Section 29 Toxicology
29.1 Approach to the poisoned patient
Introduction
Deliberate self-poisoning is one of the commonest reasons for general hospital admission in the UK1 and accounts for 1–5% of all public hospital admissions in Australia.2,3 The bulk of the medical management of cases presenting to hospital is carried out in the emergency department (ED), and the emergency physician is expected to be expert in the field. Although the management must vary considerably according to the nature and severity of the poisoning, some general principles apply.
Above all, it must be remembered that the acute overdose presentation is only a discrete time-limited event in the course of the underlying condition, which is usually psychiatric or social in origin.
Pathophysiology and clinical features
The most frequent life-threatening respiratory complication of poisoning is ventilatory failure, which is usually a consequence of CNS depression. Less commonly, it is secondary to ventilatory muscle paralysis. The frequency and depth of respirations are reduced. Respiratory failure may also be caused by direct pulmonary toxicity, or complications such as pulmonary aspiration or non-cardiogenic pulmonary oedema (Table 29.1.1).
Table 29.1.1 Toxic causes of respiratory failure
Cardiovascular manifestations of poisoning include tachycardia, bradycardia, hypertension, hypotension, conduction defects and arrhythmias (Table 29.1.2). Bradycardia is relatively rarely observed and is associated with a number of potentially life-threatening ingestions. Tachycardia is commonly observed and is usually benign. It may be due to intrinsic sympathomimetic or anticholinergic effects of a drug, or a reflex response to hypotension or hypoxia. Hypotension is also commonly observed and may be due to a number of different causes (Table 29.1.2). Hypertension is unusual. Severe hypertension is usually associated with illicit drug use and is important because it may produce complications such as intracerebral haemorrhage.
Table 29.1.2 Cardiovascular effects of poisoning
CNS manifestations of poisoning include decreased level of consciousness, agitation or delirium, seizures and disordered temperature regulation. A decreased level of consciousness is a common presentation of poisoning and is associated with many drugs, some of which are listed in Table 29.1.1. Although usually a direct drug effect, CNS depression is occasionally secondary to hypoglycaemia, hypoxia or hypotension. Common causes of agitation or delirium following overdose are listed in Table 29.1.3. Toxic seizures are potentially life-threatening, and important causes are listed in Table 29.1.4.
Table 29.1.3 Toxic causes of agitation or delirium
Alcohol |
Anticholinergic syndrome |
Antidepressants |
Atypical antipsychotic agents |
Benzodiazepines and other sedative-hypnotics |
Cannabis |
Hallucinogenic agents |
Serotonin syndrome |
Sympathomimetic syndrome |
Theophylline |
Withdrawal syndromes |
Table 29.1.4 Toxic causes of seizures
Amphetamines |
Bupropion |
Carbamazepine |
Chloroquine |
Cocaine |
Isoniazid |
Mefanamic acid |
Theophylline |
Tramadol |
Tricyclic antidepressants |
Venlafaxine |
Hypothermia is usually a complication of environmental exposure secondary to a decreased level of consciousness or altered behaviour. Hyperthermia is a direct toxic effect and causes are listed in Table 29.1.5. Severe hyperthermia is rapidly lethal if not corrected.
Table 29.1.5 Toxic causes of hyperthermia
Amphetamines |
Anticholinergics |
Cocaine |
MAO inhibitors |
Salicylates |
Serotonin syndrome |
Pulmonary aspiration frequently complicates a period of decreased level of consciousness or a seizure. It is a leading cause of in-hospital morbidity and mortality following overdose. This complication is characterized by rapid onset of dyspnoea, cough, fever, wheeze and cyanosis.
Assessment
Risk assessment
A risk assessment should be made as soon as possible in the management of the poisoned patient. Only resuscitation is a greater priority (see Table 29.1.6). Risk assessment is a distinct quantitative cognitive step through which the clinician attempts to predict the likely clinical course and potential complications for the individual patient at that particular presentation.4 An accurate risk assessment allows informed decision-making in regard to all subsequent management steps including duration and intensity of supportive care and monitoring, screening and specialized testing, decontamination, enhanced elimination, antidotes and disposition. Factors that are taken into account when formulating this risk assessment include: the agent(s), the dose, the time since ingestion, the clinical features present and patient factors (Table 29.1.6). Specialized testing may refine risk assessment. Access to specialized poisons information in the form of a poisons information centre or in-house databases is often necessary to formulate an accurate risk assessment.
