Introduction
Thomas E. Starzl performed the first successful orthotopic pediatric liver transplant in 1967 at the University of Colorado. The patient was an 18-month-old who survived for the next 400 days [1]. Between January 1988 and December 2013 there were 126 142 liver transplants performed in the United States. Just over one-tenth of these were performed in pediatric patients [2]. While the average number of pediatric transplants has stayed consistent over the past two decades, the pediatric percentage of total liver transplants has been halved. Due to the large number of those requiring transplants, several transplant options have been developed. Liver transplants can be either cadaveric or living donor. To make up for a lack of pediatric-size livers, smaller pediatric patients are able to receive reduced-size grafts or single segment grafts (segment III) or a reduced left lateral segment [3]. As the technique has developed, some studies are showing an improved outcome in living donor transplants over split liver transplants, possibly due to shorter cold and warm ischemia times [4].
General Indications and Contraindications
There are several disease-specific indications for pediatric orthotopic liver transplant. The primary categories of disease include (1) extrahepatic cholestatic liver disease (biliary atresia accounts for half of transplanted patients); (2) metabolic disorders (maple syrup urine disease, α1-antitrypsin deficiency, urea cycle defects, primary oxaluria, Wilson’s disease, and Criggler–Najjar syndrome); (3) intrahepatic cholestasis (TPN cholestasis, Alagille’s syndrome, sclerosing cholangitis); and (4) acute liver failure. Hepatic neoplasms such as unresectable hepatoblastoma, hepatocellular carcinoma, and Budd–Chiari syndrome and cystic fibrosis are also indications for a transplant [1,5].
Absolute contraindications include active and untreated infections at the time of transplant, including actively replicating hepatitis B, acquired immune deficiency syndrome, and cancer outside the liver that is not curable. Relative contraindications include advanced cardiopulmonary disease, multi-organ failure, and human immunodeficiency virus. However, some contraindications may be specific to the individual transplant center.
Pathophysiology and Systemic Manifestations of End-Stage Liver Disease
Some of the indications above, such as the inborn errors of metabolism or neoplasms, do not progress to end-stage liver disease (ESLD), and as such will not develop some of the associated pathophysiologic derangements. End-stage liver disease results from significant hepatic injury or insult and the liver’s subsequent attempts at regeneration, which ultimately results in diffuse hepatic fibrosis and cirrhosis. As the liver becomes damaged, impaired glucose homeostasis and impaired drug clearance develop. Impaired protein synthesis may result in high free plasma levels of drugs that are normally protein-bound. Portal hypertension and liver dysfunction develop, affecting the cardiovascular, pulmonary, renal, neurologic, and hematologic systems [6].
Portal hypertension causes peripheral vasodilation and shunting, resulting in a lowered systemic vascular resistance (SVR) with a decreased sensitivity to vasopressors. This leads to a hyperdynamic state and a rise in cardiac output. The renin–angiotensin–aldosterone system (RAAS) is activated, and antidiuretic hormone is released, resulting in salt and water retention and a decrease in water excretion. This may lead to fluid overload, congestive heart failure, cirrhotic cardiomyopathy (over 70 percent of biliary atresia patients), and pulmonary hypertension [4].
From a respiratory standpoint, the patient develops a restrictive lung disease pattern, alveolar hypoventilation, and a decrease in functional residual capacity (FRC) secondary to abdominal ascites and pleural effusions. There is increased pulmonary blood flow from the fluid overload and increased cardiac output, but intrapulmonary shunting occurs and hypoxic pulmonary vasoconstriction is reduced, predisposing the patient to arterial hypoxemia. Hepatopulmonary syndrome is an abnormal pulmonary vasodilation that results in an increased V/Q mismatch severe enough to cause progressive hypoxemia on room air. Alternately, portopulmonary hypertension may develop as systemic endogenous vasoconstrictors bypass the liver where they are normally metabolized and directly enter the pulmonary circulation. This results in increased pulmonary vascular resistance and may cause acute right-sided heart failure and sudden death [7].
