Airway pressures: measured at proximal airways during mechanical ventilation
Peak inspiratory pressure (PIP)
Maximal pressure measured at end inspiration
Determined by elastic and resistive properties of the respiratory system
Plateau pressure (Pplat)
Estimation of peak alveolar pressure
Measured by applying end-inspiratory breath hold for 0.5 to 2 seconds (zero flow)
Determined by tidal volume and compliance of respiratory system
Measurement only valid during passive inflation (use assist control mode, not pressure support ventilation)
Compliance
Distensibility of system; inverse of elastance
Change in volume divided by the pressure required to produce that volume
Respiratory system compliance (compliance of chest wall + lung)
CRS = ΔV/ΔP = VT/(Pplat – PEEP)
Normal value for mechanically ventilated patient is 50 to100 mL/cm H2O
Chest wall compliance
Calculated using esophageal pressure (PES) as surrogate for pleural pressure (PPL)
CCW = ΔV/ΔP = VT/ΔPPL
Normal value = 200 mL/cm H2O
Causes of increased chest wall compliance: paralysis, flail chest
Causes of decreased chest wall compliance: abdominal distension, thoracic deformities, chest wall burns/edema, increased muscle tone
Lung compliance
Calculated using transpulmonary pressure
CL = ΔV/ΔP = VT/((Pplat – PEEP) – ΔPPL)
Normal value = 200 mL/cm H2O
Causes of increased lung compliance: emphysema
Causes of decreased lung compliance: consolidation, atelectasis, pulmonary edema, pulmonary fibrosis, pneumonectomy, mainstem intubation
Resistance
Opposition to air flow within airways
Change in pressure divided by flow
Inspiratory resistance:
RI = (PIP – Pplat)/V˙
Set ventilator for constant inspiratory flow
Normal value for intubated and mechanically ventilated patients < 10 cm H2O/L/s at flow of 1 L/s
Causes of increased resistance: bronchospasm, secretions, small endotracheal tube
Pressure-Volume Curves
Depict static relationship between pressure and volume of respiratory system.
Constructed by measuring pressure as lungs are inflated or deflated (different curves due to hysteresis); accurate measurements require sedation and often paralysis.
Normal shape is sigmoidal with lower and upper inflection points.
Lower inflection point may represent the closing volume of the alveoli. Similarly, the upper inflection point may represent overdistension of the alveoli.
Clinical utility of adjusting ventilator to avoid alveolar collapse and overdistension based on pressure-volume curve is not clear.
SUGGESTED READINGS
Bigatello LM, Davignon KR, Stelfox HT. Respiratory mechanics and ventilator waveforms in the patient with acute lung injury. Respir Care. 2005;50(2):235-245.
Hess DR, Bigatello LM. The chest wall in acute lung injury/acute respiratory distress syndrome. Curr Opin Crit Care. 2008;14(1):94-102.
Hess DR, Medoff BD, Fessler MB. Pulmonary mechanics and graphics during positive pressure ventilation. Int Anesthesiol Clin. 1999;37(3):15-34.
Jubran A, Tobin MJ. Monitoring during mechanical ventilation. Clin Chest Med. 1996;17(3):453-473.
Lucangelo U, Bernabè F, Blanch L. Lung mechanics at the bedside: make it simple. Curr Opin Crit Care. 2007;13(1):64-72.
Lucangelo U, Bernabé F, Blanch L. Respiratory mechanics derived from signals in the ventilator circuit. Respir Care. 2005;50(1):55-65.
3.2
Respiratory Failure, Mechanical Ventilation, and Weaning
Jean Kwo and Daniel Chipman
Definitions
Respiratory failure: inability of the lungs to perform their basic task of gas exchange: the transfer of oxygen from inhaled air into the blood and the transfer of carbon dioxide from the blood into exhaled air
Type 1 respiratory failure: hypoxemic (PaO2 < 60 mmHg)
Due to ventilation/perfusion (V/Q) mismatch or shunt
Type 2 respiratory failure: hypercarbic (PaCO2 > 45 mmHg)
Due to increased CO2 production (fever, sepsis, burns, overfeeding) or decreased minute ventilation (decreased respiratory rate or tidal volume, increased dead space)
Common Causes to Remember
Indications for Mechanical Ventilation
Epidemiology
Data from study in 2010: 790,257 hospitalizations involving mechanical ventilation in 2005; rate of 2.7 per 1,000 population
Mortality: 34.5%
Incidence and mortality increases with age.
