The transition from in utero fetal physiology to the immediate postnatal state involves rapid changes in cardiopulmonary function and metabolic regulation. Among the most significant events are (1) the initiation of spontaneous respiration to create a fluid–air interface for alveolar gas exchange; (2) the clearance of fetal lung fluid via the pulmonary lymphatic system; (3) the reduction in pulmonary vascular resistance and increase in pulmonary blood flow due to lung inflation and oxygen exposure; and (4) the increase in systemic vascular resistance from umbilical cord clamping, leading to closure of the foramen ovale and reduced flow across the ductus arteriosus, thus increasing systemic arterial oxygen saturations.
Resuscitation in the delivery room is unique because the primary goal is to support the process of cardiopulmonary transition. Interruption at any point can lead to critical consequences such as persistent pulmonary hypertension of the newborn, in which the pulmonary vascular resistance remains elevated and there is shunting of desaturated venous blood to the systemic circulation via the foramen ovale and ductus arteriosus. In most cases, the use of basic resuscitation measures such as stimulation, airway suctioning, and positive pressure ventilation are sufficient. Less frequently, the neonate requires tracheal intubation, chest compressions, or administration of intravascular fluids or medications.
There are multiple antenatal and intrapartum factors that increase the likelihood that neonatal resuscitation will be necessary, in addition to a number of congenital conditions that may compromise the neonate immediately after birth. Aziz et al. [1] developed a predictive model using a large database of deliveries and found that the elements listed in Table 9.1 were most highly associated with the need for positive pressure ventilation or tracheal intubation. The presence of major congenital malformations or hydrops fetalis was also significantly associated with the need for resuscitative measures.
Table 9.1 Risk factors for neonatal resuscitation
Antenatal risk factors | Intrapartum risk factors |
---|---|
Multiple pregnancy < 35 weeks | Prematurity <36 weeks |
Maternal infection | Breech presentation |
Hypertension | Meconium-stained amniotic fluid (MSAF) |
Oligohydramnios | Non-reassuring fetal heart rate |
Emergency Cesarean section | |
Shoulder dystocia | |
Scalp pH <7.1 |
Oxygen Therapy and Airway Management
Perhaps the most fundamental difference between delivery room resuscitation and resuscitation in other settings is the recommendation regarding the use of supplemental oxygen for term newborns. A landmark prospective controlled trial by Scandinavian investigators challenged the assumption that initial resuscitation of the newborn should be performed with 100 percent oxygen and, in fact, found that the use of room air for asphyxiated newborns with birthweight >1000 g decreased the time to first spontaneous breath [2]. Meta-analyses of several trials furthermore demonstrated decreased mortality for the groups resuscitated with room air [3,4]. Many areas of the world now initiate resuscitation with room air; current North American recommendations for initial resuscitation of both term and preterm infants is to use room air or a blended air/oxygen mixture titrated to achieve targeted oxygen saturations [5] (see Table 9.2). Preterm infants are more likely to require supplemental oxygen, based on studies demonstrating persistent hypoxemia in infants less than 32 weeks’ gestation when using ventilation with room air [6–8].
Table 9.2 The Neonatal Resuscitation Program’s targets for oxygen saturations in the immediate newborn period
Time after birth | Preductal SpO2 |
---|---|
1 min | 60–65 percent |
2 min | 65–70 percent |
3 min | 70–75 percent |
4 min | 75–80 percent |
5 min | 80–85 percent |
10 min | 85–95 percent |
Cyanosis is common immediately after birth as the fetal oxyhemoglobin saturation is around 60 percent. Preductal room air oxygen saturations in healthy term infants will typically reach 85–95 percent by ten minutes of life [9]. The Neonatal Resuscitation Program (American Academy of Pediatrics/American Heart Association) recommends targets for oxygen saturations in the immediate newborn period as shown in Table 9.2 [5].