Table 29.1.6 Risk assessment-based approach to poisoning
Supportive care and monitoring |
Investigations |
Decontamination |
Enhanced elimination |
Antidotes |
Disposition |
Reproduced from Toxicology Handbook. Murray L, Daly F, Little M, Cadogan M. Elsevier, Sydney 2007.
Physical examination
The focused physical examination of the poisoned patient aims to:
Several toxic autonomic syndromes, or ‘toxidromes’, have been described in relation to poisoning. The principal ones are listed in Table 29.1.7. Identification of these syndromes may narrow the differential diagnosis in cases of unknown poisoning.5
Table 29.1.7 Toxic autonomic syndromes or ‘toxidromes’
Toxidrome | Features | Common causes |
---|---|---|
Anticholinergic | Agitated delirium | Antihistamines |
Tachycardia | Benztropine | |
Hyperthermia | Carbamazepine | |
Dilated pupils | Phenothiazines | |
Dry flushed skin | Plant poisonings | |
Urinary retention | Tricyclic antidepressants | |
Ileus | ||
Mixed cholinergic | Brady- or tachycardia | Organophosphates |
Hypo- or hypertension | Carbamates | |
Miosis or mydriasis | ||
Sweating | ||
Increased bronchial secretion | ||
Gastrointestinal hyperactivity | ||
Muscle weakness | ||
Fasciculations | ||
Mixed α- and β-adrenergic | Hypertension | Amphetamines |
Tachycardia | Cocaine | |
Mydriasis | ||
Agitation | ||
β-Adrenergic | Hypotension | Caffeine |
Tachycardia | Salbutamol | |
Hypokalaemia | Theophylline | |
Hyperglycaemia | ||
Serotonin | Altered mental status | Amphetamines |
Autonomic dysfunction | Antihistamines | |
Fever | Monoamine oxidase inhibitors | |
Hypertension | NSSRIs | |
Sweating | SSRIs | |
Tachycardia | Tricyclic antidepressants (Usually combined overdose) | |
Motor dysfunction | ||
Hyperreflexia | ||
Hypertonia (esp. lower limbs) | ||
Myoclonus |
NSSRI, non-selective serotonin re-uptake inhibitor; SSRI, selective serotonin re-uptake inhibitor.
Poisons information
Information on the clinical course and toxic doses of specific pharmaceutical and non-pharmaceutical poisons is available on a 24 h basis throughout Australia by telephoning 131126. The poison information centres are staffed by pharmacists and are also able to refer cases to clinical toxicologists for consultation.
Treatment
The management of poisoning should be approached in a systematic way. Following initial resuscitation, further treatment is informed by the risk assessment (Table 29.1.6).
Resuscitation, supportive care and monitoring
Supportive care is the key element in the management of poisoning. The vast majority of poisonings result in temporary dysfunction of one or more of the body systems. If appropriate support of the system in question is instituted in a timely fashion and continued until the toxic substance is metabolized or excreted, a good outcome can be anticipated. In severe poisonings supportive care may be very aggressive, and possible interventions are listed in Table 29.1.8.
Table 29.1.8 Supportive care measures for the poisoned patient
Airway | Endotracheal intubation |
Breathing | Supplemental oxygen |
Ventilation | |
Circulation | Intravenous fluids |
Inotropes | |
Antihypertensives | |
Antiarrhythmics | |
Defibrillation/cardioversion | |
Cardiac pacing | |
Cardiopulmonary bypass | |
Metabolic | Hypertonic dextrose |
Hypertonic saline | |
Insulin/dextrose | |
Calcium salts | |
Sodium bicarbonate | |
Agitation/delirium | Benzodiazepines |
Butyrophenones | |
Seizures | Benzodiazepines |
Barbiturates | |
Body temperature | External rewarming |
External cooling | |
Impaired renal function | Rehydration |
Haemodialysis |
Toxic hypertension rarely requires specific therapy. Most cases are mild and simple observation is sufficient. Agitation or delirium is a feature of many intoxications associated with hypertension, and adequate sedation with benzodiazepines usually lowers the blood pressure. Severe toxic hypertension is most likely in toxicity from cocaine or amphetamine-type drugs, and treatment may be indicated to avoid complications such as cardiac failure or intracerebral haemorrhage. The drug of choice in this situation is sodium nitroprusside by intravenous infusion. The extremely short duration of action of this vasodilator allows accurate control of hypertension during the toxic phase, and avoids the development of hypotension once toxicity begins to wear off.