Key Pathophysiology
Acute respiratory failure can result from pathology affecting any component of the respiratory pathway from the central nervous system (CNS) control centers to the spinal cord to the muscles of respiration to the lungs and their vasculature.
Causes are often multifactorial.
Understanding the cause of the acute respiratory failure is key to treating and reversing it.
Differential Diagnosis
CNS: drugs, sedatives, central sleep apnea, encephalopathy
Spinal cord: trauma, transverse myelitis
Neuromuscular system: polio, Guillain-Barré syndrome, critical care/steroid myopathy
Chest wall/pleural space: kyphosis, obesity, pleural effusion, pneumothorax, hemothorax
Upper airways: obstructive sleep apnea, vocal cord paralysis, tracheomalacia
Lower airways: bronchospasm, chronic obstructive disease, infection, CHF
Lung parenchyma: infection, interstitial lung disease
Pulmonary vasculature: pulmonary embolism, pulmonary hypertension
Management and Treatment
Mechanical ventilation may be needed to treat hypoxemia, hypercarbia, and respiratory fatigue.
Ventilator settings should be chosen to not only provide adequate oxygenation and ventilation but also to promote patient-ventilator synchrony, maintain alveolar recruitment while avoiding alveolar overdistention, and avoid auto-positive end-expiratory pressure (PEEP) and ventilator-induced lung injury.
Understanding the variables that determine how a ventilator breath is delivered, how to set the ventilator, and ventilator modes is essential to avoid the harmful effects of mechanical ventilation.
Ventilator settings
Should be tailored to underlying condition resulting in respiratory failure
Tidal volume
Target tidal volume of 4 to 10 mL/kg
Should use predicted body weight (based on gender and height of patient) to determine tidal volume:
Males: Predicted Body Weight (PBW) = 50 + 2.3 × (Height (inches) − 60)
Females: PBW = 45.5 + 2.3 × (Height (inches) − 60)
In patients with acute respiratory distress syndrome (ARDS), acute lung injury (ALI), should decrease tidal volumes to 6 mL/kg or less because lower tidal volumes are associated with a lower mortality rate than higher tidal volumes.
Patients with neuromuscular disease may need higher tidal volumes (8 to 10 mL/kg) to prevent atelectasis.
Keep plateau pressures < 30 cm H2O.
Consider decreasing tidal volume if plateau pressure exceeds 28 cm H2O in patients with normal chest wall compliance.
Inspiratory time
Set directly in pressure-controlled ventilation
Determined by tidal volume, flow rate, and flow pattern in volume-controlled ventilation
Determined by cycle off (flow deceleration) sensitivity in pressure support ventilation
If set incorrectly (too long or too short) may result in patient/ventilator asynchrony
Should be set shorter than expiratory time to prevent inverse I:E ratio
Respiratory rate
Minute ventilation is determined by tidal volume and respiratory rate.
Aim for minute ventilation of 8 to 12 L/min (higher with increased CO2 production and/or deadspace).
Adjust rate to achieve desired pH and pCO2.
High respiratory rates can be associated with air-trapping and auto-PEEP.
I:E ratio
Set directly in pressure-controlled ventilation
Determined by tidal volume, respiratory rate, and flow pattern in volume-controlled ventilation
Longer inspiratory time can result in improved oxygenation but is also associated with auto-PEEP and hemodynamic instability.
Expiratory time should be longer than inspiratory time and is determined by inspiratory time and respiratory rate.
PEEP
Increases Functional residual capacity (FRC), decreases shunt, improves lung compliance
Can titrate by
Oxygenation
PEEP tables
Pressure-volume curves—lower inflexion point
PEEP trial to determine best respiratory system compliance
PEEP titrated using esophageal pressure
No evidence for best method of titrating PEEP
Excessive PEEP may cause overdistention increasing dead space and reducing lung compliance.
