Introduction
Fetal intervention is a fascinating, unique, and rapidly developing field of medicine that involves the expertise and teamwork of many subspecialties including surgery, anesthesia, obstetrics, radiology, and neonatology. It is unique in that one is asked to provide care to two patients – the maternal patient who can communicate her needs and discomforts, is easily monitored, and can readily have medications administered, and the frequently “hidden” fetal patient, whom one can only get intermittent glimpses of through advanced imaging techniques. In the fetus, one can only assume or infer nociceptive capabilities, and monitoring is intermittent and often unreliable. Drug administration is usually difficult and many times indirect.
The first successful fetal intervention was performed by Sir William Liley in 1963, and involved blind, intraperitoneal blood transfusion to a fetus affected with erythroblastosis fetalis. It was not until almost 20 years later that Harrison and his group at the University of California at San Francisco reported the first open fetal surgical intervention in a 21-week-old fetus with severe obstructive hydronephrosis. Through bilateral ureterostomies, the urinary tract of the fetus was decompressed and the fetus was able to continue to grow and develop for three months prior to delivery. Unfortunately, because of extensive renal and pulmonary damage, the infant died within a day of delivery [1]. Despite this initial disappointment, a group of pioneers in the field of fetal surgery joined with other subspecialists to study the pathophysiology and natural course of many fetal anomalies and diseases. The goal was to both arrest the progression of the disease and allow definitive postnatal repair, or to correct the disease outright. A consensus document was drafted in 1982 by the newly created International Fetal Medicine and Surgery Society that is still referenced today (Table 34.1) [2]. A period of enormous research followed with randomized multicenter trials involving human patients, which established the role of in utero surgery as a clinical reality designed to help once-doomed fetuses. It is estimated that approximately 1000 fetal surgeries were performed in 2012 in the United States alone, and this number is predicted to rise substantially in the near future [3]. Another evolution in fetal medicine is underway, with many current areas of research focusing on stem cell and gene therapy [4]. Promising candidates for human application include those fetuses affected with hemophilia, muscular dystrophy, and central nervous system disorders. Although great progress has been made over the last 40 years, much remains to be discovered and accomplished.
Table 34.1 Criteria for Fetal Surgery
1. | Accurate diagnosis and staging of the disease with noted exclusion of other associated anomalies. |
2. | The natural history of the disease is documented and the prognosis is established. |
3. | In utero surgery proven feasible in animal models, reversing deleterious effects of the conditions. |
4. | There is no current effective postnatal treatment. |
5. | Intervention can only be ethically justified if there is a reasonable probability of benefit. |
6. | The family is counseled about risks and benefits and should agree to treatment including long-term follow-up to determine efficacy. |
7. | Interventions should be performed at specialized multidisciplinary fetal treatment centers after approval by an institutional review board. |
8. | All case material should be reported, regardless of outcome, to a fetal-treatment registry and/or in the medical literature, so that the benefits and liabilities of fetal therapy can continue to be established |
The Maternal Patient
Implicit in the criteria for fetal surgery is the understanding that one must “Do No Harm.” The mother has been referred to as an “innocent bystander” who, although exposed to significant surgical and postpartum risk, receives no direct health benefits. It is essential that the anesthesiologist taking care of the fetus has an awareness that whatever hemodynamic changes affect the mother also affect the fetus. Although virtually every organ system in the mother undergoes physiological changes during pregnancy, it is most important for the fetal anesthesiologist to focus on the maternal cardiovascular, respiratory, and nervous system changes of pregnancy and the anesthetic implications in the perioperative period.
Cardiovascular
Cardiac output will increase by as much as 35–40 percent by the end of the first trimester [5]. It will continue to rise throughout the second trimester until it reaches a level that is 50 percent greater than nonpregnant women. The majority of the increase in cardiac output in early pregnancy is due to increases in heart rate of 15–25 percent above prepregnancy levels that then remain stable after the second trimester [6]. Stroke volume will progressively increase by 25–30 percent by the end of the second trimester and remain this way until term [7].
The gravid uterus may decrease preload, cardiac output, and maternal blood pressure by compressing the aorta and/or vena cava of supine women. This becomes most pronounced at term. Although the majority of women will experience minimal if any hypotension when supine due to increased systemic vascular resistance, any anesthetic that decreases sympathetic tone (such as neuraxial blockade or some general anesthetics) will worsen the effects of aortocaval compression, resulting in significant hypotension. Maternal hypotension will decrease uteroplacental perfusion and impair fetal oxygenation. For these reasons, the more traditional left uterine displacement or in some cases right uterine displacement must be provided.
For many open fetal interventions, high concentrations of volatile anesthetics may be necessary for adequate uterine relaxation to prevent preterm labor and inadvertent placental separation. These agents are potent vasodilators and at higher doses are cardiac depressants. Therefore prompt treatment of hypotension is very important. Because uteroplacental blood flow is not autoregulated, falls in maternal blood pressure will result in decreased blood flow to the fetus with resultant hypoxia and acidosis. Intravenous ephedrine and/or phenylephrine are drugs of choice used in most cases to treat maternal hypotension. Although fluid boluses may also help in improving preload and maternal blood pressure, careful attention must be paid to the fluid status of the mother. Aggressive fluid resuscitation combined with the abundant use of prophylactic tocolytics has been implicated in occurrences of pulmonary edema in some fetal surgery patients [8].
Pulmonary
Pregnancy results in progressive increases in oxygen consumption and minute ventilation along with a reduced functional residual capacity and residual volume [9]. These increases in metabolic demand make the pregnant patient more prone to hypoxia during periods of apnea or hypoventilation. Prior to anesthetic induction, it is imperative to adequately preoxygenate the mother and immediately intubate following a rapid sequence induction. Maternal hypoxia will obviously result in fetal hypoxia.
Resting maternal PaCO2 decreases from 40 mmHg to approximately 30 mmHg by 12 weeks’ gestation and remains at this level until term [9]. Ventilating the pregnant patient demands meticulous attention. Excessive hyperventilation below a PaCO2 of 20 mmHg will likely lead to uterine vessel vasoconstriction and decreased placental perfusion, jeopardizing the fetus [10].