If the newborn has respiratory effort but oxygen saturations fail to improve in the first few minutes of life, administer supplemental oxygen and consider CPAP. If the infant has absent of ineffective respiratory effort or becomes bradycardic, positive pressure ventilations with titrated concentrations of oxygen are indicated. For term newborns, initial inflating pressures of 30–40 cmH2O may be required to recruit lung volume and establish an air–alveolar interface. Preterm infants are more prone to barotrauma and should be ventilated with enough inflating pressure to generate chest rise, usually <20 cmH2O. In either population, ventilation pressures should be adjusted as lung compliance improves. The associated application of positive end-expiratory pressure (PEEP) in the delivery room supports the establishment of functional residual capacity (FRC) [10]. Neonates that remain cyanotic or bradycardic despite the use of effective positive pressure ventilations via facemask should undergo tracheal intubation and assisted ventilation. Laryngeal mask airways are an alternative if manual ventilations or tracheal intubation are not effective in infants ≥34 weeks’ gestation and ≥2000 g [11]. Persistent bradycardia despite a secure airway and effective ventilations is an indication for chest compressions and ventilation with 100 percent oxygen (Figure 9.1).
Figure 9.1 The Neonatal Resuscitation Program (American Academy of Pediatrics/American Heart Association) algorithm illustrates the progressive escalation of measures for delivery room resuscitation.
Emergency Vascular Access
The presence of patent umbilical vessels facilitates emergency vascular access in the delivery room. Indications for umbilical line placement include the need to administer volume expanders or medications. While several drugs can be delivered intratracheally (e.g., epinephrine), the intravenous route is always preferred. During resuscitation the placement of an umbilical venous catheter (UVC) provides rapid access to the central circulation for fluids, blood products, or medications. In the delivery room setting it is appropriate to place a “low” UVC in which the catheter is advanced only a few centimeters until blood return is achieved. Further advancement of the catheter requires X-ray confirmation of placement to ensure the line traverses the ductus venosus and is not positioned in the portal vein or a hepatic vein. If umbilical venous catheterization is not possible, alternatives include peripheral intravenous access or intraosseous infusion. Cannulation of the umbilical artery is typically reserved for neonates requiring continuous arterial blood pressure monitoring or frequent laboratory sampling in the intensive care unit. If volume expansion is indicated based on poor perfusion or a history of blood loss, isotonic crystalloid or packed red blood cells (PRBCs) can be administered in aliquots of 10 ml kg–1 followed by reassessment.
Resuscitation Medications
While it is rare to require medication administration during neonatal resuscitation, dosing accuracy is critical, especially with low birth weight infants. Other than volume expansion for suspected hypovolemia, the only other medication that is routinely used in the delivery room is epinephrine. Epinephrine is indicated when, despite effective ventilations with 100 percent oxygen and chest compressions, the newborn remains bradycardic (heart rate <60 min–1) [5]. Although epinephrine can be administered intratracheally, the intravenous route is strongly preferred. The recommended dose of intravenous epinephrine is 0.01–0.03 mg kg–1 of 1:10,000 (0.1 mg mL–1) epinephrine. The use of high-dose (0.1 mg kg–1) intravenous epinephrine is not recommended, although doses of 0.05–0.1 mg kg–1 may be given via the endotracheal tube if intravenous access cannot be established [5].
Of note, the use of naloxone for newborns with respiratory depression in the delivery room is not recommended; instead, the patient’s cardiorespiratory status should be supported with ventilation and oxygenation. Other medications such as sodium bicarbonate, glucose, or vasopressors are typically initiated once the neonate is in the intensive care unit with additional laboratory and monitoring capabilities.
Special Circumstances
Meconium-Stained Amniotic Fluid
In utero passage of meconium occurs in both normal pregnancies and those characterized by fetal distress during labor. Meconium-stained amniotic fluid (MSAF) is seen more commonly in post-date newborns and those who are small for gestational age (SGA). Meconium-stained amniotic fluid is a major risk factor for development of meconium aspiration syndrome (MAS), a potentially life-threatening respiratory disorder. Tracheal suctioning prior to initiation of respirations was widely used in the past to prevent MAS; however, management of MSAF has changed significantly over the past two decades due to findings from large studies demonstrating no benefit of tracheal suctioning for vigorous neonates [12]. Even for infants who are depressed at birth, current recommendations are to initiate the usual steps of resuscitation, including clearance of the mouth and nose, to avoid harm related to delay in ventilation [13].
Prematurity
Prematurity carries a unique set of considerations for resuscitation, including regulation of temperature, pulmonary immaturity, and risk of oxygen toxicity. Outcome for preterm infants is related to both gestational age and birth weight, and is also influenced by gender and singleton vs. multiple births. The use of antenatal corticosteroids to accelerate fetal lung development is also associated with lower mortality and morbidity [14]. The National Institute of Child Health and Human Development (NICHD) maintains a Neonatal Research Network that provides extremely preterm birth outcome data for gestational ages between 22 and 25 weeks [15].
