Section 28 Environmental
28.1 Heat-related illness
Epidemiology and pathophysiology
The other, more serious, heat-related disorders are all associated with hyperthermia, which if not treated promptly results in similar pathophysiology at a cellular and organ system level. A core body temperature greater than 41°C results in progressive denaturing of a number of vital cellular proteins, failure of vital energy-producing processes and loss of cell membrane function. At a cellular level the exact mechanisms leading to loss of cell membrane function and cell death in heat illness remain uncertain. At an organ system level these changes may manifest as rhabdomyolysis, acute pulmonary oedema, disseminated intravascular coagulation, cardiovascular dysfunction, electrolyte disturbance, renal failure, liver failure and permanent neurological damage.1 Any or all of these complications must be expected in severe heat illness.
Heatstroke shares some aetiological similarities with heat exhaustion but the hallmark of heatstroke is failure of the hypothalamic thermostat, leading to hyperthermia and the associated additional pathophysiological features described above. Clinically, heatstroke can be divided into ‘exertional heatstroke’ due to exercise in a thermally stressful environment, and ‘classic heatstroke’, which occurs in patients with impaired thermostatic mechanisms. Common risk factors for heatstroke are listed in Table 28.1.1.
Table 28.1.1 Heatstroke risk factors
Behavioural |
---|
Drugs |
---|
Illness |
---|
The elevation of central nervous system serotonin in serotonin toxicity is usually associated with combinations of serotoninergically active drugs, taken either therapeutically or in overdose. The incidence of serotonin toxicity when such combinations are taken is not known, but is low, and there is much individual variation in susceptibility.2 The syndrome is rarely precipitated by a single serotoninergic agent. Drugs associated with the serotonin syndrome are listed in Table 28.1.2. The most commonly implicated combinations are MAOI with SSRI, MAOI with tricyclics, and MAOI with pethidine.
Table 28.1.2 Drugs causing severe serotonin toxicity
Antidepressants |
---|
Analgesics |
---|
Antiparkinsonian agents |
---|
OTC preparations |
---|
Recreational drugs |
---|
Neuroleptic malignant syndrome (NMS) is a rare idiosyncratic reaction to neuroleptic agents with an incidence of between 0.02% and 3.0%, depending on the diagnostic criteria used. It occurs in response to a single agent, usually at therapeutic dosage. In individuals, the occurrence may be dose-related. Certain at-risk groups have been identified and are listed in Table 28.1.3.
Table 28.1.3 Risk factors for neuroleptic malignant syndrome
Patient factors |
---|
Drug dosing factors |
---|
NB: Duration of drug exposure and toxic overdose are not related to risk of developing NMS.
Clinical features
Heatstroke
The classic clinical features of heatstroke are neurological dysfunction, core temperature above 41°C and hot, dry skin. However, relying on this classic triad to make the diagnosis will result in a number of cases being missed. Loss of consciousness is a constant feature of heatstroke,1 but by the time of ED presentation conscious state may be improving, although some neurological abnormality will persist. Temperature readings may be misleadingly low, due either to effective pre-hospital care or to measurements at inappropriate sites, such as the oral cavity or axilla. Profuse sweating is a common feature.1 Other clinical features may include tachycardia, hyperventilation, seizures, vomiting and hypotension.
Serotonin toxicity
Serotonin syndrome is characterized by CNS, autonomic and motor dysfunction (Table 28.1.4). It develops after a latent period, which is normally a few hours but may be as long as several days.4 The spectrum of illness produced is broad. Most patients are only mildly affected and may escape clinical detection. Only the most serious develop hyperthermia severe enough to produce the complications of rhabdomyolysis, disseminated intravascular coagulation and renal failure. Most cases will resolve within 24–48 h once the precipitating agents are withdrawn. Even in severe cases, the underlying biochemical abnormality rapidly improves. The morbidity and mortality in these cases is caused by the complications that develop while the syndrome is active.