Nervous System
Pregnancy will decrease the minimal alveolar concentration (MAC) of volatile anesthetics by approximately 30 percent [11]. While the concentration of inhaled agent required to achieve a desired anesthetic effect is decreased, one must remember that high concentrations are often required for many open fetal procedures. Local anesthetic dose requirements for spinal and epidural anesthesia are also lower than for the nonpregnant patient and hence adequate doses are usually about one-third less than those of nonpregnant women.
The Fetal Patient
The combination of immature organ systems and the stress of a surgical intervention place the fetus at a considerable anesthetic risk. The well-being of the fetus is dependent on both maternal oxygenation as well as the adequacy of fetoplacental perfusion.
Fetal Oxygenation
The supply of oxygen to the fetus depends on a number of factors. First, the mother must be adequately oxygenated. Second, there must be sufficient flow of well-oxygenated blood to the uteroplacental circulation. As discussed in the previous section, maternal hypotension can result from aortocaval compression as well as the direct cardiac depressant effects of the inhaled anesthetics or the vasodilatory effects of local anesthetics. Significant maternal hemorrhage reduces maternal blood flow and thus uterine blood flow. It is also known that the surgical incision of hysterotomy that is required for open fetal procedures reduces uteroplacental blood flow by as much as 73 percent in the sheep model, whereas fetoscopic procedures have no effect [12]. Furthermore, anything that increases maternal catecholamine production (i.e., light anesthesia) will increase uteroplacental vascular resistance and decrease flow.
Tocolytic agents are often required to prevent preterm labor in fetal surgery patients. Uterine contractions are particularly dangerous during a fetal intervention, not only because uterine blood flow is reduced, but also because inadvertent placental separation can occur, leading to disastrous outcomes.
Even if the uterine circulation is adequate, the fetus will still rely on blood flow to the placenta and the umbilical vein for tissue oxygenation. Increased amniotic fluid volume will increase intra-amniotic pressure and will impair uteroplacental perfusion [13,14]. Studies in animals suggest that uteroplacental perfusion has to be reduced by >50 percent before there are adverse effects on fetal gas exchange [15]. In the setting of increased fetal catecholamine production (i.e., fetal stress), fetoplacental vascular resistance will rise, which will in turn increase fetal cardiac afterload [16]. Other factors that can impair umbilical blood flow and hence fetal oxygenation include umbilical cord kinking, umbilical vessel spasm, or significant fetal hemorrhage. Rapid, uncontrolled loss of amniotic fluid may result in kinking of the umbilical vessels, or even direct compression of the umbilical vessels by a fetus that is no longer suspended in the amniotic fluid.
Fetal Cardiovascular Response
Unlike adults and older children, fetal cardiac output depends more on heart rate than on stroke volume [17]. Because fetal myocardial contractility is likely maximally stimulated, the fetus has a limited ability to increase stroke volume. In animal studies, increases in preload had a limited effect on cardiac output – volume loading increased cardiac output by only 15–20 percent [18].
The choice of a maternal anesthetic will impact the fetus. All inhaled anesthetics rapidly cross the placental barrier [19], yet the uptake occurs more slowly in the fetus than in the mother. The partial pressures of volatile anesthetics are also lower in the fetal umbilical vessels than in maternal arterial blood [20]. However, MAC in the fetus is below that of the mother [21]. During moderately deep (1.5 MAC) isoflurane or halothane anesthesia, maternal arterial pressure and cardiac output were decreased. Uterine vasodilation occurred and uteroplacental perfusion was maintained [22]. Fetal oxygenation and base excess were also maintained. However, at higher concentrations of inhaled anesthetic (2.0 MAC), maternal hypotension resulted in reduced uteroplacental perfusion despite uterine vasodilation leading to fetal hypoxia and acidosis [22,23]. The administration of 1.5–2.0 MAC of anesthesia should result in fetal anesthesia as long as uteroplacental gas exchange is maintained.
Fetal Pain
Because pain is a subjective experience, some may argue whether the fetus even feel pain and, if it does, when does it feel pain? It has been suggested that to feel pain one needs to be aware, or conscious, and consciousness cannot be demonstrated in the fetus. The fetus cannot tell us what it is feeling, and there is no objective method available to measure pain. Given current knowledge, one must rely on anatomic evidence and the fetal stress response to help guide the anesthetic management of the fetus during surgery.
It is known that cutaneous receptors are present in the human fetus by the 20th week of gestation. However, the fetal awareness of noxious stimuli requires functional thalamocortical connections which appear at 23–30 weeks’ gestation [24]. Electroencephalogram patterns are discontinuous before 25 weeks. From 25 to 29 weeks, periods of activity increase, such that by 30 weeks, electroencephalogram patterns show a distinction between wakefulness and sleep [25]. At this point in development, it seems certain that the fetus has the necessary neuroanatomic connections to feel pain. In fact, the descending inhibitory pathways are not complete until after birth so conceivably the fetus may be even more sensitive to painful stimuli than the older child.
Activation of the hypothalamic–pituitary–adrenal axis (i.e., stress response) may also be an indicator of pain. Human fetal endocrine responses to stress have been demonstrated as early as 20 weeks’ gestation. Giannakoulopoulos and colleagues demonstrated a fetal endocrine stress response after obtaining blood samples from fetuses at 20–34 weeks’ gestation with intrauterine needling [26]. Needling of the innervated intrahepatic vein (IHV) resulted in increases in fetal plasma concentrations of cortisol and beta-endorphin (known stress hormones), whereas needling of the denervated placental cord failed to showed these pronounced hormonal responses. Fetal plasma levels of noradrenaline were also significantly elevated in those fetuses (18–37 weeks’ gestation) who had IHV sampling when compared with those fetuses who had umbilical venous blood sampling from the placental cord [27]. Convincing evidence for the importance of providing analgesia to the fetus came from the landmark study by Fisk and colleagues in which intravenous fentanyl (10 μg kg–1 estimated fetal weight × 1.25 placental correction) ablated the beta-endorphin response in fetuses having IHV cannulation [28]. Further support for providing analgesia to fetuses undergoing invasive procedures comes from primate studies indicating that periods of perinatal stress during critical times of development can have permanent detrimental effects on hippocampal development and stress behavior [29–31]. A final reason for providing anesthesia and analgesia to the fetal patient is a humanitarian and ethical one. As Glover and Fisk so eloquently stated in a 1996 editorial, “We don’t know better; better to err on the safe side from mid-gestation” [32].