Proportional assist
Used with proportional assist ventilation (PAV)
Determines the percentage of the work of breathing that will be provided by the ventilator
Increasing the level of proportional assist results in decreased work for the patient.
NAVA level
Used with neurologically adjusted ventilatory assist (NAVA)
Determines the level of support provided by the ventilator
Common Modes of Mechanical Ventilation
Indications
Pulmonary edema
Acute COPD exacerbation
Immunocompromised patient with acute respiratory failure
Postextubation in patients at high risk for reintubation
Chronic heart failure
Stridor not requiring immediate reintubation
Multiple comorbidities
Severe COPD
Obesity hypoventilation syndrome
Contraindications
Cardiac or respiratory arrest
ARDS
Severe encephalopathy (e.g., GCS < 10)
Severe upper gastrointestinal bleeding/gastric or esophageal surgery
Hemodynamic instability or unstable cardiac arrhythmia
Facial surgery, trauma, or deformity
Inability to cooperate/protect the airway
Inability to clear respiratory secretions
High risk for aspiration
Use of NIV requires frequent reassessment for success or failure. Delay in intubation may result in increased mortality. Consider prompt intubation if there is
Increased accessory muscle use
Increased respiratory rate
Worsening arterial blood gas
Increased dyspnea
Increased heart rate
Discontinuation of mechanical ventilation
Assessment of potential for ventilator discontinuation
Is there evidence of resolution of underlying disease that led to respiratory failure and need for mechanical ventilation?
Is gas exchange adequate?
Oxygenation: PaO2/FiO2 > 150 to 200, PEEP < 8 cm H2O, and FiO2 <40% to 50%
Ventilation: pH ≥ 7.25
Is the patient hemodynamically stable (no active myocardial ischemia or clinically important hypotension requiring vasoactive drug therapy)?
Can the patient initiate an inspiratory effort?
Sedation should be adjusted so patient can cooperate with weaning process.
The spontaneous breathing trial (SBT) is the best predictor of successful ventilation discontinuation.
SBT can be performed with a T-piece or with ventilator settings PS 0/PEEP 0.
A low level of PS (5 to 7 cm H2O) may be used to overcome resistance through an endotracheal tube.
Most patients who tolerate an SBT of 30 to 120 minutes can be discontinued successfully from mechanical ventilation.
Criteria to determine tolerance of an SBT
Set ventilator to comfortable, nonfatiguing mode for patient.
Look for cause of failed SBT.
Most common cause is need for further resolution of underlying disease.
Other contributory causes include dynamic hyperinflation, myocardial ischemia and cardiac disease, critical illness neuropathy/myopathy.
Tracheostomy tube malposition should be considered if patient fails to wean off a low level of PS or develops respiratory distress quickly after removal of positive pressure ventilation.
For example, bronchoscopic examination might reveal occlusion of airway device by the posterior membrane of the trachea.
SBT should be repeated once the cause for failed SBT is corrected.
Use of weaning protocols is associated with reductions in weaning duration, total duration of mechanical ventilation, and ICU length of stay.
Criteria for extubation
Successful completion of SBT
Ability to protect airway and clear secretions
Quality of cough
Quantity of secretions
Frequency of suctioning
Absence of upper airway edema
Can occur with prolonged mechanical ventilation, smaller airways (women, children), trauma, repeated or traumatic intubations
May be diagnosed with a leak test and/or direct visualization of airway with laryngoscopy or fiberoptic examination of glottis
Leak test is performed with patient breathing spontaneously, off positive pressure ventilation, and endotracheal tube cuff deflated.
Presence of a leak suggests absence of significant airway swelling.
However, absence of a leak is not necessarily an absolute contraindication for extubation.
Methylprednisolone 20 mg IV 12 hours before extubation and every 4 hours until tube removal has been shown to decrease the incidence of postextubation laryngeal edema and reintubation secondary to laryngeal edema.
Patient cooperation
Patient should be awake, alert, comfortable, and able to follow instructions to cough.