Decontamination
Gastric emptying can be attempted by the administration of an emetic, most commonly syrup of ipecac, or by gastric lavage. In volunteer studies both of these techniques removed highly variable amounts of marker substances from the stomach even if performed immediately after ingestion, and the effect diminished rapidly with time to the point of being negligible after one hour.6,7 Clinical outcome trials have failed to demonstrate improved outcome as a result of routine gastric emptying in addition to administration of activated charcoal, except, perhaps, in patients presenting unconscious within 1 h of ingestion.8–10
The principal adsorbent available to clinicians is activated charcoal (AC), which effectively binds most pharmaceuticals and chemicals, and is currently the decontamination method of choice for most poisonings. Materials that do not bind well to charcoal are listed in Table 29.1.9.
Table 29.1.9 Materials that do not bind well to activated charcoal
Alcohols |
Corrosives |
Hydrocarbons |
Metals and their salts |
Volunteer studies demonstrate that the effect of AC diminishes rapidly with time and that the greatest benefit occurs if it is administered within 1 h. There is as yet no evidence that AC improves clinical outcome.11
There is no evidence to suggest that the addition of a cathartic such as sorbitol to AC improves clinical outcome.12,13
Apart from rarely employed endoscopic and surgical techniques, whole-bowel irrigation (WBI) is the most aggressive form of gastrointestinal decontamination. Polyethylene glycol solution (Golytely™) is administered via a nasogastric tube at a rate of 2 L/h until a clear rectal effluent is produced. This usually takes about 6 h and requires one-to-one nursing. In volunteer studies, this technique reduced the absorption of slow-release pharmaceuticals and so may be of benefit in life-threatening overdoses of these agents.13,14 Again, clinical benefit has not yet been conclusively demonstrated.15 The use of WBI has also been reported in the management of potentially toxic ingestions of iron, lead and packets of illicit drugs. Whole-bowel irrigation is contraindicated if there is evidence of ileus or bowel obstruction, and in patients who have an unprotected airway or haemodynamic compromise.16
Enhanced elimination
A number of techniques are available to enhance the elimination of toxins from the body. Their use is rarely indicated, as only a very few drugs capable of causing severe poisoning have pharmacokinetic parameters that render them amenable to these techniques (Table 29.1.10).
Table 29.1.10 Techniques of enhanced elimination
Technique | Suitable toxin |
---|---|
Repeat-dose activated charcoal | Carbamazepine |
Dapsone | |
Phenobarbitone | |
Phenytoin | |
Salicylate | |
Theophylline | |
Urinary alkalinization | Phenobarbitone |
Salicylate | |
Haemodialysis | Ethylene glycol |
Lithium | |
Methanol | |
Salicylate | |
Theophylline | |
Haemoperfusion | Theophylline |
Repeat-dose AC (25–50 g every 3–4 h) may enhance drug elimination by interrupting the enterohepatic circulation or by ‘gastrointestinal dialysis’. Gastrointestinal dialysis is the movement of a toxin across the gastrointestinal wall from the circulation into the gut down a concentration gradient that is maintained by charcoal binding. For this technique to be effective, a drug must undergo considerable enterohepatic circulation or, in the case of ‘gastrointestinal dialysis’, have a small volume of distribution, small molecular weight, low protein binding, slow endogenous elimination and bind to charcoal.16,17 The advantages of this technique are that it is non-invasive and simple to carry out.
Alkalinization of the urine enhances urinary excretion of drugs that are filtered at the glomerulus and are unable to be reabsorbed across the tubular epithelium when in an ionized form at alkaline pH. For elimination to be effectively enhanced by this method, the drug must be predominantly eliminated by the kidneys in the unchanged form, have a low pKa, be distributed mainly to the extracellular fluid compartment and be minimally protein bound.18
Antidotes
Very few drugs have effective antidotes. Occasionally, however, timely use of an antidote may be life saving or substantially reduce morbidity, time in hospital or resource requirements. Antidotes that may be indicated in the ED setting are listed in Table 29.1.11. However, it must be remembered that antidotes are also drugs, and are frequently associated with adverse effects of their own. An antidote should only be used where a specific indication exists, and then only at the correct dose, by the correct route, and with appropriate monitoring. Because many antidotes are so infrequently used, obtaining sufficient supplies when the need arises can be difficult. Every ED must review its stocking of antidotes and have a plan for obtaining further supplies should the need arise.