Diseases and Interventions
Cardiac
Pharmacological fetal cardiac interventions have been attempted when the fetus is at risk for demise or severe morbidity due to arrhythmia or fetal heart block. Maternal oral administration of digoxin, propranolol, and more specific antiarrhythmics are part of the treatment for fetal arrhythmias and high-grade AV block. When transplacental passage is compromised as in the setting of hydrops, a more direct administration can be achieved with intramuscular or intravenous injection to the fetus, under ultrasound guidance [33].
Anatomic cardiac defects are the most common congenital newborn malformation and they can be diagnosed prenatally as early as 14 weeks’ gestation. The advances in fetal imaging techniques allow for a better understanding of the natural history and progression of these lesions. Clinicians struggle to improve outcome in instances when the survival of the fetus is at stake or when significant neonatal morbidity is anticipated. Fetal interventions for congenital cardiac malformations have seen a tremendous growth and are now therapeutic options for specific conditions. By and large, minimally invasive, percutaneous, and image-guided fetal cardiac interventions are typically performed at mid or late gestation. A team that includes an experienced ultrasonographer, obstetrician, interventional cardiologist, maternal anesthesiologist, and fetal anesthesiologist work together in the operating room. The immediate risks to the fetus are mitigated by the goals of improving survival and morbidity. Appropriate follow-up will help define long-term risks and benefits of these techniques. Before attempting difficult and high-risk procedures, it is imperative to define their anticipated goals (Table 34.2). Currently three cardiac conditions fit criteria for prenatal intervention and each has specific objectives (Table 34.3).
Table 34.2 Fetal cardiac interventions
Goals |
---|
Prevent fetal death |
Prevent arrhythmias |
Normalize hemodynamics |
Normalize pulmonary development |
Lesions |
Aortic stenosis |
Pulmonary stenosis or atresia with intact ventricular septum |
Hypoplastic left heart syndrome with intact or restrictive atrial septum |
Table 34.3 Goals of specific fetal cardiac interventions
Aortic valvuloplasty | Pulmonary valvuloplasty | Atrial septostomy |
---|---|---|
Decrease LV afterload | Increase RV growth | Decompress LA |
Promote flow through left heart | Improve pulmonary vasculature | Prevent pulmonary venous hypertension |
Prevent HLHS | Reverse hydrops | Prevent postnatal hypoxia |
Obstructive cardiac valve lesions progress during pregnancy and according to the flow theory, normal blood flow through the heart contributes to normal growth of the ventricles. Ultimately the decision to intervene is guided by the objective of avoiding single ventricle physiology in the postnatal period. Despite an increased survival in specialized centers with expertise managing single ventricle patients, the long-term complications after palliative surgery remain quite debilitating. Two-ventricle circulation is preferred as it is a superior predictor of improved life expectancy and quality of life [34].
Fetal Aortic Valvuloplasty
Rationale for Intervention
Echocardiographic predictors of the evolution of critical aortic stenosis (AS) to hypoplastic left heart syndrome include (1) valvar aortic stenosis with dilated or normal size left ventricle; (2) reversed flow in the transverse aortic arch and foramen ovale; and (3) monophasic mitral inflow [35]. With critical AS and impaired left ventricular growth the left ventricle becomes hypoplastic. Endocardial fibroelastosis (EFE) contributes to impaired filling and redirection of flow across the foramen ovale to the right ventricle, now in charge of the whole cardiac output. A different picture evolves when the increase in left ventricle pressure results in rapid left ventricle dilation and mitral regurgitation. The absence of synchronous left ventricle contractions combined with EFE and impaired coronary perfusion cause progressive and irreversible myocardial damage. Extreme left ventricle dysfunction and dilation with severe mitral regurgitation and increased left atrial pressure can cause closure of the foramen ovale, thus rendering the left atrium unable to decompress into the right atrium. The pulmonary veins are dilated and ultimately congestive heart failure (CHF) or hydrops occur when the right ventricle is unable to maintain cardiac output.
The technical steps involve the advancement of a needle through the maternal abdomen into the amniotic sac under continuous ultrasound guidance. The left ventricle is reached through the fetal chest. After alignment of the insertion needle with the left ventricle outflow tract, a coronary balloon catheter is introduced through the needle and inflated across the obstructed valve. A technically successful procedure results in flow across the valve accompanied with moderate to severe aortic regurgitation and decrease in left ventricular size.
Results
The group at Boston Children’s Hospital performed their first fetal aortic valvuloplasty in 2000. After the obligatory learning curve, the Boston group now reports an 80 percent technical success rate [36]. In their most recent review they report that 30 percent of fetuses after a technically successful procedure are born with biventricular circulation, while 8 percent are converted to two-ventricle physiology after initial univentricular palliation. Half of the fetuses experienced hemodynamic instability during the procedure, namely bradycardia requiring treatment with intramuscular or intracardiac injections of epinephrine and bicarbonate and drainage of pericardial effusions. There were no maternal complications, but there was a 10 percent rate of fetal death or premature delivery.
The Austrian group published the second largest series with 24 procedures and a 67 percent technical success rate [37]. Of these, 63 percent achieved biventricular physiology after birth. It seems reasonable to eliminate candidates for fetal valvuloplasty when there is high velocity forward flow in the aortic arch and good ventricular function, as these patients can wait for postnatal treatment. Fetuses with ventricles that are too small to avoid univentricular circulations should not be candidates for the procedure.