The kidneys are also prone to injury in ESLD from diuretics, acute tubular necrosis and hepatorenal syndrome. Diuretics are used to treat volume overload, as evidenced by ascites and peripheral edema, but patients remain intravascularly depleted due to the portal hypertension and hypoalbuminemia (secondary to decreased hepatic synthesis). Prerenal azotemia contributes to renal injury secondary to aggressive diuretic therapy and paracentesis without appropriate volume replacement. Acute tubular necrosis can develop from decreases in perfusion, secondary to relative hypovolemia and increased abdominal pressure from ascites. Hepatorenal syndrome may develop along with sodium retention and poor sodium excretion secondary to severe renal vasoconstriction. It does not respond to volume expansion as prerenal azotemia does. It has a very poor prognosis but is more common in adults than children. It also resolves with liver transplantation [8].
Neurologically, ESLD patients may develop hepatic encephalopathy. There are conflicting reports concerning its etiology, which is likely multifactorial. The liver metabolizes ammonia into urea, and thus ammonia toxicity is thought to be one of the contributing factors. With ESLD, more aromatic amino acids compared with branched chain amino acids develop. They cross the blood–brain barrier and may be neuroinhibitory in nature. Other neuroinhibitors, hypoglycemia, or decreased metabolism of various drugs may also play a role. Cerebral edema and increased intracranial pressure (ICP) may be present and may be exacerbated by the presence of hyponatremia [1].
The liver is responsible for the synthesis of all coagulation factors, except for von Willebrand’s factor and factor VIII, which are made in the vascular endothelium (and are supranormal in liver disease states). Decreased synthetic liver function results in a coagulopathy. However, decreased levels of antithrombin III, protein C, and protein S may predispose to thrombosis. Anemia is common from variceal bleeding and a decrease in erythropoietin production from the underperfused kidney and bone marrow suppression. Thrombocytopenia is very common in these patients and is a result of decreased thrombopoietin and splenic sequestration.
Surgical Technique
The following section highlights the surgical approach through four phases.
Phase 1: The Pre-Anhepatic or Dissection Stage
Phase 1 occurs from the induction of anesthesia through the devascularization of the diseased liver. The main objective is the dissection of the liver from the surrounding tissues. In patients with previous abdominal surgery (Kasai, redo) this can take significant time and have considerable bleeding due to vascularized adhesions, collaterals, and portal hypertension. Careful attention is paid to the structures of the hilum. The hepatic artery and portal vein are assessed for adequacy of blood flow, which is imperative for the perfusion of the new liver. If needed, the surgical team will augment blood flow with vein or artery grafts, or bypass the native vessels. The next portion involves the dissection of the inferior vena cava (IVC). In the standard complete hepatectomy, this is accomplished with the complete excision of the IVC with the liver. This has significant consequence on preload. The piggyback hepatectomy is the alternative method of managing the IVC and is the one primarily used with pediatric liver transplants. It involves preserving the native IVC. The method may take additional dissection time, but by preserving the native IVC there is one less anastomosis to make later. Pediatric patients generally tolerate this better because complete IVC occlusion is avoided. The liver is then devascularized and the phase is concluded.
Phase 2: The Anhepatic Stage
Phase 2 begins with the removal of the liver and concludes when the IVC and portal vein anastomoses are complete. In the standard hepatectomy, the suprahepatic IVC of the donor graft is clamped and anastomosed to the recipient’s IVC. In the piggyback hepatectomy, a common cuff of the three ostia of hepatic veins is formed and anastomosed to the upper cava of the donor liver. Following this, a separate anastomosis is made between the lower cava of the donor liver and the infrahepatic IVC. Next, a vascular anastomosis is made between the donor portal vein and the recipient portal vein or a mesenteric vein graft. Resumption of flow through the portal vein signifies the end of this phase.
Phase 3: The Reperfusion Stage
The third phase commences with reperfusion of the donor liver through the portal vein and ends when the hepatic artery is reconstructed. Immediately after reperfusion, initial hemostasis should be assessed and achieved. Once stability is maintained, attention can be turned to the hepatic artery anastomosis with the native hepatic artery or aortic graft. Due to the small and delicate nature of the artery, this portion can be time-intensive. Maintaining perfusion pressure and preventing thrombosis is imperative. During the hepatic artery reconstruction, the aorta may be cross-clamped. This typically results in hypertension but in pediatric patients is usually well tolerated.
