developed and validated a clinical risk score for AKI for patients undergoing general surgery. Five classes of risk were created based on the presence of 11 preoperative risk factors. The risk index showed progressively greater AKI risk with higher class, with a 9% risk in those in Class V (at least six risk factors) compared to a 0.2% risk in those with Class I (up to two risk factors).

FIGURE 132.2 Histogram showing the effect on renal blood flow of experimental sepsis in sheep. Renal blood flow increased threefold while creatinine increased from 80 to 400 μmol/L, providing evidence that acute renal failure in sepsis can occur during renal hyperemia.
Modern clinical information systems can be designed to trigger alerts to clinicians for “at-risk” patients who are developing early AKI or who are exposed to unnecessary nephrotoxins. Recently, the use of automated alerting from clinical information systems that can integrate bedside information such as urine output, laboratory information such as absolute and relative changes in serum creatinine, and pharmacy information, such as potential nephrotoxin exposure have been shown to improve the recognition of AKI in hospitalized patients. Electronic alerting has been shown to translate into earlier interventions, reduce severity among those developing AKI, and improve outcomes (26,27).
PATHOGENESIS OF SPECIFIC SYNDROMES
Septic AKI
Sepsis is a leading predisposing factor to AKI in critically ill patients (19). Epidemiologic studies estimate between 45% and 70% of all AKI encountered in the ICU is associated with sepsis (18,19,28). The distinction between septic and nonseptic AKI may have particular clinical relevance considering recent evidence to suggest that septic AKI may be characterized by a unique pathophysiology (29–32).
The classic teaching is that sepsis brings about hypotension, leading to a reduction in critical organ blood flow including in the kidney, causing ischemic injury and AKI. Furthermore, sepsis would lead to activation of the sympathetic nervous system, stimulating the release of potent vasoconstrictors that induce renal vasoconstriction and aggravate kidney ischemia, thus worsening AKI. However, growing evidence questions this ischemic-induced paradigm of septic AKI (29,30,32). An experimental study in a large mammalian model of hyperdynamic sepsis found that RBF was marked increased above baseline despite significant reductions in kidney excretory function (Fig. 132.2) (31). These findings are supported by small clinical studies of resuscitated patients with septic AKI that also show increases in RBF (33–35). The implications are that in hyperdynamic sepsis, AKI is hyperemic, rather than ischemic, with global RBF considerably increased. Moreover, experimental studies have shown that regional cortical and medullary RBF are preserved in sepsis and can be further augmented by infusion of norepinephrine (Fig. 132.3) (36). This concept of hyperemic AKI in sepsis is consistent with the relative paucity of renal histopathologic evidence of tubular necrosis in patients with septic AKI (37).
Thus, evolving evidence suggests that the pathogenesis of septic AKI predominantly involves toxic and immune-mediated mechanisms. Sepsis is known to release a vast array of pro- and anti-inflammatory mediators such as cytokines (damage-associated molecular proteins [DAMPs] and pathogen-associated molecular proteins [PAMPs], arachidonic acid metabolites, and thrombogenic agents that all may participate in the development of AKI (38). Similarly, experimental studies have found evidence of renal tubular cell apoptosis in response to inflammatory mediators in endotoxemia (39,40). Renal tubular apoptosis may prove an important mechanism of septic AKI in critically ill patients (37,41,42). No studies exist to tell us which of the above mechanisms are most important and when they might be active in the course of an episode of septic AKI. However, interventions with antiapoptotic properties, such as with selective caspase inhibitors, may theoretically aid in attenuating kidney injury and promote recovery of function (38). To date, however, no human randomized controlled trials have assessed the impact of these interventions on kidney function and their value is unknown.

FIGURE 132.3 Histogram showing the effect of norepinephrine on mean arterial blood pressure (MAP) compared to high-dose dopamine in a randomized controlled trial in humans. MAP is more reliably restored using norepinephrine when given alone as an alternative to high-dose dopamine or after high-dose dopamine has failed. BSL, baseline.