Table 28.1.4 Features of the serotonin toxicity
Central nervous system |
---|
Motor |
---|
Autonomic |
---|
Neuroleptic malignant syndrome
This syndrome manifests in patients who have recently been started on neuroleptic treatment, or in whom the dose of a neuroleptic agent has been increased. It has also been reported in patients in whom a dopaminergic agent has been rapidly withdrawn (e.g. in parkinsonism). There is a latent period of several days. Characteristically, there are four classic signs: fever, rigidity, altered mental state and autonomic instability. In practice, it may be difficult to distinguish clinically from serotonin toxicity unless a good drug history is obtained. As in serotonin toxicity, the spectrum of severity may be very broad, with only the more severe cases developing hyperthermia and its complications.5
Clinical investigation
All other heat disorders warrant a far more extensive laboratory and radiological work-up, as multiorgan system dysfunction is the rule.6 Tests should include an ECG (electrocardiograph), serum electrolytes, arterial blood gases, disseminated intravascular coagulation (DIC) screen, liver function tests, muscle enzyme assays, renal function and urinalysis, serum glucose and a chest X-ray.
Treatment
Heatstroke
This is a true medical emergency. Early recognition and aggressive therapy in the field and in hospital can prevent substantial morbidity and mortality. The key management is aggressive cooling. Cooling rates of at least 0.1°C/min should be achievable. Several cooling methods have been proposed, including evaporative cooling, iced water immersion, ice slush, cool water immersion, iced peritoneal lavage and pharmacological methods.7 A combination of methods is most widely used in EDs. All of the patient’s clothing should be removed and the patient sprayed with a fine mist of tepid water while gentle fanning is commenced (a ceiling fan is ideal). At the same time, areas with vascular beds close to the surface (neck, axillae and groins) should be packed with ice bags. This technique facilitates patient access and monitoring when compared to methods such as ice-bath immersion even though an iced bath may offer more rapid cooling.
Serotonin toxicity
Treatment of the drug-related hyperthermia involves both specific pharmacological therapy and full supportive and cooling measures, as described above. The objective is to recognize and treat before serious complications occur. In mild cases of the serotonin syndrome, benzodiazepines may be all that is required while awaiting spontaneous resolution. In severe cases, neuromuscular paralysis should be considered early, especially in cases of altered mental state. Specific antiserotoninergic drugs that can be used include chlorpromazine (12.5–50 mg i.m./i.v.),8,9 and cyproheptadine (4 mg orally 4-hourly, max. 20 mg in 24 h).2
Neuroleptic malignant syndrome
Again early recognition and full supportive care, combined with specific therapy, is the mainstay of treatment. Dopamine agonists such as bromocriptine may reduce the duration of the syndrome that, in contrast to serotonin syndrome, takes several days to resolve spontaneously. It can be administered orally or by nasogastric tube at an initial dose of 2.5–10 mg tds.3
Prognosis and disposition
In heatstroke both the maximum core temperature and the duration of temperature elevation are predictors of outcome. Prolonged coma and oliguric renal failure are poor prognostic signs.1 Mortality is still of the order of 10%, but most survivors will not suffer long-term sequelae.1 Any patient with suspected heatstroke should routinely be referred to the intensive care unit for ongoing care. Most cases of heat exhaustion and EAC will be suitable for short-stay ED treatment.
1 Shapiro Y, Seidman DS. Field and clinical observations of exertional heat stroke patients. Medicine and Science in Sports and Exercise. 1990;22:6-14.
2 Isbister GK, Buckley NA, Whyte IM. Serotonin toxicity: a practical approach to diagnosis and treatment. Medical Journal of Australia. 2007;187:361-365.
3 Heimann-Patterson TD. Neuroleptic malignant syndrome and malignant hyperthermia. Medical Clinics of North America. 1993;77:477-492.
4 Sternbach H. The serotonin syndrome. American Journal of Psychiatry. 1991;148:705-713.
5 Bristow MF, Kohen D. How malignant is the neuroleptic malignant syndrome? British Medical Journal. 1993;307:1223-1224.
6 Dematte JE, O’Mara K, Buescher J, et al. Near fatal heat stroke during the 1995 heat wave in Chicago. Annals of Internal Medicine. 1998;130:173-181.