Anxiolytics and pain medications may be titrated to achieve above goal.
Outcomes
Mortality associated with mechanical ventilation: 30% to 60% depending on patient population studied.
Predictors of mortality include advanced age, a medical admitting diagnosis (vs surgical), respiratory failure due to primarily a pulmonary cause, and the inability to wean from mechanical ventilation.
Predictors of failure to discontinue mechanical ventilation include a medical admitting diagnosis (vs surgical) and history of underlying respiratory disease.
One study using ICD-9 codes to identify patients receiving mechanical ventilation found that only 30.8% of patients were discharged home from the hospital; 28.2% of patients were discharged to a nursing facility.
In another study of Medicare beneficiaries aged ≥ 65, mechanical ventilation during hospitalization was associated with a 1-year mortality of 72% and significant disability.
Estimated costs were $27 billion nationally or 12% of all hospital costs.
SUGGESTED READINGS
Barnato A, Albert S, Angus D, et al. Disability among elderly survivors of mechanical ventilation. Am J Respir Crit Care Med. 2011;183: 1037-1042.
Bigatello L, Stelfox H, Berra L, et al. Outcome of patients undergoing prolonged mechanical ventilation after critical illness. Crit Care Med. 2007;35:2491-2249.
Blackwood B, Alderdice F, Burns K, et al. Use of weaning protocols for reducing duration of mechanical ventilation in critically ill adult patients: cochrane systematic review and meta-analysis. BMJ. 2011;342:c7237.
de la Olivia P, Schuffelmann C, Gomez-Zamora A, et al. Asynchrony, neural drive, ventilatory variability and COMFORT: NAVA versus pressure support in pediatrics. A non-randomized cross-over trial. Intensive Care Med. 2012;38:838-846.
Esan A, Hess D, Raoof S, et al. Severe hypoxemic respiratory failure: ventilation strategies. Chest. 2010;137:1203-1216.
Estaban A, Frutos F, Tobin MJ, et al. A comparison of four methods of weaning patients from mechanical ventilation. N Engl J Med. 1995;332:345-350.
Esteban A, Frutos F, Ferguson ND, et al. Noninvasive positive pressure ventilation for respiratory failure after extubation. N Engl J Med. 2004;350:2452-2460.
Francois B, Bellissant E, Gissot V, et al. 12-h pretreatment with methylprednisolone versus placebo for prevention of postextubation laryngeal oedema: a randomized double-blind trial. Lancet. 2007;369:1083-1089.
Grasso S, Puntillo F, Mascia L, et al. Compensation for increase in respiratory workload during mechanical ventilation. Pressure-support versus proportional-assist ventilation. Am J Respir Crit Care Med. 2000; 161(3 Pt 1):819-826.
MacIntyre N. Current issues in mechanical ventilation for respiratory failure. Chest. 2005;128:561S–567S.
MacIntyre NR, Cook DJ, Ely EW, et al. Evidence-based guidelines for weaning and discontinuing ventilatory support. Chest. 2001;120: 375S-395S.
Mercat A, Richard J, Vielle B, et al. Positive end-expiratory pressure setting in adults with acute lung injury and acute respiratory distress syndrome: a randomized controlled trial. JAMA. 2008;200: 637-645.
NIH/NHLBI ARDS Network. Ventilation with lower tidal volumes as compared with traditional tidal volumes for acute long injury and acute respiratory distress syndrome. N Engl J Med. 2000;342:1301-1308.
Sinderby C, Navalesi P, Beck J, et al. Neural control of mechanical ventilation in respiratory failure. Nat Med. 1999;5:1433-1436.
Wunsch H, Linde-Zwirble W, Angus D, et al. Epidemiology of mechanical ventilation use in the United States. Crit Care Med. 2010;38: 1947-1953.
Acute Respiratory Distress Syndrome and Acute Lung Injury
Sheri Berg
Introduction
Syndrome of respiratory failure is characterized by
Onset of acute respiratory is distress syndrome (ARDS)
PaO2/FiO2 ≤200 (ARDS) and ≤300 Acute Lung Injury (ALI)
Bilateral (patchy, diffuse, or homogenous) infiltrates in chest x-ray (CXR)
No clinical evidence of left atrial hypertension (diagnosis no longer requires pulmonary capillary wedge pressure [PCWP]; ECHO can be used to diagnose systolic or diastolic dysfunction as a cause of pulmonary edema).