Phase 4: The Biliary Reconstruction Stage
The final phase of liver transplantation consists of biliary reconstruction. In larger children this is often done with a duct-to-duct anastomosis, as their native ducts are larger. In smaller children or those with diseased fragile ducts, the reconstruction requires a biliary-enteric anastomosis through a choledochojejunostomy to a Roux-en-Y limb. Integrity of this anastomosis is paramount for preventing biliary and enteric leaks, which can lead to significant morbidity and mortality. The final part of the surgery is the closure of the abdomen. Sometimes because of the different sizing ratios of the donor liver to the native body, as well as reperfusion associated inflammation and edema of the allograft, immediate abdominal closure (skin and fascia) is not possible secondary to intra-abdominal compartment syndrome. Even slight increases in intra-abdominal pressure with hemodynamic instability can result in vascular thrombosis (hepatic artery). Attention to ventilatory pressure changes or hemodynamic instability during abdominal closure is important. Delayed abdominal closure, temporary mesh closure, or skin closure alone without underlying fascia may be necessary until the edema subsides.
Anesthesia Management
Preoperative evaluation of the pediatric transplant patient should focus on both the organ systems involved in the underlying disease as well as those affected by the diseased liver.
The cardiac preoperative evaluation should include a pulse oximetry, electrocardiogram, and a transthoracic echo to assess cardiac function, arrhythmias (heart block), valvular dysfunction, and oxygen saturation.
Lung disease in ESLD can be significant. Mild arterial hypoxemia is a common occurrence and results from the physiologic changes of ESLD, namely decreased FRC, total lung capacity, and V/Q mismatch. Hepatopulmonary syndrome, as described above with the classic triad of chronic liver disease, hypoxemia, and intrapulmonary shunting in absentia of primary pulmonary or cardiac disease, can be reversed with liver transplant. Portopulmonary hypertension can also occur secondary to liver disease. Pulmonary hypertension is not as common in pediatric patients, but if it is present it may predict intraoperative morbidity [7]. Underlying disorders such as α1-antitrypsin deficiency can have pulmonary involvement as well (emphysematous changes). Cystic fibrosis patients also have underlying pulmonary disease. Poor preoperative nutrition may result in delayed recovery and prolonged mechanical ventilation [5].
History and physical should elicit baseline hypoxemia, exertional dyspnea, ankle edema, chest pain, and syncope. Various studies to examine depend on history, and these include oxygen saturations, chest X-rays, arterial blood gas on room air and 100 percent oxygen, and transthoracic echocardiogram to assess ventricular performance and estimate pulmonary pressures.
Table 31.1 Anesthesia for liver transplantation
Preop preparation | Transthoracic echo, EKG |
Type and cross | |
CBC, PLT, PT, PTT, metabolic profile | |
IV access | Two peripheral IVs |
In adolescents, consider a rapid infusion catheter (RIC line) | |
Monitors | One arterial line (avoid femoral artery because of arterial cross clamping) |
One central venous line | |
Thromboelastography/ROTEM | |
Standard ASA monitors | |
Stage 1 Hepatectomy | Goal: Resuscitate from dissection of native liver, may be > 1 blood volume |
Immunosuppression: if using Thymoglobulin, premedicate with diphenhydramine, solumedrol 2 mg kg–1, acetaminophen 10 mg kg–1 | |
Antibiotics: piperacillin and tazobactam 75 mg kg–1 | |
Labs: Obtain baseline ABG, glucose and TEG, repeat as needed for resuscitation | |
Stage 2 Anhepatic | Goal: Continue resuscitation as needed and prepare for reperfusion, do not fluid overload, inotropic support may be required to maintain MAP without increasing CVP, correct acid–base status, lower K+ to <4.5 meq l–1, maintain hgb 8–9 g dl–1 |
Immunosuppression: administer solumedrol 10 mg kg–1 when graft is placed on surgical field | |
Labs: Obtain as needed for resuscitation, and ABG just prior to reperfusion | |
Stage 3 Reperfusion | Goal: have epinephrine and calcium in line to treat hypotension after reperfusion |
Labs: recheck ABG and TEG 5 minutes after reperfusion and 30 minutes later, check lactate and continue to repeat lactate for trend | |