7 Smith JE. Cooling methods used in the treatment of exertional heat illness. British Journal of Sports Medicine. 2005;39:503-507.
8 Gillman P. Successful treatment of serotonin syndrome with chlorpromazaine. Medical Journal of Australia. 1996;165:345.
9 Chan BSH, Graudins A, Whyte IM, et al. Serotonin syndrome resulting from drug interactions. Medical Journal of Australia. 1998;169:523-525.
28.2 Hypothermia
Epidemiology and pathophysiology
Hypothermia may occur in any setting or season.1 True environmental hypothermia occurring in a healthy patient in an adverse physical environment is less common in clinical practice than that secondary to an underlying disorder. Common precipitants include injury, systemic illness, drug overdose and immersion, and are outlined in more detail in Table 28.2.1. The elderly are at greater risk of hypothermia because of reduced metabolic heat production and impaired responses to a cold environment.2 Alcohol is a common aetiological factor and probably acts by a number of mechanisms, including cutaneous vasodilatation, altered behavioural responses, impaired shivering and hypothalamic dysfunction. Hypothermia in the ED setting is often associated with underlying infection.3
Table 28.2.1 Hypothermia aetiologies
Environmental | Cold, wet, windy ambient conditions |
Cold water immersion | |
Exhaustion | |
Trauma | Multitrauma (entrapment, resuscitation, head injury) |
Minor trauma and immobility (e.g. #NOF, #NOH) | |
Major burns | |
Drugs | Ethanol |
Sedatives (e.g. benzodiazepines) in overdose | |
Phenothiazines (impaired shivering) | |
Neurological | CVA |
Paraplegia | |
Parkinson’s disease | |
Endocrine | Hypoglycaemia |
Hypothyroidism | |
Hypoadrenalism | |
Systemic illness | Sepsis |
Malnutrition |
Clinical features
Despite substantial individual variations it is still possible to describe the typical patient in each category of hypothermia. The clinical manifestations of hypothermia also depend on the underlying aetiology and any associated features. To guide management in a field setting, where rectal temperature measurements are impractical or dangerous, moderate and severe hypothermia are often grouped together.4
Mild hypothermia manifests clinically as shivering, apathy, ataxia, dysarthria and tachycardia. Moderate hypothermia is typically marked by a loss of shivering, altered mental state, muscular rigidity, bradycardia and hypotension. In severe hypothermia signs of life may become almost undetectable, with coma, fixed and dilated pupils, areflexia and profound bradycardia and hypotension. The typical cardiac rhythm of severe hypothermia is slow atrial fibrillation. This may degenerate spontaneously, or with rough handling, into ventricular fibrillation or asystole.
Clinical investigation
Biochemical and haematological abnormalities are frequently associated with hypothermia,1 although there is no consistent pattern. Blood tests that are indicated include sodium, potassium, glucose, renal function, calcium, phosphate, magnesium, amylase, creatine kinase, ethanol, full blood count, clotting profile and arterial blood gases. Blood gas results should be accepted at face value, rather than adjusting for the patient’s temperature.5
A 12-lead electrocardiograph (ECG) and continuous ECG monitoring should be routine in moderate-to-severe hypothermia. The typical appearance is slow atrial fibrillation, with J or Osborn waves most prominent in leads II and V3–V6 (Fig. 28.2.1). The J wave is the extra positive deflection after the normal S wave, and is more obvious and more commonly seen with increasing severity of hypothermia.
Treatment
General
Magnesium may be the antiarrhythmic drug of choice in hypothermia.
The pharmacokinetics and dynamics of most drugs are substantially altered at low body core temperatures. Indeed, for many of the common drugs used in an ED they are unknown. Insulin is known to be inactive at <30°C. Hyperglycaemia, due in part to loss of insulin activity, is common in hypothermia, but should probably be managed expectantly until sufficient rewarming has occurred to ensure full endogenous insulin activity.