Common Causes to Remember
Epidemiology
Affects more than 200,000 people in the United States per year
Associated mortality is 30% to 35%.
Key Pathophysiology
Systemic inflammatory response that injures the lungs (and likely other organs as well); leads to the classic “diffuse alveolar damage”:
Alveolar injury: involves all aspects of the alveolar-capillary interface; damage to the type 2 cells amplifies the inflammatory response (remember, these are the surfactant-producing pneumocytes that are also responsible for clearing edema via fluid transport).
Exudative phase: interstitial and alveolar edema develop from the increased permeability of damaged endothelium; exudates eventually line the alveolar walls with the characteristic hyaline membranes; the combination of edema, collapse, and consolidation leads to decreased lung compliance and produces hypoxemia (hallmarks of ARDS).
Fibroproliferative phase: acquisition of chronic inflammatory characteristics can occur within 1 to 2 weeks; fibroblasts and collagen deposits lead to destruction of air spaces and interstitial fibrosis.
Pulmonary edema contributes to hypoxemia via shunting (perfusion, no ventilation) and decreases compliance as the lungs “stiffen.”
Can attempt to increase PaO2 via recruitment of nonventilated alveoli (PEEP) and increasing the FiO2
Hypoxic pulmonary vasoconstriction (PA constriction in the setting of hypoxia to divert blood away from hypoventilated alveoli) is a naturally occurring phenomenon that helps to attenuate hypoxemia.
Decreased pulmonary blood flow, vascular occlusion, and airway overdistention can create areas of dead space and lead to hypercapnia.
Thrombosis of small arteries and remodeling often obliterates sections of pulmonary vasculature, which can lead to worsening edema, pulmonary hypertension, and progression to right heart failure.
Differential Diagnosis
A number of noninfectious processes mimic ARDS:
Acute eosinophilic pneumonia: can also see severe hypoxemia (PaO2 < 60 mmHg), diffuse pulmonary infiltrates—as in ARDS—but can be diagnosed by an increased number of eosinophils in bronchoalveolar lavage (BAL) fluid; treatment with corticosteroids results in rapid reversal of respiratory failure.
Acute cryptogenic organizing pneumonia (COP)/bronchiolitis obliterans organizing pneumonia (BOOP): hypoxemia, patchy pulmonary infiltrates, BAL will reveal a predominance of lymphocytes; alveolar exudates can lead to fibrosis; patients may have underlying chronic inflammatory disease (which can help with the diagnosis of COP/BOOP); treat with corticosteroids.
Acute hypersensitivity pneumonitis: results from inhalation of particulate matter, hypoxemia, bilateral opacities can be observed; differentiated from ARDS as there is a clear inciting agent; treat with corticosteroids.
Diffuse alveolar hemorrhage: flooding of alveoli with blood and BAL will be grossly sanguineous; hypoxemia (can be severe); usually an underlying vasculitis; mainstay of treatment is corticosteroids and immunosuppressive agents.
Management and Treatment
Diagnose and begin to treat the underlying condition; support hemodynamics, treat infections; support other organ systems while the lungs recover.
THE VENT: initiate lung-protective ventilation to help minimize further injury to undamaged alveoli.
Small tidal volumes (≤6 cc/kg), low airway pressures (plateau pressures < 30 cm H2O) may improve survival = “ARDS – net protective ventilation.”
As this mechanical ventilation strategy (low tidal volumes and plateau pressures) often results in a concomitant respiratory acidosis, permissive hypercapnia may need to be tolerated. If the respiratory rate cannot be increased further (shoot for pH ~7.3 and PaCO2 < 55; however, this is not always possible, especially if there is an underlying metabolic acidosis (as seen in sepsis)) and a severe acidosis develops, then the patient may require renal replacement therapy (CVVH) to help correct the acidosis; should be seriously considered once the pH < 7.2.