Stage 4 | Goal: Continue resuscitation during hepatic artery, biliary and roux-en-Y reconstruction |
Labs: repeat CBC, PLT, PT, PTT, fibrinogen | |
Antibiotics: redose piperacillin and tazobactam every 2 h × 2, and then every 6 h | |
Emergence | Intubation: Extubation may be performed in operating room if abd closed, hemodynamically stable, min. resp. acidosis, no hypoxia and no significant intraoperative events |
Renal dysfunction may result from ESLD, and as described above the kidney may be susceptible to prerenal azotemia, acute tubular necrosis (ATN), and hepatorenal syndrome. Acute renal failure may result and require a combined renal/liver transplant. Studies may include electrolytes, BUN and creatinine, renal ultrasound, urinalysis, and calculating a fractional excretion of sodium (FENa). A metabolic panel will be important to evaluate for hyponatremia, hyperkalemia, and hypocarbia (metabolic acidosis). This information is essential for resuscitation and reperfusion. Low urine output from prerenal azotemia can be improved with a fluid challenge but not if it is secondary to hepatorenal syndrome. Both disorders have a FENa of less than 1 percent. Acute tubular necrosis has a FENa greater than 1 percent.
Anesthetic challenges will vary depending on the stage of the transplant. Induction of anesthesia depends on the current condition of the patient. Often a premedication such as midazolam is given prior to entry to the operating room, unless there is a contraindication (encephalopathy). Preoxygenation is important as the decrease in FRC can lead to rapid desaturation. If the patient doesn’t have the stigmata of portal hypertension (variceal bleeding, ascites), full stomach, cardiac disease or multi-organ failure, then a standard inhalation or intravenous induction followed by endotracheal intubation is generally well tolerated. Otherwise a rapid sequence intravenous induction is more appropriate. A cuffed endotracheal tube (ETT) is used to allow positive pressure ventilation when the surgeons are retracting in the right upper abdomen, and guards against aspiration. If succinylcholine is used with induction, practitioners need to be aware that there may be decreased circulating pseudocholinesterase from decreased synthetic function of the liver and may result in longer duration of action. Also these patients may have impaired renal function and thus may have a preoperative hyperkalemia; succinylcholine may raise the potassium levels by 0.5 mmol L–1.
Maintenance of anesthesia should include inhaled anesthetic agents, neuromuscular blockade, and intravenous pain medications. Nitrous oxide should be avoided in orthotopic liver transplantation (OLT) patients due to its tendency to cause bowel distention, which can obstruct the surgical field. General anesthesia and surgical compression have been shown to decrease hepatic perfusion, which is critical to maintain, especially during hepatic reperfusion. Sevoflurane and isoflurane have been shown to maintain the relationship of hepatic oxygen supply and demand better than halothane or enflurane at 1.2–1.4 MAC [9–13]. Neuromuscular blockade is ideally maintained with cisatracurium secondary to its consistent rate of metabolism by Hoffmann elimination and ester hydrolysis in patients with liver disease. Other nondepolarizing agents (some with active metabolites) are metabolized in the liver and excreted by the kidneys, and thus have variable metabolism. An opioid is an essential part of anesthetic maintenance. Fentanyl, morphine, or hydromorphone are commonly used.
Monitoring should include standard ASA monitors, urinary catheter, temperature, nerve stimulator, arterial blood pressure, and central venous pressure (CVP). Arterial monitoring is preferred in an upper extremity secondary to possible aortic cross-clamping during hepatic artery anastomosis. Two large-bore IVs should also be placed above the diaphragm to allow easy flow to the heart and circulation as blood loss can be massive and rapid during these cases, especially during retransplantation or previous abdominal surgeries [5]. Given the large abdominal incision, large surface area to volume ratio, and volume resuscitation, it is important to use warming blankets, fluid warmers, and heat the room as necessary.