Table 29.1.11 Useful emergency antidotes
Poisoning | Antidote |
---|---|
Atropine | Physostigmine |
Benzodiazepines | Flumazenil |
Cyanide | Dicobalt edetate, hydroxocobalamin |
Digoxin | Digoxin-specific Fab fragments |
Insulin | Dextrose |
Iron | Desferoxamine |
Isoniazid | Pyridoxine |
Methaemoglobinaemia | Methylene blue |
Methanol and ethylene glycol | Ethanol, fomepizole |
Organophosphates and carbamates | Atropine, oximes |
Opioids | Naloxone |
Paracetamol | N-acetyl cysteine |
Sulphonylureas | Dextrose, octreotide |
Tricyclic antidepressants | Sodium bicarbonate |
Warfarin, brodifacoum | Vitamin K |
Clinical investigation
Measurement of serum drug concentrations is only useful if this provides important diagnostic or prognostic information, or assists in planning management. Some drug levels that may be useful are listed in Table 29.1.12. For most cases, drug overdose management is guided by clinical findings and not by drug levels. Some drugs commonly taken in overdose for which serum concentrations are of no value in planning management are listed in Table 29.1.13.
Table 29.1.12 Drug levels that may be helpful in the management of selected cases of overdose
Carbamazepine |
Digoxin |
Dilantin |
Lithium |
Iron |
Paracetamol |
Phenobarbitone |
Salicylate |
Theophylline |
Valproate |
Table 29.1.13 Drug levels that are not helpful in the management of overdose
CNS drugs | Cardiovascular drugs |
---|---|
Antidepressants | ACE inhibitors |
Benzodiazepines | Beta-blockers |
Benztropine | Calcium channel blockers |
Cocaine | Clonidine |
Newer antipsychotics | |
Opiates | |
Phenothiazines |
Disposition
Both the medical and the psychiatric disposition of the overdose patient must be considered. A good risk assessment is essential to determining timely and safe disposition.
1 Hawton K, Fagg J. Trends in deliberate self-poisoning and self-injury in Oxford. British Medical Journal. 1992;304:1409-1411.
2 McGrath J. A survey of deliberate self-poisoning. Medical Journal of Australia. 1989;150:317-322.
3 Pond SM. Prescription for poisoning. Medical Journal of Australia. 1995;162:174-175.
4 Murray L, Daly F, Little M, Cadogan M, editors. Toxicology handbook. Sydney: Elsevier, 2007.
5 Kulig K. Initial management of ingestion of toxic substances. New England Journal of Medicine. 1992;326:1677.
6 Krenzelok EP, McGuigan M, Lheureux P. Position statement: ipecac syrup. American Academy of Clinical Toxicology; European Association of Poisons Centres and Clinical Toxicologists. Journal of Toxicology – Clinical Toxicology. 1997;35(7):699-709.
7 Vale JA. Position statement: gastric lavage. American Academy of Clinical Toxicology; European Association of Poisons Centres and Clinical Toxicologists. Journal of Toxicology – Clinical Toxicology. 1997;35(7):711-719.
8 Kulig K, Bar-Or D, Kantrill SV, et al. Management of acutely poisoned patients without gastric emptying. Annals of Emergency Medicine. 1990;14:562-567.
9 Merigian KS, Woodard M, Hedges JR, et al. Prospective evaluation of gastric emptying in the self-poisoned patient. American Journal of Emergency Medicine. 1990;8:479-483.
10 Pond SM, Lewis-Driver DJ, Williams G, et al. Gastric emptying in acute overdose: a prospective randomised controlled trial. Medical Journal of Australia. 1995;163:345-349.
11 Chyka PA, Seger D. Position statement: single-dose activated charcoal. American Academy of Clinical Toxicology; European Association of Poisons Centres and Clinical Toxicologists. Journal of Toxicology – Clinical Toxicology. 1997;35(7):721-741.