Improved lung function, decreased duration of mechanical ventilation, and lower number of deaths from hypoxia with moderate (vs low) PEEP levels; “Best PEEP” measurements can be made, although they do not appear to improve outcome.
Although there is not enough evidence to support routine usage, PEEP can also be titrated to positive transpulmonary pressure via estimation from an esophageal balloon catheter.
Routine use of recruitment maneuvers has not been shown to be beneficial (and can further compromise patients who are gravely hemodynamically unstable), although it may be worth a try in patients with refractory hypoxemia.
The underlying goal is prevention of ventilator-induced lung injury (VILI), which can potentially provide added damage to the alveoli.
Types of VILI include:
Barotrauma: excessive airway pressures
Volutrauma: overdistention of alveoli
Atelectrauma: opening alveoli (inspiration) and collapse (expiration) cause shear injury
Biotrauma: release of proinflammatory cytokines from disproportionate mechanical forces on the lung
Conservative fluid management/restriction has been shown to be beneficial in ALI/ARDS, with associated improvement of lung function, respiratory mechanics, gas exchange, and minimizing pulmonary edema.
“Proning”: it is thought that the prone position initiates redistribution of ventilation to previously collapsed areas of the lung; it has been shown that many patients demonstrate improved oxygenation; however, the effects are transient and mortality is not reduced.
Remember: hemodynamic instability can rapidly ensue when patients are prone and extreme vigilance must be employed with regard to maintenance of the secured airway.
To date, its use is inconclusive and has not been shown to decrease time spent on the ventilator or increase survival.
Neuromuscular blockade (NMB)
A recent study concluded that initiation of neuromuscular blockade in patients meeting ARDS criteria may be of benefit, as mortality was reduced with the use of cisatracurium for 48 hours; the benefit is likely due to improvement of gas exchange via enhancement of patient-ventilator synchrony and thus, the ability to provide proficient lung-protective ventilation.
There is concern of prolonged weakness and myopathy of critical illness; however, the study failed to demonstrate an increase in myopathy (this is a well-known consequence with an increased incidence associated with the use of steroid neuromuscular blockers).
Other drugs
Nitric oxide will improve oxygenation and decrease pulmonary vascular resistance, but the effects are temporary and are usually a bridge to extracorporeal membrane oxygenation (ECMO) which can allow the lungs to “rest”; unfortunately, neither has rendered a survival benefit in patients with ARDS.
Steroids were postulated to help attenuate inflammation (which is thought to be involved in the pathogenesis of ARDS); however, they failed to demonstrate any benefit on survival (one study showed increased mortality when steroids were started 14 days after the onset of ALI/ARDS).
Other drugs that have been studied and have failed to improve survival include NSAIDs, antioxidants, ketoconazole, surfactant, neutrophil elastase inhibitors, and pentoxyfilline.
Current research is evaluating the possible benefits of statins and antiplatelet drugs in ARDS.
Salvage therapies
High frequency ventilation: more often used in the neonate and pediatric population; very small tidal volumes with fixed mean airway pressures and high respiratory rates; airway pressures remain low in hopes of minimizing VILI; however, short expiratory times can lead to auto-PEEP (which will not be reflected when measuring airway pressures).
Inverse ratio ventilation: extends the inspiratory time concept with aims at improving gas exchange at lower levels of PEEP; can also lead to auto-PEEP, adverse hemodynamic effects, and need for sedation in most patients.