The ventilation goal is to maintain a normal end-tidal CO2 and to maintain peak airway pressures less than 20 cmH2O while maintaining adequate tidal volumes (6–8 ml kg–1). This may be difficult depending on pulmonary compliance. PEEP is added to prevent progressive atelectasis.
During the pre-anhepatic stage hypotension is very common. Bleeding can be significant when the liver is dissected, collaterals are ligated, and adhesions are taken down. Preexisting coagulopathy can make the bleeding worse. The liver is lifted during the dissection of the major hepatic vessels on its posterior side, and this can temporarily obstruct the IVC, decreasing venous return. The goals during this phase are to maintain euvolemia, transfuse to maintain the hemoglobin at 8–9 g dl–1, and correct coagulopathy to minimize bleeding. It is important to not over-transfuse (hgb > 10 g dl–1), or correct the coagulation to normal because this may increase the risk for hepatic artery thrombosis. Maintaining euvolemia is important for preparing the patient for vena cava cross-clamping in phase 2.
The second surgical phase is the anhepatic stage. The goals of anesthetic management for this phase are to maintain euvolemia, support the hemodynamics and prepare for reperfusion. Ideally the warm ischemia time for the donor liver is less than 60 minutes, so this phase may be shorter. In the standard complete hepatectomy, the portal vein and IVC is cross-clamped and the resulting decrease in cardiac preload or venous return decreases the cardiac output and CVP and increases the SVR and heart rate. Using the piggyback approach, these changes may be partly compensated since there is some flow through the IVC. The ability to tolerate vena cava cross-clamping depends on the development of collateral blood flow. A study comparing pediatric liver transplants for biliary atresia (BA) with those for glycogen storage diseases (GSDs) showed that the CVP and blood pressure were significantly lower and heart rate significantly higher for the GSD group, demonstrating that the cardiac output was more adversely affected during the vena cava cross-clamping. A possible explanation for this is that the BA group was more likely to have developed cirrhosis and significant collaterals than the GSD group (which did not progress to cirrhosis) and thus was better able to maintain venous return and tolerate caval cross-clamping [14]. Colloid or crystalloid should be used to maintain euvolemia but vasopressor support may be required to maintain the blood pressure. The donor liver is then flushed (retrograde direction) in order to eliminate hepatic air bubbles that can cause an embolus and preservatives that might lead to hyperkalemia or cardioplegic effects. Bleeding can be significant during this stage as well; the coagulation factors may be diluted and the platelets may be consumed.
The reperfusion stage is the third phase of the liver transplant. As the graft is reperfused, the preservation solution is flushed back into the recipient’s circulation. This solution is cold, acidotic, heparin-containing, and high in potassium. A common preservative used for the liver graft is histidine-tryptophan-ketoglutarate (HTK). This solution has less potassium than University of Wisconsin solution (used for bowel transplantation) and results in less hyperkalemia immediately following reperfusion. The donor liver can also form endogenous heparinoids that are released upon reperfusion. Post-reperfusion syndrome is a phenomenon characterized by cardiovascular dysfunction, bradyarrhythmias, hypotension, and increased pulmonary artery pressure and CVP. Although the clinical severity may vary, all patients experience some form of reperfusion syndrome when the graft is reperfused [15,16]. Preparing for reperfusion is imperative to minimize the associated hemodynamic instability, arrhythmias, and possible left ventricular dysfunction. This includes increasing the inspired oxygen percentage, increasing minute ventilation, decreasing the inhaled anesthetic, correcting hyperkalemia prior to reperfusion, having calcium immediately available to treat hyperkalemia, and having vasopressors such as epinephrine and dopamine in-line for hemodynamic instability. Blood should be drawn immediately after reperfusion to evaluate for hyperkalemia. Lactate levels should also be measured after reperfusion and repeated during the reconstruction phase. A declining lactate level indicates graft function.