Rewarming therapies
Rewarming therapies in hypothermia have generated substantial debate. Unfortunately, there are only limited clinical trials on which to base recommendations. Although more invasive and rapid techniques are advocated for more severe hypothermia, there is little evidence to support this advice. The traditional concern of afterdrop (a paradoxical initial drop in core temperature with rewarming) is probably of little or no relevance in a clinical setting.6
Rewarming therapies are broadly divided into three groups: endogenous rewarming, which allows the body to rewarm by its own endogenous heat production; external exogenous rewarming, which supplies heat to the outside of the body; and core exogenous rewarming, which applies the heat centrally. The classification of the common rewarming therapies is outlined in Table 28.2.2.
Table 28.2.2 Rewarming therapy classification
Endogenous rewarming | Warm, dry, wind-free environment |
Warmed intravenous fluids | |
External exogenous rewarming | Hot bath immersion |
Forced-air blankets | |
Heat packs | |
Body-to-body contact | |
Core exogenous rewarming | Warmed, humidified inhalation |
Body cavity lavage | |
-peritoneal | |
-pleural | |
Extracorporeal |
In moderate hypothermia, endogenous heat production is likely to progressively fail and more aggressive exogenous rewarming therapies are indicated. Hot-bath immersion has the theoretical disadvantage of causing peripheral vasodilatation, with shunting of cool blood to the core and convective heat loss. This might be expected to increase core afterdrop and produce circulatory collapse. In fact, rewarming rates of at least 2.5°C/h with minimal afterdrop have been achieved using baths at 43°C.7 Nevertheless, substantial practical difficulties are obvious with monitoring a more seriously ill patient immersed in a bath. This method of rewarming can only be recommended for otherwise healthy patients who are expected to make a rapid recovery from accidental environmental hypothermia (e.g. immersion in very cold water).
The two therapies that have been best studied and are widely used in moderate hypothermia are forced-air rewarming and warm humidified inhalation.8 Forced-air rewarming is achieved by covering the patient with a blanket filled with air at 43°C. These devices direct a continuous current of air over the patient’s skin through a series of slits in the patient surface of the blanket. This method produces minimal, if any, afterdrop, is apparently without complication and, combined with warm humidified inhalation, should produce rewarming at about 2.5°C/h. The value of warm humidified inhalation is probably by preventing ongoing respiratory heat loss. Given its widespread availability and lack of complications, it seems reasonable to combine it with forced-air rewarming in moderate hypothermia.9 Body-to-body contact and chemical heat packs are often recommended as field treatments for all degrees of hypothermia. In mild hypothermia it seems that the benefit of any heat they deliver is negated by an inhibition of shivering thermogenesis. In more severe cases, where shivering is absent, it may be that even the small amount of exogenous heat they deliver is beneficial, but this remains unproven.
A suggested rewarming algorithm based on the evidence available to date is reproduced in Fig. 28.2.2.
Prognosis and disposition
Attempts at developing a valid outcome prediction model for hypothermia are likely to be frustrated by its multifactorial aetiology. Recovery with appropriate treatment is likely from accidental environmental hypothermia when there is no associated trauma. To date, the coldest patient to survive accidental hypothermia neurologically intact had an initial measured temperature of 13.7°C.10 Although increasing severity of hypothermia does worsen prognosis, the major determinant of outcome is the precipitating illness or injury. Reported mortality rates vary from 0 to 85%.
1 Danzl DF, Pozos RS, Auerbach PS, et al. Multicentre hypothermia survey. Annals of Emergency Medicine. 1987;16:1042-1055.
2 Weinberg AD. Hypothermia. Annals of Emergency Medicine. 1993;22:370-377.
3 Delaney KA, Vasallo SU, Larkin GL, Goldfrank LR. Rewarming rates in urban patients with hypothermia: prediction of underlying infection. Academic Emergency Medicine. 2006;13:913-921.
4 Forgey WM, editor. Wilderness Medical Society Practice Guidelines for Wilderness Emergency Care, 5th edn, Guilford: Globe Pequot, 2006.
5 Danzl DF. Accidental hypothermia. In Marx JA, et al, editors: Rosen’s emergency medicine: concepts and clinical practice, 6th edn, Philadelphia: Mosby, 2005.
6 Rogers IR. Which rewarming therapy in hypothermia? A review of the randomised trials. Emergency Medicine Australasia. 1997;9:213-220.