12 Barceloux D, McGuigan M, Hartigan-Go K. Position statement: cathartics. American Academy of Clinical Toxicology; European Association of Poisons Centres and Clinical Toxicologists. Journal of Toxicology – Clinical Toxicology. 1997;35(7):743-752.
13 Kirshenbaum LA, Mathew SC, Sitar DS, et al. Whole-bowel irrigation versus activated charcoal in sorbitol for the ingestion of modified-release pharmaceuticals. Clinical Pharmacology Therapy. 1989;46:264-271.
14 Smith SW, Ling LJ, Halstenson CE. Whole-bowel irrigation as a treatment for acute lithium overdose. Annals of Emergency Medicine. 1991;20:536-539.
15 Tenenbein M. Position statement: whole bowel irrigation. American Academy of Clinical Toxicology; European Association of Poisons Centres and Clinical Toxicologists. Journal of Toxicology – Clinical Toxicology. 1997;35(7):753-756.
16 Pond SM. Role of repeated oral doses of activated charcoal in clinical toxicology. Medical Toxicology. 1986;1:3-11.
17 Chyka PA. Multiple-dose activated charcoal and enhancement of systemic drug clearance: summary of studies in animals and human volunteers. Clinical Toxicology. 1995;33:399-405.
18 Winchester JF. Active methods for detoxification. In Haddad LM, Shannon MW, Winchester JF, editors: Clinical management of poisoning and drug overdose, 3rd edn, Philadelphia: WB Saunders, 1998.
19 Whyte IM, Dawson AH, Buckley NA, et al. A model for the management of self-poisoning. Medical Journal of Australia. 1997;167:142-146.
20 Lee V, Kerr JF, Braitberg G, et al. Impact of a toxicology service on a metropolitan teaching hospital. Emergency Medicine. 2001;13:37-42.
29.2 Cardiovascular drugs
Pharmacokinetics
Standard CCB preparations are rapidly absorbed from the gastrointestinal tract, with onset of action occurring within 30 min.1 Pharmacokinetic parameters are shown in Table 29.2.1. Verapamil and diltiazem undergo significant first-pass hepatic clearance. Verapamil is metabolized to norverapamil, which possesses 15–20% of verapamil’s pharmacological activity and is renally excreted. Diltiazem is metabolized to deacetyldiltiazem, which has half the potency of the parent compound and undergoes biliary excretion. The elimination half-lives of all CCBs may be prolonged following massive overdose. Amlodipine has a longer plasma half-life (30–50 h) than other CCBs.
Absorption of β-blockers is rapid, with peak clinical effects occurring within 1–4 h. Pharmacokinetic parameters of the principal β-blockers are detailed in Table 29.2.2. Agents with high lipid solubility, such as propranolol, penetrate the blood–brain barrier better than the water-soluble agents, and hence cause greater central nervous system (CNS) toxicity.
Pathophysiology
CCBs antagonize the entry of extracellular calcium into cardiac and smooth muscle, but not skeletal muscle. Upon entry into cells, calcium participates in mechanical, electrical and biochemical reactions. It is involved in excitation–contraction of cardiac and smooth muscles, as well as phase 0 depolarization in the sinus and atrioventricular (AV) nodes by calcium influx through channels.2 CCBs affect myocardial contractility and slow conduction through the sinus and AV nodes. Contraction of smooth muscle is mediated by calcium influx, which is inhibited by CCBs. This results in vasodilatation and secondary reflex tachycardia from an increase in sympathetic activity.
The different classes of CCB have somewhat different pharmacological and toxic effects, as a consequence of their different binding characteristics to the dihydropyridine (DHP) receptors. Verapamil, a phenylalkylamine, produces more profound cardiac conduction defects and equal reductions in systemic vascular resistance when compared with other CCBs on a mg/kg basis.1 Verapamil is more likely to produce symptomatic decreases in blood pressure, heart rate and cardiac output than diltiazem, a benzothiazepine. The DHPs, which include amlodipine, felodipine, lercanidipine and nifedipine preferentially bind to vascular smooth muscle and predominantly decrease systemic and coronary vascular resistance. With the exception of felodipine, they also produce a reflex tachycardia by the unloading of baroreceptors.