The management of coagulation is an essential part of anesthetic maintenance. In addition to major acute blood loss seen during surgery and the diseased liver’s hypocoaguable state and thrombocytopenia, coagulation can change during the surgery because of consumption and dilution. Various coagulation tests such as prothrombin time (PT), partial thromboplastin time (PTT), D-dimer, hematocrit, and platelet count should be checked at certain time points during the case. Thromboelastography (TEG) is also used to help guide coagulation therapy. Fibrinolytic activity can increase during phase 2 and peak during phase 3, and can be observed on the TEG. This may necessitate the use of antifibrinolytics. These should only be started in consultation with the surgical team. Pediatric transplant patients are more prone to hepatic artery thrombosis (HAT) than their adult counterparts. Risk factors include age <2 years old, the hypercoagulability that develops secondary to a reduction in protein C and antithrombin III, high fibrinogen levels, and the increase in plasminogen activator inhibitor seen in children but not adults [17].
The type of graft may have an impact on the type of transfusions required during the intraoperative period. A study of 157 patients comparing deceased and live donor pediatric liver transplantations found that live donor transplants required more packed red blood cells (PRBCs), crystalloid, and colloid on a per kilogram basis, but required less fresh frozen plasma [18]. It should be noted that in this study the live donor transplants were significantly longer in duration.
Other metabolic changes that occur include derangement of plasma levels of sodium, potassium, glucose, calcium, magnesium, and temperature. Hyponatremia may be present at induction and care must be taken not to correct this deficiency too quickly because of the risk for central pontine myelinolysis. Sodium bicarbonate and 5 percent albumin have a higher sodium content and they may correct hyponatremia too quickly.
Hypokalemia may also be present at induction due to chronic diuretic use and metabolic alkalosis. It is not typically treated because a sudden acute rise in potassium levels following graft reperfusion will occur. Hypokalemia is the more common metabolic disturbance during the post-reperfusion period in pediatric liver transplants [19]. Hyperkalemia should always be treated. Packed red blood cells may be washed in a cell saver prior to being transfused, and acidosis should be treated to help prevent hyperkalemia.
Glucose metabolism is altered in chronic liver disease and glycogen stores may be depleted. In addition, insulin may not be degraded and thus persist longer in circulation. Hypoglycemia may occur and should be monitored throughout the intraoperative period. Conversely, hyperglycemia is common from intravenous steroids given to start immunosuppression. Hypocalcemia and hypomagnesemia can develop secondary to chelation from the citrate in PRBCs. This may result in coagulopathy, hemodynamic instability, and arrhythmias, and may require replacement.
Hypothermia is common from the large abdominal incision, cold donor, cold preservation solution, and cold intravenous fluids. Hypothermia may cause platelet dysfunction, fibrinolysis, and bleeding in an already coagulopathic patient. Measures to maintain euthermia include covering the head in plastic wrap, keeping the patient and operating room table dry, warming the operating room, using a humidifier in the anesthetic circuit, warming IV fluids, and using forced-air warmers. Finally, the head should be rotated every hour to minimize pressure sores on the scalp. This may result in permanent alopecia.
Following the end of the final phase of surgery, the abdomen may not be closed depending on the size differential between the donor liver and patient as well as edema of the bowels. Both of these can result in an abdominal compartment syndrome or may alter the perfusion of the new graft. The patients are often left intubated after the surgery. Some centers extubate these patients immediately after surgery. In general, fast-tracking extubation can occur if the patient is hemodynamically stable, the abdomen is closed (fascia may be open), and if there are no significant intraoperative complications. Patients that do not have portal hypertension (maple syrup urine disease) may bleed less and may be ideal candidates for fast-tracking. Risk factors for prolonged mechanical ventilation include intraoperative bleeding, mechanical ventilation prior to transplant, retransplantation, and acute liver failure. The data seem to indicate that impaired health prior to transplant is a major cause for prolonged ventilation [20].