AKI in Association with Major Surgery
AKI is a common complication following major surgery (19, 43). The incidence is variable and dependent on the prevalence of pre-existing comorbid illnesses, preoperative kidney function, and the type and urgency of surgery being performed. Numerous intraoperative events can act to negatively affect kidney function, including the following:
- Hemodynamic instability (e.g., intravenous or inhaled anesthetic agents)
- Hypovolemia due to blood loss or third spacing
- Details of the operative field (e.g., aortic cross-clamping in major vascular surgery)
- Increases in intra-abdominal pressure (e.g., laparoscopic insufflation of CO2)
- Concomitant sepsis
- Use of nephrotoxin drugs
Any of these factors, alone or in combination, may contribute to a critical reduction in RBF and ischemia, impaired oxygen delivery, and toxin- or inflammatory-mediated injury. Postoperative AKI is believed to be, in part, mediated by proinflammatory mechanisms such as increased endothelial cell adhesion, tubular cell infiltration, generation of reactive oxygen species, proinflammatory cytokines, and reperfusion injury (44,45). Cardiac surgery with cardiopulmonary bypass (CPB) commonly induces early postoperative AKI. The mechanisms whereby CPB causes injury are incompletely understood, although there is a suggestion that CPB is proinflammatory, activating components of the nonspecific immune system. In turn, this leads to oxidative stress with the generation of oxygen-free radical species and serum lipid peroxidation products (46). In addition, CPB has been shown to deplete serum antioxidative capacity for a prolonged duration after surgery. Such oxidant stress has been shown to directly induce kidney injury in experimental studies (47).
Surgery-specific clinical prediction tools have been developed and validated to assess risk and predict AKI after surgery. Such tools can be utilized to identify patients at greater risk of perioperative AKI prior to planned procedures or among those requiring urgent or emergent procedures. These patients can be triaged to higher levels of perioperative monitoring if indicated (i.e., high-dependency units or intensive care; frequent assessments of serum creatinine; continuous urine output monitoring; strict avoidance of nephrotoxins).
Hepatorenal Syndrome
This condition is a form of AKI, which typically occurs in the setting of advanced cirrhosis; however, it can occur with severe liver dysfunction due to alcoholic hepatitis or other forms of acute hepatic failure (48). The recent consensus definition of HRS includes any form of kidney disease occurring in patients with cirrhosis regardless of etiology (Table 132.6) (49). The pathogenesis of hepatorenal syndrome (HRS) is incompletely understood; however, there are several potential mechanisms that may contribute to HRS, including activation of the renin–angiotensin system in response to systemic hypotension, activation of the sympathetic nervous system in response to systemic hypotension and increased intrahepatic sinusoidal pressure, increased release of arginine vasopressin due to systemic hypotension, and reduced hepatic clearance of various vascular mediators such as endothelin, prostaglandins, and endotoxin (48,50).
TABLE 132.6 Summary of the Diagnostic Criteria for Kidney Dysfunction in Chronic Liver Disease and Hepatorenal Syndrome (49) | |
![]() |
Although HRS can occur spontaneously in patients with advanced cirrhosis, it is important to recognize that other precipitants are much more common. These include sepsis, specifically spontaneous bacterial peritonitis (SBP), raised intra-abdominal pressure due to tense ascites, bile acid nephropathy in those with severe hyperbilirubinemia, gastrointestinal bleeding, and hypovolemia due to paracentesis, diuretics and/or lactulose, or any combination of these factors. Likewise, other contributing factors to AKI should be routinely assessed for including cardiomyopathy due to alcoholism, nutritional deficiencies or viral infection, and exposure to nephrotoxins.
Typically, HRS develops in patients with advanced cirrhosis and evidence of portal hypertension with ascites in the absence of other apparent causes of AKI. It generally presents as oligo-anuria with progressive increases in serum creatinine and/or urea and bland urinary sediment. These patients develop profound sodium and water retention with evidence of hyponatremia, a urine osmolality higher than that of plasma, and a very low urinary sodium concentration (<10 mmol/L).