Fetal Pulmonary Valvuloplasty
Rationale for Intervention
In contrast to the left ventricle, after interruption or limitation of blood flow from pulmonary valve obstruction, the right ventricle does not dilate but develops excessive muscular hypertrophy. Impaired filling and growth can result in CHF or univentricular circulation at birth. Ten percent of pulmonary valve stenosis cases progress to pulmonary atresia. Patient selection is critical since the postnatal outcome for the majority of cases of pulmonary atresia with intact atrial septum is favorable with postnatal balloon valvuloplasty. Fetal tricuspid valve size has emerged as a possible predictor of postnatal outcome. Fetuses with hypoplasia of the tricuspid valve annulus and a significant transvalvular pressure gradient are the best candidates. The optimal timing to intervene would be before right ventricular hypoplasia and coronary fistula develop. The fetal right ventricular outflow tract is accessed percutaneously with a balloon catheter through a subcostal or intercostal approach.
Results
With their series of 30 fetuses undergoing mid-gestation pulmonary valvuloplasty, Tworetzky et al. report technical success for 75 percent of cases but no decisive evidence that the intervention changed the natural course of the disease [38].The risks for the fetus remain the same as for aortic valvuloplasty, but hemopericardium is more likely due to a larger needle and bigger balloon.
Fetal Atrial Septostomy
Rationale for Intervention
In fetuses with left-sided obstructions, an open foramen ovale is crucial for drainage of the pulmonary venous return. Increased left atrial pressure resulting in premature closing of the foramen ovale will generate high pressure in the pulmonary vascular bed resulting in increased morbidity and mortality at birth despite urgent atrial septostomy. Chronic pulmonary venous hypertension in utero has been shown to reduce the survival rate of Norwood stage 1 from 90 percent to 52 percent [39].
Results
The largest series to date, from the Boston experience, contains 21 procedures performed between 24 and 34 weeks’ gestation [40]. The creation of a defect of at least 3 mm in diameter is associated with better oxygenation and less frequent need for emergent postnatal intervention, but the impact on survival has not been demonstrated. Two fetal deaths were directly attributed to the intervention. To prevent the risk of re-stenosis after a single dilation, the authors have questioned the benefit of stenting rather than catheter and balloon inflation. In the future, short bursts of YAG laser directed at the atrial septum could achieve longer lasting results.
Airway
Extrinsic Obstruction
Lymphangiomas and teratomas around the airway do not often cause fetal demise, but this extrinsic airway obstruction will very likely result in mortality in the perinatal period. These fetuses must be delivered via ex utero intrapartum therapy (EXIT) so the airway can be secured in a controlled fashion. The EXIT procedure will give the team time to work on the fetus while the placenta still serves as the organ of fetal respiration. Direct laryngoscopy, rigid bronchoscopy, tracheostomy, neck dissection with retrograde intubation, or even tumor resection may be accomplished during an EXIT procedure. After the fetal procedure is complete, the umbilical cord is clamped and the child is delivered to a neonatal team for further resuscitation. Ideally, the EXIT will occur at or near term, but this may not always be feasible for a variety of reasons, such as preterm labor, a shortening cervix, or very rarely, worsening hydrops and impending fetal demise.
In a series of patients with a broad range of pathology cared for between 1996 to 2002, long-term survival was 89 percent [41]. Specific pathology and pathophysiology will influence survival. Giant cervical teratoma was the diagnosis in a series of 17 EXIT procedures performed from 1995 to 2010. While all patients survived the procedure, prematurity and mass effect from the giant teratomas led to pulmonary hypoplasia which resulted in a neonatal mortality rate of 23 percent [42]. Fetuses with oropharyngeal tumors seem to fare better. All four patients with oropharyngeal tumors delivered between 2006 and 2012 survived [43].
Intrinsic Obstruction
Atresia, webs, cysts, or agenesis at the laryngeal or tracheal level may cause intrinsic obstruction of the fetal airways. This condition is commonly called CHAOS (congenital high airway obstruction syndrome) and is associated with a set of findings on prenatal imaging which includes the following: large dilated lungs, flattened or everted diaphragms, distal airway dilation, and hydrops fetalis [44]. Mortality in cases of CHAOS occurs for two reasons, and the fetus must make it past these two hurdles to survive. The first hurdle occurs during gestation. Death is due to massively enlarged lungs compressing the fetal heart. This compression of the heart by the lungs will result in a physiology similar to cardiac tamponade. Fetal hydrops and death may result. The second hurdle occurs at birth. Simple airway obstruction is the cause of demise.
In the fetal period, decompression of the fetal airway and lungs is necessary to relieve pressure on the fetal heart, and this may allow resolution of the hydrops. Decompression may occur spontaneously [45]. Fetoscopic laser decompression of the airways has been performed in selected cases [46]. A fetus with CHAOS palliated by airway decompression has made it past the first hurdle, and may survive longer in utero, hopefully delivering closer to term. At this time the fetus must clear the second hurdle and be delivered via EXIT procedure to secure the airway. Long-term survival is possible [45,47].
Myelomeningocele
Rationale
Myelomeningocele does not threaten the life of the fetus, and it is not a direct cause of neonatal mortality, but it is a cause of serious morbidity, such as paralysis, bowel and bladder dysfunction, Arnold–Chiari II malformation, and hydrocephalus. Repair of the myelomeningocele in utero would hopefully improve neurologic function by decreasing the exposure of the developing spinal cord to the amniotic fluid and by helping improve the hindbrain herniation that occurs with the Chiari II malformation.