7 Hoskin RW, Melinshyn MG, Romet TT, Goode RC. Bath rewarming from immersion hypothermia. Journal of Applied Physiology. 1986;61:1518-1522.
8 Steele MT, Nelson MJ, Sessler DI, et al. Forced air speeds rewarming in accidental hypothermia. Annals of Emergency Medicine. 1996;27:479-484.
9 Giesbrecht GG. Emergency treatment of hypothermia. Emergency Medicine Australasia. 2001;13:9-16.
10 Gilbert M, Busund R, Skogseth A, et al. Resuscitation from accidental hypothermia of 13°C with circulatory arrest. Lancet. 2000;355:375-376.
11 Alzaga AG, Cerdan M, Varon J. Therapeutic hypothermia. Resuscitation. 2006;70:369-380.
12 Bernard SA, Gray TW, Buist M, et al. Treatment of comatose survivors of out-of-hospital cardiac arrest with induced hypothermia. New England Journal of Medicine. 2002;346:557-563.
13 The Hypothermia after Cardiac Arrest Study Group. Mild therapeutic hypothermia to improve neurologic outcome after cardiac arrest. New England Journal of Medicine. 2002;346:549-555.
28.3 Dysbarism
Introduction
This chapter focuses on medical problems that develop secondary to breathing gases at higher than normal atmospheric pressure (dysbarism). This usually occurs in the context of scuba (self-contained underwater breathing apparatus) diving, a popular recreational activity in Australasia. Diving is generally very safe, and serious decompression incidents occur approximately 1: 10 000 dives. However, because of a high participation rate, between 300 and 400 cases of decompression illness are treated in Australia each year.1 It is estimated that 10 times that number of divers experience less serious health problems after diving. Emergency physicians are often the first medical staff to assess the diver after a diving accident and it is essential they understanding the risks and potential injuries.
Diving physics and physiology
An understanding of pressure and some gas laws is essential to understand the pathophysiology of diving injuries. Absolute pressure at sea level is 1 atmosphere (ATA). Multiple units are used to measure pressure (Table 28.3.1). For every 10 m a diver descends in sea-water, the pressure increases by 1 ATA. This pressure change impacts on gas spaces within the body according to Boyle’s Law.
Table 28.3.1 Atmospheric pressure at sea level in various units
1 Atmosphere absolute (ATA) |
101.3 kPa (SI units) |
1.013 Bar |
10 m of sea water (MSW) |
760 mm of mercury (mmHg) |
14.7 pounds per square inch (PSI) |
where P = pressure, V = volume and k = constant.
The proportionate change in volume is greatest near the surface (Table 28.3.2).
Table 28.3.2 Depth vs pressure and gas volume (Boyle’s law)
Depth (m) | Absolute pressure (ATA) | Gas volume (%) |
---|---|---|
0 | 1 | 100 |
10 | 2 | 50 |
20 | 3 | 33 |
30 | 4 | 25 |
40 | 5 | 20 |
A diver breathing air at 40 m is inhaling a gas with a partial pressure of oxygen equivalent to breathing 100% oxygen at the surface. At partial pressures above 3 ATA, the PN2 affects coordination and judgement (‘nitrogen narcosis’). Oxygen may also become toxic at partial pressures greater than 1 ATA. Recreational scuba diving generally has a limit of 40 m because of these effects.
where Q = volume of gas dissolved in a liquid, k = constant and Pgas = partial pressure of the gas.