Preterm infants are more likely to require advanced respiratory management in the delivery room due to surfactant deficiency associated with pulmonary immaturity. Surfactant, a phospholipid produced by type II pneumocytes, reduces alveolar surface tension and facilitates elastic recoil and maintenance of lung volume at end-expiration. Pulmonary surfactant production begins at 23–24 weeks’ gestation, and can be stimulated by the use of antenatal corticosteroids [14]. Surfactant deficiency results in neonatal respiratory distress syndrome (RDS) characterized by atelectasis, hypoxemia, and decreased lung compliance. The initial treatment for RDS includes supplemental oxygen and application of continuous positive airway pressure (CPAP) to maintain alveolar inflation. Despite the use of noninvasive ventilation, the premature newborn is at high risk of respiratory failure due to unfavorable pulmonary mechanics and immature control of respiration.
For infants requiring assisted ventilation, surfactant replacement therapy with synthetic or animal-derived exogenous surfactant reduces neonatal mortality and the risk of acute and chronic lung disease. Prophylactic surfactant administration (i.e., tracheal intubation and surfactant instillation prior to development of signs of RDS) is no longer used routinely with the widespread adoption of early nasal CPAP [16]. However, the use of early, selective surfactant administration for preterm infants with respiratory failure is favored over delayed administration in the setting of worsening RDS [17]. A recent multicenter study showed no difference in the composite outcome of death or bronchopulmonary dysplasia (BPD) for extremely preterm infants (24–27 weeks’ gestation) receiving nasal CPAP versus tracheal intubation and surfactant administration in the first hour of life [18].
Exogenous surfactant is administered intratracheally in a volume based on weight, using a small feeding tube to provide 2–4 aliquots while adjusting the infant’s position. During surfactant administration the neonate may experience desaturation and bradycardia that typically responds to assisted ventilation. Following surfactant administration, lung compliance may rapidly improve; close monitoring of chest excursion and exhaled tidal volumes is critical to prevent overdistention and pneumothorax, especially if the infant is to be transported.
Exposure to high concentrations of supplemental oxygen is potentially toxic to the immature lung and the developing retina. Although BPD is multifactorial, oxidative stress and inflammation from oxygen exposure are major contributors. Oxygen saturation goals for preterm infants represent a balance between avoiding oxygen toxicity and providing adequate oxygen delivery to developing organs. The SUPPORT study (Surfactant, Positive Pressure, and Oxygenation Randomized Trial) was a large randomized trial that compared two saturation targets, 85–89 percent vs. 90–95 percent, in preterm infants of less than 28 weeks’ gestational age [19]. The higher oxygen saturation target was associated with lower rates of necrotizing enterocolitis (NEC) and higher survival rates, despite an increased incidence of retinopathy of prematurity (ROP).
Based on similar findings from studies in the United Kingdom, Australia, and New Zealand, current recommendations for preterm infants after initial resuscitation are for titration of oxygen concentration to achieve saturation goals of 90–95 percent [20]. In terms of ventilation targets, hypocarbia is to be avoided as it is associated with a higher incidence of BDP and periventricular leukomalacia [21]. Likewise, hypercarbia is associated with worse outcomes when combining death with serious intraventicular hemorrhage, bronchopulmonary dysplasia, and neurodevelopmental impairment [22].
Preterm neonates are vulnerable to hypothermia due to their lack of subcutaneous fat, thin skin, and high body surface area-to-mass ratio. Deleterious effects of hypothermia include hypoglycemia, metabolic acidosis, and RDS, and hypothermia is associated with increased mortality [23]. The neutral thermal environment (ambient temperature at which heat is neither gained or lost) depends on the gestational age and weight. Extremely low birth weight (<1000 g) or very low birth weight (<1500 g) neonates require active warming in addition to measures to prevent heat loss, such as head covering and occlusive wrap to the skin. The use of a servo-controlled radiant warmer is preferred to a conventional incubator immediately after birth [24]. Other measures to combat hypothermia include increasing the room temperature, use of an insulated transwarmer mattress, and warming inspired gases [25,26].