Results
A randomized trial of prenatal versus postnatal repair of myelomeningocele was conducted between 2003 and 2010 [48]. The trial was stopped after an interim analysis as it demonstrated efficacy of prenatal surgery. The trial found a difference between the groups for the composite primary outcome of neonatal death or need for placement of a ventriculoperitoneal shunt (68 percent in the prenatal surgery group vs. 98 percent in the postnatal repair group). Another outcome was a composite score of mental development and motor function. This composite score was improved in the prenatal repair group. The procedure is not without risk to the fetus. In the prenatal surgical group, bradycardia occurred in 10 percent, death occurred in 3 percent, and mean gestational age at delivery was 34 weeks. While maternal morbidity was not a primary outcome studied in this trial, this large series of mothers undergoing open fetal surgery allowed some quantification of the risk to the mothers undergoing mid-gestation surgery and subsequent Cesarean delivery. Chorioamniotic membrane separation occurred in 26 percent of mothers undergoing the prenatal repair, while 21 percent developed oligohydramnios. Only 64 percent of the mothers were found to have a well-healed, intact uterus at the time of delivery, and 9 percent had to be transfused at the time of delivery. The Cesarean section that must be performed after open fetal surgery is more complex, as the omental flap must be taken down, and the prior hysterotomy must also be inspected and repaired if needed.
Thoracic Tumors
Two common thoracic tumors encountered in the fetal period are congenital cystic adenomatoid malformation (CCAM) and bronchopulmonary sequestration (BPS). The CCAM results from overgrowth of terminal bronchioles, so there is a connection between the tumor and the tracheobronchial tree. The CCAM may be primarily cystic, solid, or mixed. A BPS does not originate from lung tissue, and there is no connection to the fetal airways, but the BPS does have a connection to the systemic vascular supply, typically via one or several arterial feeding vessels. Occasionally a “hybrid lesion” develops, which has characteristics of both CCAM and BPS in that connections to both the airways and the systemic vasculature exist. In utero, tumors may cause cardiac compression, and may also grow enough to impede cardiac filling and function. This will result in hydrops [49]. Postnatally, neonates are at risk for respiratory compromise as these tumors do not participate in gas exchange. Additionally, mass effect from the tumor causes hypoplasia of the normal lung tissue.
The size, growth rate, pathology, and gestational age at which symptoms appear will dictate the therapy for the fetus. In most cases small tumors do not perturb the development of the fetus, and the child may be delivered normally and go home with the family after a period of observation. The child may then return at several weeks to months of age for elective resection of the tumor. Larger, growing tumors may cause symptoms of hydrops, and frequent prenatal observation is warranted. If steroids are given to the mother, the growth may slow or tumors may shrink [50]. If symptoms persist and occur before lung maturity, fetal surgical intervention is warranted. Intervention may be as minimally invasive as aspirating and decompressing a cystic lesion, or may be as complex as performing a mid-gestation hysterotomy and fetal thoracotomy with pulmonary lobectomy for solid lesions not amenable to decompression.
In some cases, if the symptoms of hydrops do not develop until later in gestation, or if the size and mass effect of the tumor are deemed too great, delivery may be via an EXIT procedure with neonatal pulmonary lobectomy before the umbilical cord is clamped [51,52]. Survival in a series of nine patients undergoing EXIT for lung resection was 89 percent [52]. The neurodevelopmental outcome of the majority of children undergoing these procedures is age-appropriate, with 77 percent scoring in the normal range for neuromotor outcome and 23 percent in the mildly delayed range [53].
Congenital Diaphragmatic Hernia
As pulmonary hypoplasia and pulmonary hypertension are major factors in the mortality of children with congenital diaphragmatic hernia (CDH), the rationale for fetal therapy is clear. If the herniated bowel could be returned to the abdominal cavity during fetal life, the lungs would have more time to grow. Open fetal procedures involving fetal laparotomy were not successful, so less invasive strategies involving fetal tracheal occlusion were developed [54]. The goal of tracheal occlusion was to stimulate lung growth, as in cases of CHAOS. Unfortunately, after much work in this field, trials of minimally invasive fetal surgical intervention with tracheal occlusion have not been shown to be better than optimal postnatal care [55]. The approach consists of tracheal fetal occlusion and subsequent delivery via EXIT procedure. This approach is still a major undertaking in terms of risk and potential morbidity for the mother. European centers are studying an even less invasive approach that involves percutaneous, fetoscopically guided placement of a balloon to occlude the fetal trachea. This balloon is removed several weeks later, also minimally invasively. This approach limits maternal risk and morbidity [56,57]. Some centers in the United States may be participating in trials in the future utilizing this less invasive strategy.
Sacrococcygeal Teratoma
Rationale
A prenatal diagnosis of sacrococcygeal teratoma (SCT) carries a high mortality rate. Death can be due to high-output heart failure from the large, rapidly growing SCT or simply from tumor rupture and fetal anemia. Symptomatic tumors will result in fetal hydrops, and a variety of ultrasound findings can be used to assess the gravity of the situation. Serial fetal echocardiography will allow for trending of fetal heart function and measurement of the combined cardiac output. Evidence of an enlarging placenta, increasing diameter of the fetal inferior vena cava, and increased blood flow to the descending aorta are all signs that the fetal status is worsening [58,59]. Palliative fetal treatment is aimed at decreasing the tumor size, whether by open surgical debulking, or minimally invasive ablation of the tumor. If the tumor burden can be decreased, the high-output failure will hopefully resolve, allowing the fetus to survive to delivery and definitive surgical resection in the postnatal period.
Results
Fetal treatment for these tumors is risky and the path is not as clear as that for lung tumors. Minimally invasive therapy has been attempted, but this is not yet an optimal technique. Approaches have included laser ablation, radiofrequency ablation, sclerotherapy, electrocautery, and vascular coiling. Survival rate after minimally invasive therapy is 30 percent. Radiofrequency ablation has caused iatrogenic trauma to the surrounding area, including the ischium, gluteal region, the femoral head, and sciatic nerve. Open fetal surgery has a survival rate of 50 percent, but is a significant undertaking, with all the attendant risks to both the mother and the fetus [60]. Open surgery involves a hysterotomy, fetal vascular access, and debulking of the tumor. The anatomy of the tumor must be considered when planning open fetal surgery. The tumors should be primarily external with a small stalk. Broad-based tumors would pose technical challenges given the time constraints of open fetal surgery. Early Cesarean delivery with early or immediate postnatal surgery in an adjacent operating room has also been proposed as a management approach [61,62].