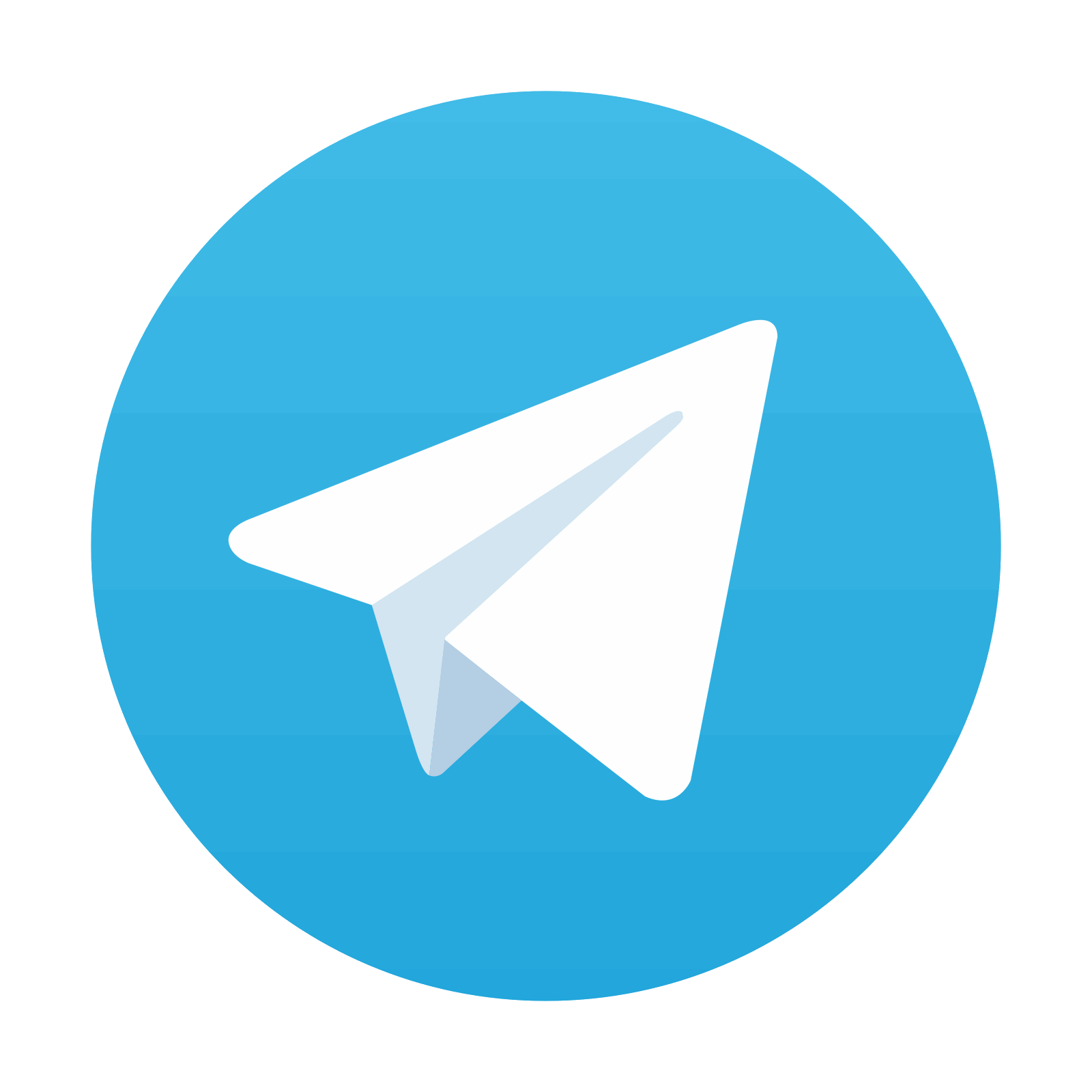
Stay updated, free articles. Join our Telegram channel

Full access? Get Clinical Tree
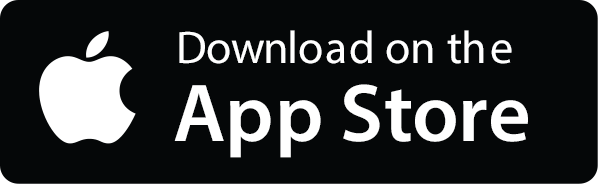
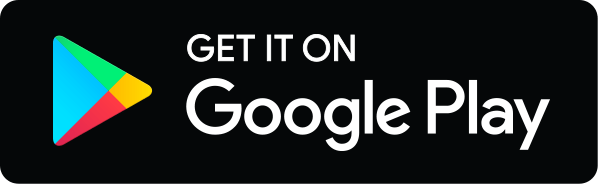