The different β-blockers have slightly differing pharmacological properties, including selectivity for β adrenoreceptors, intrinsic sympathomimetic activity and membrane-stabilizing activity. The relative affinity for β adrenoreceptors may influence expression of toxicity. Atenolol, esmolol and metoprolol are β1-selective agents, and therapeutic use of these drugs is less likely to produce the peripheral vasoconstriction, bronchospasm and disturbances in glucose homoeostasis that result from β2 inhibition. However, pharmacological specificity decreases with increasing dose.3 Several β-blockers have partial agonist activity such that, although they block the β receptor to catecholamines, they also weakly stimulate the receptor. This partial agonist activity may have a protective effect in overdose.
Clinical features
Calcium channel blockers
The severity of toxicity is determined by a number of factors, including the amount and characteristics of the drug ingested, the underlying health of the patient, co-ingestants and delay until treatment. The majority of serious cases result from the ingestion of verapamil or diltiazem, the most toxic of the CCBs. Elderly patients and those with congestive cardiac failure are more prone to develop CCB poisoning. The principal clinical features are shown in Table 29.2.3. Ingestion of toxic amounts of standard preparations typically produces symptoms within 2 h, although maximal toxicity may not occur for up to 6–8 h. The slow-release preparations can produce significant toxicity, with onset of symptoms more than 6 h post ingestion. The major threats to life are myocardial depression and hypotension. Nifedipine produces tachycardia with normal blood pressure during the first 30 min, followed later by hypotension and bradycardia in large ingestions (>10 mg/kg). With verapamil and diltiazem poisoning, nausea, vomiting, hyperglycaemia and metabolic acidosis can develop. All CCBs can cause symptoms of cerebral hypoperfusion, such as syncope, lethargy, lightheadedness, dizziness, altered mental status, seizures and coma.
Table 29.2.3 Clinical features of CCB overdose
Central nervous system |
Gastrointestinal |
Cardiovascular |
Metabolic |
β-blockers
In one large series of patients with β-blocker overdose, 30–40% of patients remained asymptomatic and only 20% developed severe toxicity.4 Toxicity is more likely to develop after ingestion of propranolol, in patients with pre-existing cardiac disease or where there is co-ingestion of other drugs with effects on the cardiovascular system, especially CCBs and cyclic antidepressants.4,5 If β-blocker toxicity is to develop, it is usually observed within 6 h of ingestion.5,6
Treatment
Oral-activated charcoal should be administered as soon as practicable to all patients presenting within 2–4 h of ingestion, and to all those presenting after ingestion of slow-release preparations. More aggressive decontamination, with whole-bowel irrigation, is indicated following overdose with slow-release CCBs.7
A number of drugs play a role in the management of significant CCB or β-blocker poisoning, although none is a completely effective antidote. Suggested doses are shown in Table 29.2.4.
Table 29.2.4 Useful drugs in the management of CCB and β-blocker toxicity
CCBs | β-Blockers | |
---|---|---|
Calcium | 0.5–1 g (5–10 mLs) calcium chloride or 1–2 g (10–20 mLs) calcium gluconate i.v. over 5–10 minutes. Repeat every 10–15 minutes as required. Further administration guided by serum calcium concentrations. | |
Catecholamines | Adrenaline (epinephrine) infusion started at 1 μg/kg/min and titrate to maintain organ perfusion. | Isoprenaline or adrenaline (epinephrine) infusion titrated to maintain organ perfusion. |
Glucagon | A bolus dose of 5–10 mg followed by an infusion of 1–5 mg/h. | A bolus dose of 5–10 mg followed by an infusion of 1–5 mg/h. |
Hyperinsulinaemia euglycaemia | Actrapid 1 U/kg i.v. bolus followed by 0.5–1 U/kg/hr infusion. Give with 50% dextrose 50 mL followed by infusion to maintain euglycaemia. | Actrapid 1 U/kg i.v. bolus followed by 0.5–1 U/kg/hr infusion. Give with 50% dextrose 50 mL followed by infusion to maintain euglycaemia. |
Calcium, an inotropic agent, is the initial drug of choice for CCB toxicity. Administration must be closely monitored, with ionized calcium measured 30 min after commencing the infusion, and then second-hourly.8 Catecholamines are useful in attempting to restore adequate tissue perfusion.