Management of the patient with HRS can be challenging. However, it should include the systematic identification and prompt treatment of potential reversible precipitants (i.e., sepsis). The avoidance of hypovolemia by albumin administration in patients with SBP has been shown to decrease the incidence of AKI in a randomized controlled trial (51). These causes must be looked for and promptly treated. Some studies suggest that vasopressin derivatives (i.e., terlipressin) may improve GFR in this condition (52,53). Other treatment options include oral α-adrenergic agonist midodrine with subcutaneous octreotide or referral for albumin-based extracorporeal therapies. Placement of a transjugular intrahepatic portosystemic stent-shunt (TIPS) has been associated with modest improvements in kidney function in those with HRS, may improve outcome and represent a measure for those who are not a candidate for or awaiting transplant (54,55). In general, the ideal solution for reversal of AKI in these patients is either improvement in hepatic function by therapy for the underlying primary liver disease and/or referral for and successful liver transplantation.
AKI with Rhabdomyolysis
The incidence of rhabdomyolysis-induced AKI is estimated at 1% in hospitalized patients but it may account for close to 5% to 7% of cases of AKI in critically ill patients depending on the setting (23,28). Its pathogenesis involves the interplay of prerenal, intrarenal, and postrenal factors including concurrent hypovolemia, ischemia, direct tubular toxicity mediated by the heme pigment in myoglobin, and intratubular obstruction (56). The causes of muscle injury that can result in rhabdomyolysis include major trauma, burns, drug overdose (i.e., narcotics, cocaine, or other stimulants), vascular embolism, prolonged seizures, neuroleptic malignant syndrome, various infections (i.e., pyomyositis, necrotizing fasciitis, influenza, HIV), severe exertion, impaired cellular energy production (i.e., hereditary enzyme disorders, toxins), increased calcium influx (i.e., malignant hyperthermia), alcoholism, and in response to a variety of agents which can interact to induce major muscle injury (i.e., combination of macrolide antibiotics or cyclosporin and statins). The clinical manifestations of rhabdomyolysis include an elevated serum creatine kinase and evidence of pigmented granular casts and red to brown coloring of the urine. Patients can also have various electrolyte disorders as a result of muscle breakdown including hyperphosphatemia, hyperkalemia, hypocalcemia, and hyperuricemia.
The principles of prevention of AKI include identification and elimination of potential causative agents and/or correction of underlying compartment syndromes; prompt and aggressive fluid resuscitation and maintenance of polyuria (i.e., ≥1.5 to 2 mL/kg ideal or adjusted body weight per hour, usually more than about 300 mL/hr to restore vascular volume and potentially flush obstructing cellular casts); and urine alkalinization to a goal pH above 6.5 in order to reduce renal toxicity by myoglobin-induced lipid peroxidation and improve the solubility of myoglobin (56). Experimental studies have suggested mannitol may act as a scavenger of free radicals and reduce cellular toxicity; however, the role of forced diuresis with mannitol remains controversial. Other controversial therapies include allopurinol; deferoxamine; dantrolene, and glutathione, and high volume and/or high cut-off membrane hemofiltration.
AKI due to Nephrotoxins
Several mechanisms have been reported to play a role in the development of kidney injury after exposure to nephrotoxins. Particular drugs can often invoke a variety of pathophysiologic effects on the kidney that collectively contribute to AKI. Alterations in intra-renal hemodynamics are an important initial consequence of many nephrotoxins. These changes to regional renal blood flow may occur through increased activity of local vasoconstrictors such as angiotensin II, endothelin, adenosine; at the same time, there is diminished activity of important vasodilators (i.e., nitric oxide and prostaglandins). This imbalance can lead to renal vasoconstriction and ischemia, particularly to susceptible regions such as the outer medulla, for example, in response to radiocontrast media, or can induce humorally mediated vasoconstriction of afferent arterioles as a result of exposure to NSAIDs and cyclosporine. The end result of a reduction in regional blood flow is a critical reduction in oxygen delivery, thus predisposing to tubular hypoxia (57). In addition, nephrotoxins can directly contribute to impaired tubular metabolism and oxygen utilization. They lead to generation of radical oxygen species including superoxide anions, hydrogen peroxide, hydroxyl radicals, reduction in intrinsic antioxidant enzyme activity, accumulation of intracellular calcium, mitogen-activated protein kinases, and phospholipase A2, for example, after exposure to aminoglycosides (58–60).