Complicated Multiple Gestations
Rationale
Twin and higher order multiple gestations are at risk for complications. Monozygotic twins are at higher risk than dizygotic pregnancies, as a monozygotic twin pair may develop into a monochorionic–diamniotic or monochorionic–monoamniotic pregnancy. The monochorionic twins may share blood flow from the placenta to varying degrees, and in some cases this blood flow is uneven, with one twin getting more net blood flow than the other. The recipient twin can develop hypervolemia, polyuria, and polyhydramnios. The donor twin develops hypovolemia, oliguria, and oligohydramnios. Both twins are at risk for neurologic and cardiac complications, and death [63]. The goal is to save both twins, and treatment has ranged from aspiration of amniotic fluid from the twin with polyhydramnios, to creation of a microseptostomy between the twins’ amniotic membranes, to laser ablation of the vessels that are allowing unequal blood flow. In some cases, selective feticide via ablation of the umbilical cord to one of the twins is performed to try to optimize the chances for survival of one of the twins. Aspiration of excess amniotic fluid (amnioreduction) was intended as a symptomatic treatment to prevent preterm labor, but the fetuses may be helped by a reduction of uterine wall tension, and improved uteroplacental blood flow, as evidenced by improved Doppler waveforms. Microseptostomy, similarly, may equalize pressures between the two amniotic sacs to allow better uteroplacental blood flow. Laser ablation of the vessels on the placenta allowing the unequal blood flow is more directly targeted to the pathophysiology, but also carries more risk than either amnioreduction or microseptostomy. Selective feticide may decrease risk of neurologic injury or death to the surviving twin [64].
Results
A 2014 update of a Cochrane review remains supportive of the use of endoscopic laser ablation of the placental anastomoses to improve neurodevelopmental outcomes. This review included three studies and synthesized data for 253 women. When septostomy was compared with amnioreduction, no significant differences were found [65]. When taken together, the two trials comparing amnioreduction and endoscopic laser surgery showed no difference in overall death, but did show improved neurologic outcome [66,67]. The techniques continue to be adjusted, and a recent trial supports ablation of all vessels at the vascular “equator” between both twins as compared to selective ablation [68].
Anesthetic Management
To provide adequate maternal and fetal surgical conditions, optimal positioning, and stable hemodynamics, we work with a dedicated team knowledgeable of the challenges and differences of each procedure.
Anesthesia for Fetal Cardiac Interventions
Maternal Anesthesia
Regardless of the indications, all fetal cardiac interventions (FCIs) are currently performed percutaneously under ultrasound guidance, thus resulting in minimal maternal discomfort. General anesthesia with endotracheal intubation was standard practice when procedures lasted a few hours and a mini-laparotomy was occasionally required. The Boston group has accomplished steady technical progress and since 2012 has performed all FCIs under neuraxial anesthesia with a T4 sensory level. After placement of an epidural or combined spinal epidural dosed with bupivacaine and fentanyl the mother is positioned with left uterine displacement. She receives high-flow oxygen, is fitted with headphones, and encouraged to listen to music for distraction. Small doses of benzodiazepines, opioids, or inducing agents can be beneficial to decrease anxiety and agitation. Maternal blood pressure is maintained at 20 percent of baseline with a combination of crystalloids, phenylephrine, and ephedrine. As with any regional technique for obstetric patients, the maternal risks include failed block, intravascular injection, high spinal or aspiration from excessive sedation. In the immediate postoperative period mothers are monitored for preterm labor, and fetal cardiac ultrasound is performed prior to discharge.
Fetal Anesthesia
With the advent of regional anesthesia, the fetus is no longer exposed to anesthetic medications via transplacental passage. All cardiac procedures involve insertion of a needle through the fetus chest wall and heart. Complete fetal immobility is paramount at a time when wires, catheters, and balloons are inflated in the tiny cardiac cavity. The existence of a fetal stress response and its impact on outcome and preterm labor is well established, as is the need to blunt or attenuate this response. Once in optimal position, the fetus receives an intramuscular injection of vecuronium 0.2 mg kg–1 or 1–2 mg kg–1 rocuronium, fentanyl 50 µg kg–1, and atropine 20 µg kg–1. This anesthetic provides suitable conditions for these procedures, which rarely exceed two hours. Monitoring of the fetus is currently limited to Doppler ultrasonography. Continuous observation of the fetal heart rate and function while still in the operating room are required for up to ten minutes after the procedure ends. Fetal hemodynamic instability, specifically profound bradycardia and hemopericardium, have been reported irrespective of the indications for fetal cardiac interventions [69]. Given the limited access to the fetus, a plan for fetal resuscitation must always be put in place [70]. Based on the estimated fetal weight, syringes of epinephrine, 10–20 µg kg–1 and bicarbonate1–2 mEq kg–1 are prepared under sterile conditions for intramuscular or intracardiac administration should fetal resuscitation be necessary. Special attention must be paid to the total volume injected into the fetal cardiac cavity. An improvement of cardiac function frequently follows the administration of resuscitative medications and/or drainage of large pericardial effusion. In extreme circumstances maternal transabdominal compressions can be attempted, although with limited success. The contingencies for emergent delivery of a viable albeit severely compromised fetus must be discussed with the parents during the pre-procedure meeting, with input from the neonatologist, cardiologist, and obstetrician.
Anesthesia for Mid-Gestation Open Fetal Surgery
Maternal Anesthesia
The preoperative evaluation of the mother should include the standard anesthetic history and physical with particular attention paid to symptoms of reflux and supine hypotension, and the exam of the airway and spine. Mothers should be quite healthy overall to minimize the risks of other anesthetic complications. A complete blood count, serum electrolytes, blood urea nitrogen, and creatinine should be measured, with other laboratory and diagnostic studies as indicated by the history, physical, and procedure. An electrocardiogram is helpful to diagnose previously asymptomatic conduction abnormalities that may come to the surface under the deep general anesthesia. Blood should be cross-matched for the mother, and type O, Rh-negative blood for the fetus can be cross-matched against the mother’s sample, as maternal antibodies can cross the placenta. Fetal blood should be available as indicated by the surgical procedure.