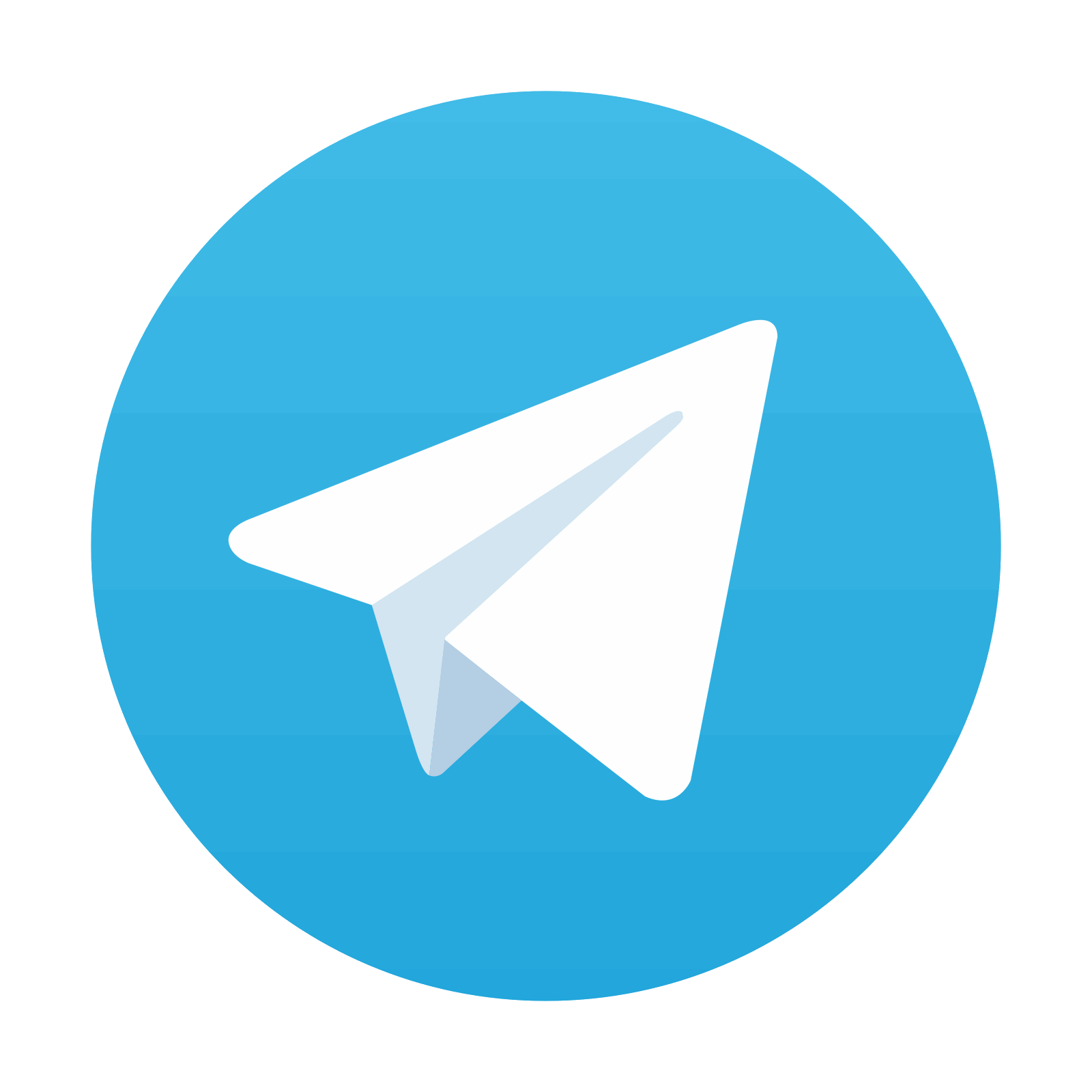
Stay updated, free articles. Join our Telegram channel

Full access? Get Clinical Tree
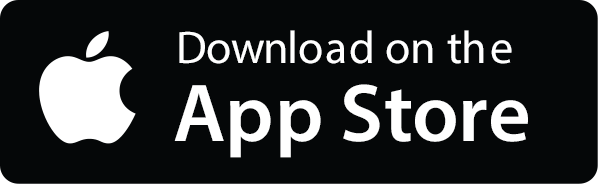
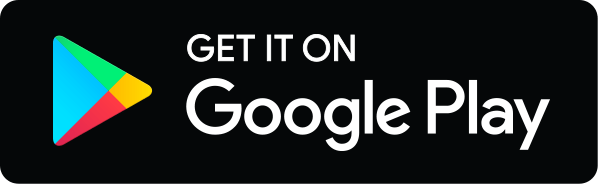