These responses to nephrotoxins can induce tubular cell vacuolization, interstitial inflammation, altered cell membrane properties, and disruption of normal tubular adhesion to basement membranes. Failure of these mechanisms contributes to tubular cell apoptosis, necrosis and tubular sloughing into the luminal space, cast formation, and obstruction (58). Raised intraluminal pressures due to obstruction, altered cellular permeability, and interstitial inflammation can contribute back diffusion of fluid and secondary edema formation.
Radiocontrast media and aminoglycosides are leading agents contributing to nephrotoxin-induced AKI (61,62). Radiocontrast media–induced toxicity is believed to occur from the interplay of alterations in renal hemodynamics due to vasoconstriction, increased intravascular viscosity and erythrocyte aggregation, direct tubular epithelial cell toxicity, and concomitant atheroembolic microshowers in the renovasculature. Aminoglycosides are taken up via organic anion transport systems in the proximal tubules where they accumulate and generate radical oxygen species, increased intracellular calcium, which lead to tubular apoptosis, necrosis, and nonoliguric AKI.
Radiocontrast-Induced Nephropathy
Radiocontrast-induced nephropathy (RCIN) is the leading cause of iatrogenic AKI in hospitalized patients and results in prolonged hospitalization, higher mortality rates, excessive health care costs, and potentially long-term kidney impairment (23). RCIN presents with an acute rise in serum creatinine within 24 to 48 hours following injection of radiocontrast media. The serum creatinine level generally peaks within 3 to 5 days and returns toward baseline within 7 to 10 days; however, in some patients kidney function may not return to baseline and a persistent reduction in function may occur. RCIN is often associated with pre-existing risk factors, in particular pre-existing CKD (GFR <60 mL/min/1.73 m2), a diagnosis of diabetes mellitus, and use of large quantities of radiocontrast media.
There are few effective prophylactic or therapeutic interventions with evidence for reducing the occurrence of radiocontrast nephropathy and no therapy has proven effective once it is established (63). Strategies for prevention include early identification of patients at risk, consideration to either delay of the investigation, or using alternative modality until kidney function can be optimized. Likewise, every effort should be made correct volume depletion and discontinue potential nephrotoxins. There is no evidence to support the routine use of diuretics, mannitol or dopamine. Studies have shown that periprocedure hydration and use of nonionic iso-osmolar (e.g., iodixanol) radiocontrast media can reduce the risk (64–67). Several randomized trials and meta-analyses have suggested potential benefit with the use of N-acetylcysteine or sodium bicarbonate; however, further definitive evidence is pending (68,69). Their effectiveness in already fluid-resuscitated ICU patients, however, remains unknown.
AKI in Association with Mechanical Ventilation
Most critically ill patients require mechanical ventilation (MV), either for disease-specific indications such as acute respiratory distress syndrome (ARDS) or simply for routine postoperative care. The application of positive pressure MV, particularly with positive end-expiratory pressure (PEEP), can have important physiologic effects on kidney function. Experimental and clinical studies have clearly established an association between MV and PEEP and alterations in kidney function. This can occur through several mechanisms, including alterations in cardiovascular function, alterations in neurohormonal activation, abnormalities in gas exchange, and alterations in systemic inflammatory mediators (70,71).
The positive pressure applied during MV acts to increase intrathoracic, intrapleural, and intra-abdominal pressures, both during inspiration and for the duration of the respiratory cycle. This increase in intrathoracic pressure, monitored clinically by changes in mean airway pressure, can act to reduce intrathoracic blood volume, decrease transmural pressure, reduce right ventricular preload, increase right ventricular afterload, exert alterations to pulmonary vascular resistance and volume, and contribute to changes in left ventricular filling and geometry. The result of these effects may be a decrease in cardiac output and renal perfusion. Similarly, raised intrathoracic pressure, by altering transmural pressures and reducing cardiac output, can act to unload intrathoracic baroreceptors. This initiates a cascade of compensatory neurohormonal events characterized by increased systemic and renal sympathetic nervous activity, increased activation of the renin–angiotensin–aldosterone system, increased secretion of vasopressin, and a reduction in release of atrial natriuretic peptide. These culminate in altered renal perfusion and kidney excretory function. Renal function may not be impaired per se with MV, but rather, may appropriately respond to stimuli by reducing osmolar, sodium, and water clearance. In addition, acute hypoxemia and/or hypercapnia, both commonly encountered in patients with ARDS, can act to alter systemic hemodynamics and increase systemic inflammation, both of which may exert negative effects on renal perfusion and function. Particular strategies of MV, specifically in ARDS, are now recognized to contribute to or provoke ventilator-induced lung injury (VILI). Evidence now suggests that the pathophysiology of VILI is multifactorial and results from the combined effects of volutrauma (excessive tidal or end-expiratory volumes), barotrauma (excessive end-inspiratory peak and plateau pressures), atelectatic trauma (cyclical opening and closing of alveolar units), and biotrauma (local release of inflammatory mediators from injured lung) (72). Such injurious MV can initiate a cascade of events that increases systemic inflammation and adversely impact kidney function (73).