A high lumbar or low thoracic epidural catheter may be placed for maternal postoperative analgesia. Indomethacin is also given to the mother. Aspiration prophylaxis, and left uterine displacement precede rapid sequence induction of general anesthesia. After intubation, an arterial catheter and large-bore intravenous catheter are placed. Administration of intravenous crystalloid is often kept to a minimum as fetal surgical patients are at increased risk for pulmonary edema [8]. While other options are possible, the central feature of many anesthetics for open fetal surgery is deep maternal general anesthesia with high-dose volatile anesthetics [71]. Profound uterine relaxation is required by the surgical team to allow easy access to the fetus, to decrease resistance to uterine blood flow, and to minimize the potential risks of abruption. Desflurane is often chosen because its low solubility allows for rapid titration. Approximately twice the minimum alveolar concentration of volatile anesthetic is used. This strategy is obviously not without risks, which include maternal hypotension and fetal cardiac depression [72]. Some centers are advocating a lower dose of volatile anesthetic supplemented by intravenous anesthetics [73,74]. Open mid-gestation surgery should be technically feasible with a pure neuraxial technique and intravenous nitroglycerin for uterine relaxation, but the length of the procedure, stress for the mother, hemodynamic shifts, and need for the operating room team to be able to concentrate fully on the needs at hand while possibly being distracted by an awake patient would make this a challenging proposition.
After the laparotomy, the uterus is exposed, uterine tone is assessed and adjusted as needed, the placental edges are mapped using ultrasound, and the hysterotomy is made with special uterine staplers which keep the edges of the chorionic and amniotic membranes together with the uterine edges. Warmed crystalloid is infused into the amniotic space to maintain fetal warmth and adequate amniotic fluid volume. Fetal incision is made, usually after an intramuscular injection of opioid and muscle relaxant. When the procedure is completed, the uterine edges are closed, and a flap of omentum is also sewn over the hysterotomy site to encourage blood flow and healing. As the uterus is being closed, an intravenous bolus of magnesium sulfate is started, the epidural catheter is activated, and the volatile anesthetic is decreased. The mother is extubated awake.
Fetal Anesthesia
Fetal pain is a controversial topic, but there is clear hormonal and hemodynamic evidence of a fetal stress response to noxious stimuli [24,28]. The fetal stress response can be attenuated by opioids, which are often administered as an intramuscular injection (20 µg kg–1 fentanyl), along with a nondepolarizing muscle relaxant (0.2 mg kg–1 vecuronium). Atropine (20 µg kg–1) may be added in cases where the fetus is felt to be at higher risk for bradycardia. As previously mentioned, volatile anesthetics cross the placenta.
Vascular access to the fetus depends on the needs of the procedure. In cases of myelomeningcele repair, tubing is prepared and primed, but an intravenous catheter would only be placed in emergent situations. In cases where blood loss is likely, peripheral venous access is obtained in the fetus. Monitoring of the fetus is accomplished by direct observation, intermittent assessment of fetal heart rates by ultrasound, or continuous fetal echocardiography, depending on the case. Continuous fetal pulse oximetry can also be used if a hand or foot is accessible. Normal fetal saturations should be between 40 and 70 percent, and the heart rate should be between 110 and 160 bpm. If the fetus becomes bradycardic, the anesthesia team must quickly assess the well-being of the mother, and ensure adequate uteroplacental flow. The patency of the umbilical cord must also be assessed, amniotic fluid volume must be assured, and pressure on the placenta or the fetus itself should be relieved. Echocardiography can guide fluid resuscitation, medication administration, and chest compressions on the fetus.
Anesthesia for the EXIT Procedure
Maternal Anesthesia
The basics of maternal anesthesia for an EXIT procedure are largely the same as for in an open mid-gestation procedure, with a few differences. Since the child will be delivered at the end of the procedure, and no magnesium bolus is needed, maternal fluid administration can be liberalized; 3–5 liters of crystalloid is usually well tolerated. After delivery of the child, reversal of the uterine atony is often accomplished simply by decreasing the volatile anesthetic along with administration of oxytocin, but it is prudent to have additional uterotonics available.
Fetal Anesthesia
The basic principles of anesthesia, in terms of vascular access, medications, and monitoring for the fetus during an EXIT procedure are also the same as for an open mid-gestation procedure, with some exceptions. Some specifics to the EXIT follow. As the fetus will be delivered in a deeply anesthetized state and will likely still be paralyzed from the intramuscular injection, intubation should occur on the field. The fetus is not ventilated, however, until the umbilical cord is about to be clamped, as ventilation and oxygenation of the fetus may start a cascade of events leading to placental separation. Surfactant is often given to the fetus before ventilation. Once the fetal chest has been observed to rise, and the oxygen saturations have begun to increase, the umbilical cord can be clamped and the baby is delivered to a team of neonatologists for further resuscitation or to another operating room for further surgery.
Another difference between an EXIT and mid-gestation open fetal surgery is the need for a second operating room. This room is staffed with another team of nurses and anesthesiologists, and is prepared and ready to go in case the fetus does not tolerate the EXIT procedure, or in case a further procedure is planned.
Anesthesia for Minimally Invasive Noncardiac Procedures
Maternal/ Fetal Anesthesia
The vast majority of minimally invasive noncardiac procedures can be performed with maternal sedation or neuraxial anesthesia. Advances in technology have resulted in smaller instruments [75], and advances in the skills of the operators has allowed a decrease in anesthetic requirement. Anesthetic techniques have ranged from general anesthesia with an endotracheal tube to sedation alone. As for any anesthetic, the needs of the patient and the procedure are taken into consideration in the formulation of an anesthetic plan. A thorough discussion with all team members and with the patient is essential. In many cases, light sedation with local anesthetic infiltration is all that is required. The preoperative evaluation should consider the patient’s expectations of level of awareness, feelings of anxiety, history of panic attacks, symptoms of supine hypotension, and severity of gastroesophageal reflux. The ability of the mother to lie still in a particular position for an hour or more should be explored, as patient position is quite variable for these cases, depending on the location of the placenta. Mild airway obstruction with paradoxical abdominal movement will make the procedure technically more difficult. The gestational age of the fetus should also be considered. If the fetus is considered viable, contingency plans should be made for the emergent delivery and resuscitation of a very premature infant.