Glucagon, a polypeptide hormone of pancreatic origin, enhances myocardial performance by increasing intracellular cAMP concentrations. This increase in cAMP triggers the release of cAMP-dependent protein kinase, which activates the calcium channels, causing an increase in heart rate and myocardial contractility. It works independently from that of the β-adrenoreceptor stimulation of the heart. Use of glucagon is supported only by case reports and some animal studies. There are no clinical trials supporting its efficacy in either calcium channel or beta-blocker poisoning and its role in management of these poisoning is questioned.9 It is frequently difficult to source adequate stocks of glucagon for use as an inotropic agent.
Hyperinsulinaemic euglycaemia therapy (HIET) is increasingly advocated as therapy for hypotension unresponsive to fluids, calcium salts and inotropes. This therapy is supported by animal work10,11 and promising initial human case reports12 but again clinical trials are lacking. Insulin administration switches cardiac cell metabolism from fatty acids to carbohydrates. It restores calcium fluxes and improves myocardial contractility. The recommended initial dose of actrapid is 1 U/kg i.v. followed by an infusion of 0.5–1 U/kg/h. This should be accompanied by an initial bolus dose of 50 mL 50% dextrose followed by an infusion to maintain euglycaemia.13
Severe propranolol toxicity is usually due to sodium channel blockade and treatment as for tricyclic antidepressant poisoning, including intubation, ventilation and sodium bicarbonate, is appropriate.
There are no clinically effective methods of enhancing the elimination of CCBs or β-blockers.
Clinical features
Two distinct clinical presentations of digoxin toxicity are observed: acute and chronic. Both are characterized by cardiac arrhythmias, and virtually all types of arrhythmia have been reported in the context of digoxin toxicity.14
Clinical investigation
In acute poisoning the serum potassium rises as Na-K ATPase function is progressively impaired. Hyperkalaemia denotes significant acute digoxin toxicity. Prior to the availability of a specific antidote for digoxin poisoning, a serum potassium concentration >5.5 mEq/L was associated with a high probability of lethal outcome.16 Hyperkalaemia is not usually observed in chronic digoxin toxicity. In fact, these patients are frequently hypokalaemic and hypomagnesaemic secondary to chronic diuretic use. Both these electrolyte disorders are important as they exacerbate digoxin toxicity.
Treatment
The best outcome is associated with early recognition of digoxin toxicity.
For chronic toxicity with minimal symptoms, management may involve no more than observation, cessation of digoxin administration, correction of hypokalaemia and hypomagnesaemia and appropriate management of any factors that contributed to the development of toxicity. However, presence of any cardiovascular system effects, particularly in elderly patients, is an indication for the administration of Fab fragments of digoxin-specific antibodies. The potential lethality of chronic digoxin poisoning is often underestimated with the result that digoxin antibody fragments are inappropriately withheld.16 From a purely economic view, the reduction in length of stay as a result of treatment with digoxin-specific antibodies outweighs the expense of the therapy.17
The specific antidote to digoxin poisoning is Fab fragments of digoxin-specific antibodies, which should be administered as soon as possible in any potentially life-threatening digoxin intoxication. Commonly accepted indications for the administration of Fab fragments are listed in Table 29.2.5.
Table 29.2.5 Indications for administration of Fab fragments of digoxin-specific antibodies following acute overdose
Hyperkalaemia (K > 5.0 mmol/L) associated with digoxin toxicity |
History of ingestion of more than 10 mg of digoxin |
Haemodynamically unstable cardiac arrhythmia |
Cardiac arrest from digoxin toxicity |
Serum digoxin concentration greater than 15 nmol/L |
Fab fragments of digoxin-specific antibodies
The extraordinary clinical efficacy of digoxin-specific fragments has been well documented in a multi-centre study.17 The same study also demonstrated the safety of the product, with the only adverse reactions reported being hypokalaemia (4% incidence) and worsening of congestive cardiac failure (3%).