AKI in Association with Intra-Abdominal Hypertension/Abdominal Compartment Syndrome
Intra-abdominal hypertension (IAH) and abdominal compartment syndrome (ACS) can worsen kidney function and precipitate AKI. Mechanical compression on abdominal and thoracic vessels results in diminished venous return, congestion, increased renal venous pressure, which leads to renal interstitial edema. This coupled with renal arterial vasoconstriction due to compensatory renin–angiotensin–aldosterone system activation contributes to impaired perfusion pressure across the renal circulation. Patients with IAH progressing to ACS commonly present with marked abdominal distension, measured IAP above 15 mmHg, and increasing serum creatinine and oliguria. ACS is commonly associated with major trauma or large burn injuries, complex intra-abdominal surgeries, pancreatitis or ruptured aneurysms, and in patients receiving large volume resuscitation.
Identification of IAH/ACS requires a high index of suspicion among susceptible patients, and should include the routine measurement of bladder pressures. Management is generally supportive and includes optimization of sedation, neuromuscular blockade, patient repositioning, nasogastric and rectal decompression, draining fluid collections and optimizing fluid balance through minimizing nonessential fluid, administration of diuretics or if necessary, initiation of RRT. In those with refractory ACS and sustained IAH above 20 mmHg, surgical decompression should be considered (74).
GENERAL MANAGEMENT
The most common clinical picture seen in the ICU is that of a patient who has sustained or is experiencing a major systemic insult such as trauma, sepsis, myocardial infarction, severe hemorrhage, cardiogenic shock, or major surgery. When the patient arrives in the ICU, resuscitation is typically well underway, or surgery may have just been completed. Despite such efforts, the patient is already anuric or profoundly oliguric, and the serum creatinine is rising, and a metabolic acidosis is developing; serum potassium and phosphate levels may be rapidly rising as well. In these critically ill patients with AKI, multiple organ dysfunction—with the need for MV and vasoactive drugs—is common. Fluid resuscitation is typically undertaken in the ICU with the guidance of invasive hemodynamic monitoring. Vasoactive drugs are often used to restore mean arterial pressure (MAP) to acceptable levels, typically greater than 65 to 70 mmHg (see Figure 132.3). The patient may improve over time, and urine output may return with or without the assistance of diuretic agents (Fig. 132.4). If urine output does not return, however, RRT needs to be considered. If the cause of AKI has been removed, and the patient has become physiologically stable, slow recovery occurs within 4 to 5 days to as long as 3 or 4 weeks. In some cases, urine output can be above normal for several days. If the cause of AKI has not been adequately remedied, the patient remains gravely ill, the kidneys do not recover, and death from multiorgan failure may occur.

FIGURE 132.4 Diagram showing the effect of norepinephrine on urine output compared to high-dose dopamine in patients in septic shock. Urine output is more effectively restored with norepinephrine infusion when given alone as an alternative to high-dose dopamine or after high-dose dopamine has failed. BSL, baseline.