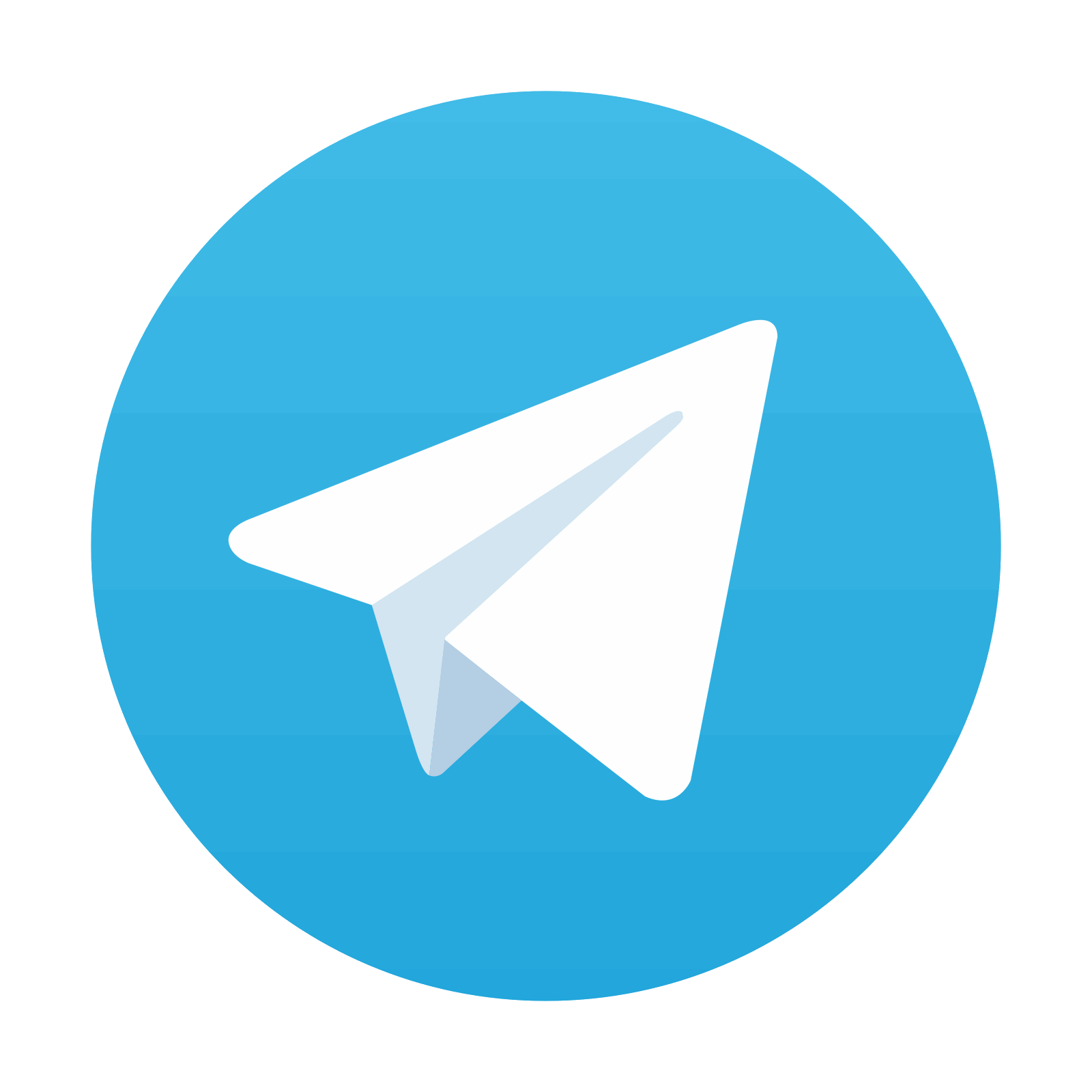
Stay updated, free articles. Join our Telegram channel

Full access? Get Clinical Tree
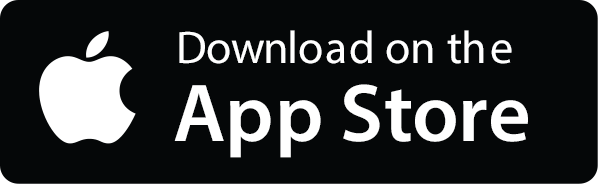
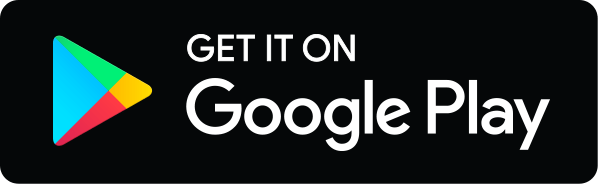