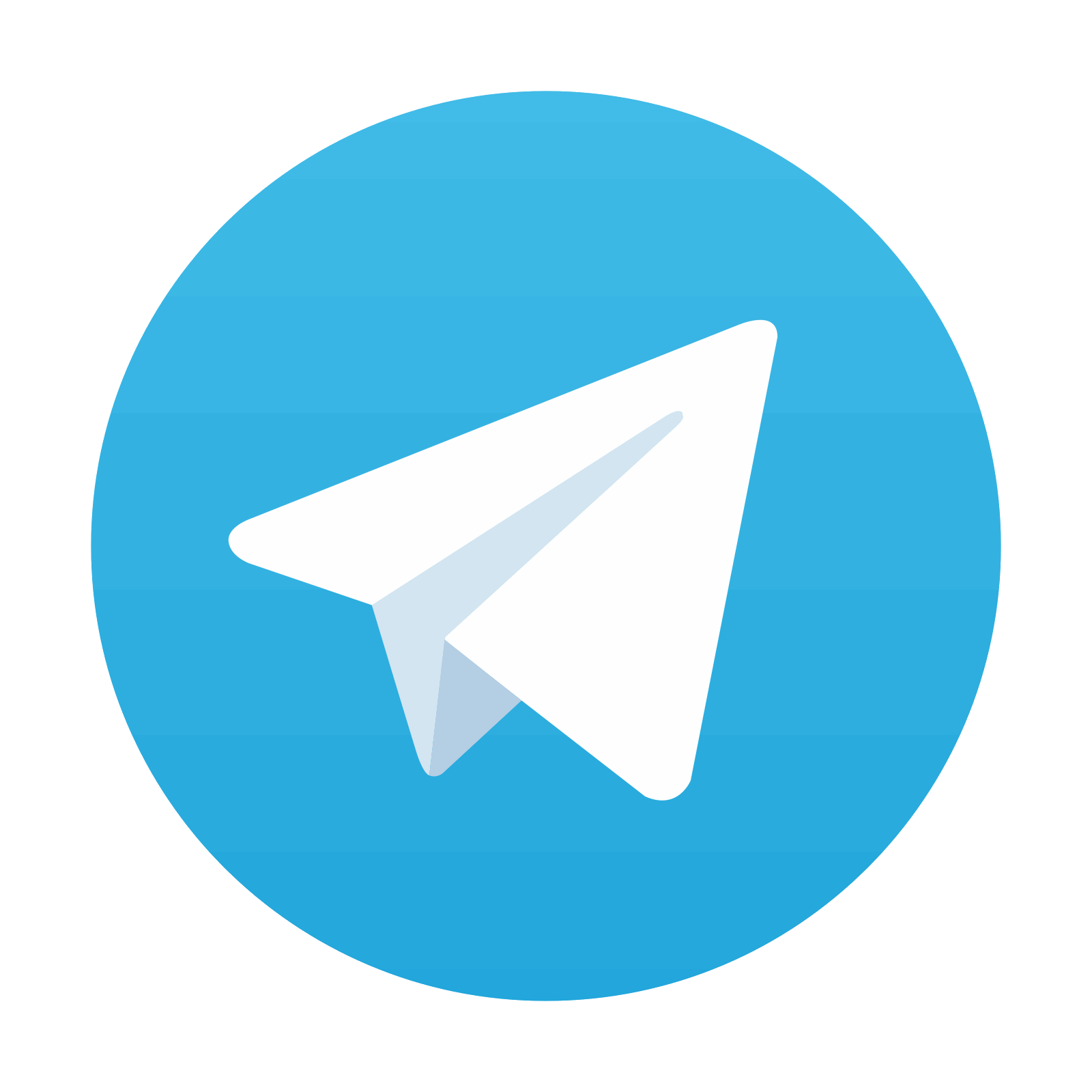
Stay updated, free articles. Join our Telegram channel

Full access? Get Clinical Tree
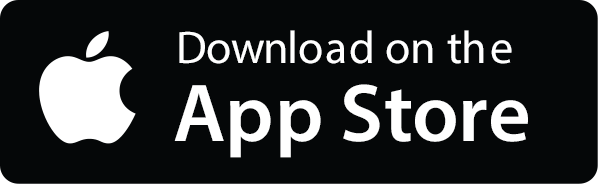
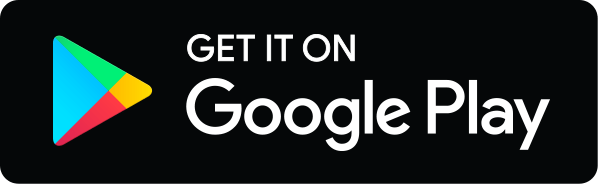