Congenital Anomalies
Advances in prenatal imaging and genetic testing have afforded the opportunity to anticipate resuscitation needs for neonates with specific prenatally diagnosed conditions. The fetus with congenital diaphragmatic hernia (CDH), for example, is often referred for delivery in a perinatal center with the capability of extracorporeal membrane oxygenation (ECMO), but the initial steps of resuscitation are adjusted due to the anatomic considerations. Newborns with a prenatal diagnosis of CDH should undergo prompt tracheal intubation, avoiding the use of positive-pressure ventilation by mask to prevent gaseous distention of the stomach and intestines. Immediately following tracheal intubation, placement of a sump-type naso- or orogastric tube is essential to remove any swallowed air using continuous suction. Since the stomach and/or intestines may be in an intrathoracic location, failure to decompress gastric air can lead to further cardiopulmonary compromise. As the presence of CDH is associated with significant pulmonary hypoplasia and pulmonary hypertension, these patients may remain desaturated, with evidence of ductal-level right-to-left shunting based on pre- and postductal oxygen saturation differences. It is important to recognize that the ipsilateral lung in CDH patients is also abnormal, and a high rate, low tidal volume mechanical ventilation strategy is recommended. In the immediate post-delivery period, ventilate with 100 percent oxygen to achieve a preductal oxygen saturation goal of >85 percent using a peak inspiratory pressure <25 cmH2O while transferring to the intensive care unit.
The EXIT procedure (ex utero intrapartum therapy) has been attempted for infants with severe pulmonary hypoplasia who are unlikely to survive without immediate use of ECMO. In the EXIT to ECMO approach the infant is partially delivered via C-section and cannulation of the neck vessels is performed while maintaining uteroplacental circulation. Although results are limited to retrospective reviews, the use of peripartum ECMO has not been shown to improve outcome for this specific patient population [27]. In other conditions the EXIT procedure has been used successfully to allow for immediate surgical intervention for critical high airway obstruction syndrome (CHAOS) or life-threatening neck or lung masses [28].
Hydrops Fetalis
Hydrops fetalis is a life-threatening complication for the fetus characterized by diffuse body wall edema and effusions in pleural, pericardial, and peritoneal spaces.
The etiologies of hydrops fetalis are diverse and include “non-immune” causes such as chromosomal abnormalities, congenital infections, congenital heart disease, and in utero cardiac rhythm disturbances (complete heart block, supraventricular tachycardia). Immune causes such as hemolytic diseases are less common with the use of maternal therapy to prevent Rh-isoimmunization in mothers with Rh-negative blood types.
Hydrops fetalis has a high rate of premature birth and postnatal morbidity and mortality, depending on the underlying cause. Delivery room resuscitation may be complicated by poor lung and chest wall compliance, requiring urgent pleural drainage to facilitate ventilation. In the setting of longstanding fetal hydrothorax pulmonary hypoplasia may be significant and there is high risk of pneumothorax during attempted resuscitation.
Post-Resuscitation Care
The most significant recent advance in neonatal resuscitation has been the use of therapeutic hypothermia to improve outcome for newborns with hypoxic-ischemic encephalopathy related to perinatal asphyxia. The use of moderate hypothermia (core temperature 33.5–34.5 °C) for depressed newborns >36 weeks reduces mortality and neurodevelopmental disability as found in several randomized clinical trials [29–31]. Clinical criteria for induced hypothermia typically include a combination of Apgar scores, umbilical cord or patient pH or base deficit, duration of resuscitation, and exam consistent with moderate or severe encephalopathy. Some centers also include the results of amplitude-integrated encephalography (aEEG). Hypothermia is typically provided using a similar approach to the published clinical trials, with initiation within six hours of age and continuation for 72 hours, followed by gradual return to normothermia.
Therapeutic hypothermia is typically provided in tertiary care centers with neonatal expertise. Timely identification of eligible newborns delivered in the community is essential due to the time-sensitive nature of initiating therapy. Passive cooling with careful temperature monitoring may be recommended prior to transfer, with caution to avoid excessive hypothermia [32]. Alternatively, a servo-controlled device can be used to provide controlled active cooling [33].