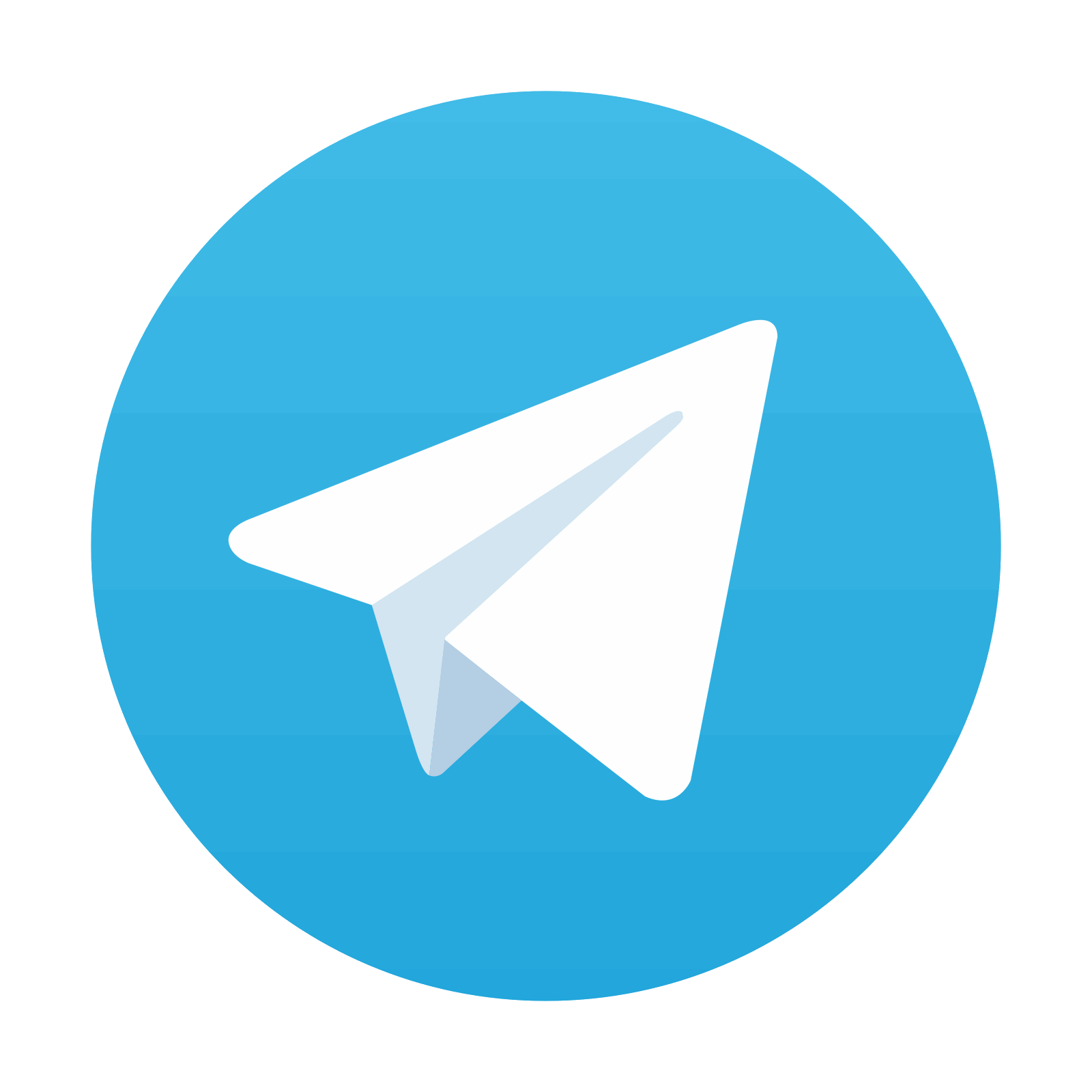
Stay updated, free articles. Join our Telegram channel

Full access? Get Clinical Tree
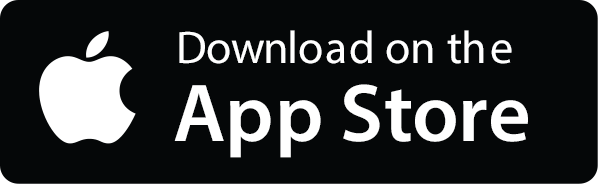
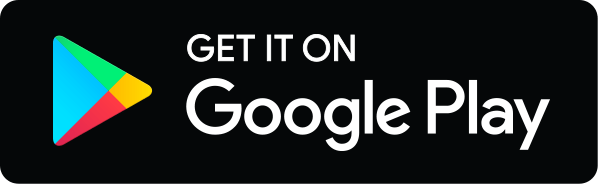