Sedation regimens can be quite variable. Various combinations of diazepam and morphine have been used, as have propofol and fentanyl. Midazolam and remifentanil allow rapid titration of analgesia, while the mothers are typically lucid enough to participate in their own positioning for these cases. Local anesthetic infiltration is important if a neuraxial technique is not being used. Regardless of the particular agents used, the goal is to minimize motion and stress in the fetus, while achieving a slightly decreased but safe level of consciousness in the mother.
The fetus is rarely given any medication directly in these procedures, as transplacental passage of the maternal anesthetics is often adequate to decrease fetal motion. If fetal motion persists, intramuscular injection of medications such as opioids or nondepolarizing muscle relaxants can be considered.
The Future
Multidisciplinary teams involved in fetal interventions face numerous challenges. The anesthesiologist plays a major role in the selection of suitable maternal candidates and safe anesthetic techniques for both mother and fetus. Enhanced imaging techniques and improved instrumentation will facilitate the procedures and the field promises to grow steadily. Long-term follow-up is essential to justify and evaluate the interventions.
References


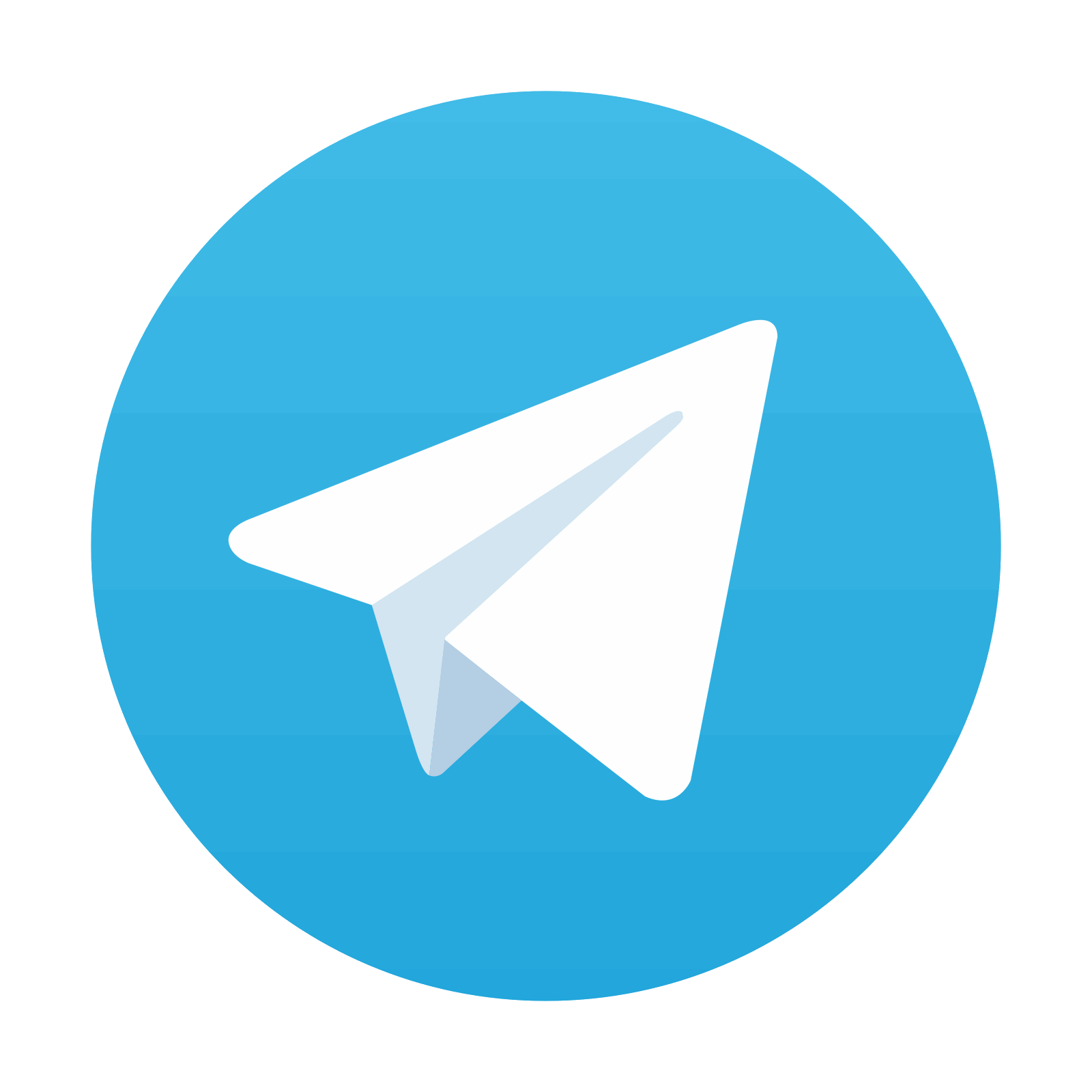
Stay updated, free articles. Join our Telegram channel

Full access? Get Clinical Tree
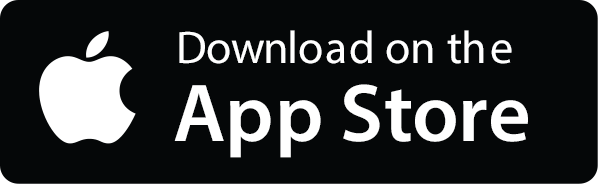
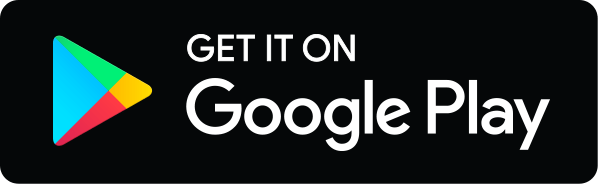