References

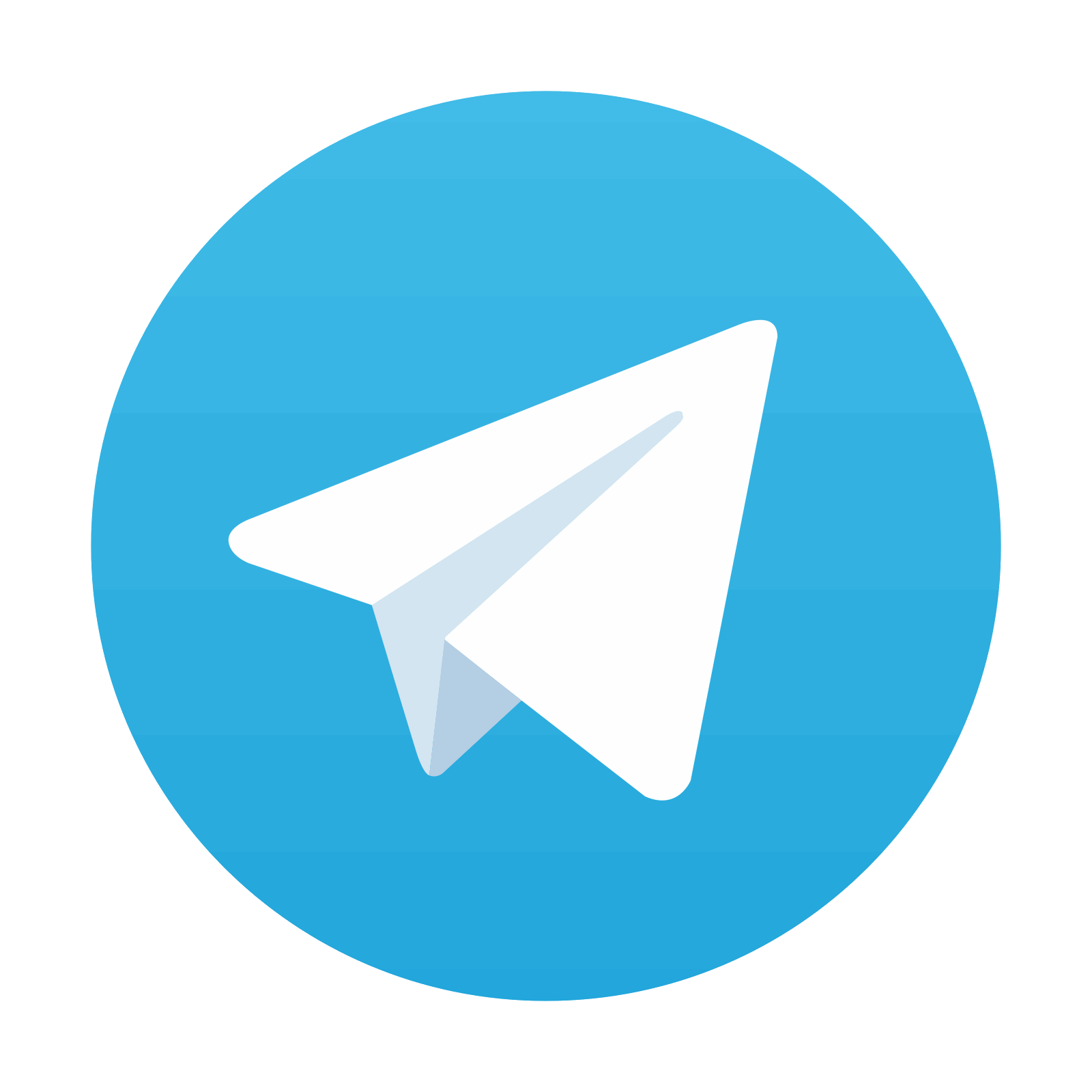
Stay updated, free articles. Join our Telegram channel

Full access? Get Clinical Tree
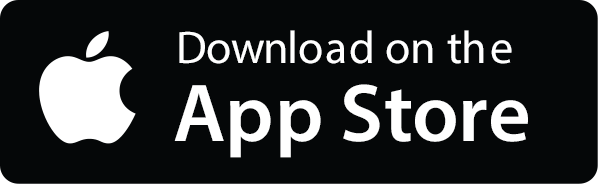
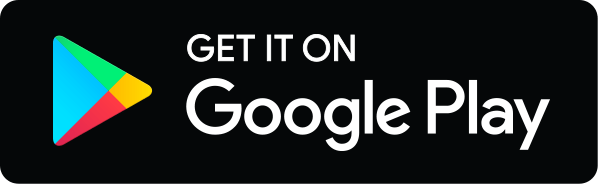
