The care of the neonate or infant undergoing thoracic surgery presents the anesthesiologist with a unique set of challenges. Here, the disparate goals of mitigating the physiologic stress to patients who often have limited cardiopulmonary reserve confront the need for surgical exposure and highlight the limitations of our available equipment and technique.
An understanding of the fundamental physiology of ventilation and perfusion balance, both normal and in illness, is essential if the iatrogenic disease that is single lung ventilation is to be managed with minimal morbidity. The physiologic disturbances that result from lung isolation are, or course, superimposed on the pathophysiology that necessitated the surgery. Both open and minimally invasive approaches to the thoracic cavity have their own advantages and risks, familiarity with which can allow anticipation and avoidance of many intraoperative complications related to the anesthetic. Finally, no discussion of this topic would be complete without a survey of the surgical pathology of the chest in this vulnerable age group.
Respiratory Development and Physiology
The pulmonary system develops as a ventral outpouching of the foregut during the first postconceptual weeks. Subsequent stages of development are pseudoglandular (5–17 weeks), canalicular (16–25 weeks), saccular (24–36 weeks), and alveolar (>36 weeks) [1]. Of note, penetration of pulmonary capillary networks into terminal air sacs begins in the saccular stage, permitting some degree of gas exchange in the extremely premature neonate. Type II pneumocytes appear at about 24 weeks’ gestation and produce surfactant [2]. Surfactant is a mixture of phospholipids, primarily phosphatidylcholine, and proteins called SP-a through SP-d, that reduces surface tension of the alveolar wall. This reduced surface tension in smaller alveoli prevents complete collapse at functional residual capacity (FRC). Prenatal administration of steroids to the mother prior to delivery has been demonstrated to accelerate fetal lung maturity and reduce the incidence of respiratory distress syndrome, but a Cochrane review concluded that the optimal drug, dose, and regimen are still unclear [3]. Postnatal development largely consists of a gradual increase in the number of alveoli, from about 10–50 million to approximately 200–300 million during the first decade of life.
At birth, the dramatic changes involved in transition to extrauterine life center on the replacement of liquid-filled airways and placental gas exchange, by gas-filled airways and pulmonary capillary/alveolar gas exchange. Extrinsic chest compression during vaginal birth is thought to expel some of this fluid. Neonates born via Cesarean section, by comparison, have increased parenchymal lung water, and a higher incidence of transient tachypnea of the newborn (TTN). Recent data suggest that active transepithelial absorption via amiloride-sensitive sodium channels actually accounts for most of this difference [4]. The first breaths, with the generation of highly negative intrapleural pressure, help move intra-alveolar fluid into the lymphatic drainage of the lung. Mechanical compression and hypoxic vasoconstriction of the small intraparenchymal vessels are diminished. Pulmonary vascular resistance falls and pulmonary blood flow increases in response to the increasing alveolar and arterial pO2. The resulting increase in left atrial volume and pressure functionally closes the foramen ovale, usually within days. While anatomic closure follows in some weeks, up to 30 percent of adults will still be probe-patent. The ductus arteriosus similarly constricts in response to oxygen and falling levels of prostaglandins. In the term newborn, functional closure of the patent ductus arteriosus (PDA) usually occurs by day 2 of life, and anatomic closure within 2–3 weeks. Because all of these changes are physiologic rather than anatomic for several weeks, the neonate is particularly vulnerable to their reversal by hypoxemia, hypercarbia, acidosis, and hypothermia. Progressive systemic hypoxemia with elevated pulmonary artery pressures and subsequent right ventricular failure rapidly ensue and can quickly become unrecoverable.
Respiratory effort is seen in utero during the second trimester, and plays an essential role in normal lung development [5]. Nuclei in the medulla generate rhythmic stimulation modulated by baroreceptors located at the carotid body, and chemoreceptors at the brainstem level, as well as a host of other inputs. These signals can originate from the respiratory epithelium, the muscles of respiration, the limbic system or the cerebral cortex. Efferents flow via the phrenic nerve to the diaphragm, and through spinal nerves to the intercostals and strap muscles of the neck.
The mechanics of respiration are fundamentally different in the infant and neonate, primarily because of the markedly increased compliance of the chest wall. The ribs are cartilaginous and the accessory muscles of respiration are incompletely developed, and the cross-section of the thorax tends more toward the square than the rectangle, with a proportionally smaller AP diameter. In combination, these factors mean that the FRC is disproportionately smaller in the newborn than in older children. The chest wall offers less resistance to the elastic recoil of the lung, hence small airways can close even in normal tidal breathing. The diaphragm is normally a complete membrane by eight weeks’ gestation, but in the newborn has only 10–30 percent type 1, slow-twitch fibers compared to about 55 percent in the adult, rendering it more susceptible to fatigue. Type 2 fibers are now described as either fast-oxidative or fast-glycolytic based on myosin isoforms, and are recruited during tachypnea and respiratory distress. These fibers are also responsible for responsiveness to caffeine, based on the presence of RyR3, which is expressed only in diaphragmatic muscle [6]. The upper airway is also dependent on cyclic activation of the pharyngeal and supraglottic musculature to maintain patency; all of the mechanisms described are negatively impacted by most anesthetics. Oxygen consumption in the neonate (6–8 ml kg–1 min–1) is about twice that of the adult (3–4 ml kg–1 min–1) and further reduces the respiratory reserve of these patients. The inverse fourth relationship of Poiselle’s law also means that the work of breathing is increased disproportionately by the high resistance of the smaller airways throughout the tracheobronchial tree.
Since the classic work of West, ventilation and perfusion have been understood to each increase across gravitational gradients, minimizing mismatch [7]. Perfusion increases at a greater rate, hence V/Q ratio declines across the same gradient, whether it’s cranial/caudad in the upright patient, or “toward the floor” in the lateral decubitus position. In a surgical setting, this implies that the dependent, presumably “good” lung is functionally more important. This is indeed true in the adult. Under spontaneous breathing, the diaphragm also functions more efficiently under Starling’s law, because it is “loaded” or under stretch from the locally increased pressure of the abdominal contents. In the infant or child, in contrast, chest compressibility opposes the diaphragmatic loading, and the higher compliance of the chest wall reduces expiratory reserve and pushes FRC closer to residual volume. Even the awake patient may experience small airway closure during normal tidal breathing, promoting atelectasis of the dependent lung. General anesthesia, neuromuscular blockade and mechanical ventilation each have additive effects on decreasing FRC and impairing oxygenation.
The primary homeostatic mechanism that minimizes V/Q mismatch is hypoxic pulmonary vasoconstriction (HPV). While still poorly understood, this refers to the calcium-mediated, eNOS-modulated increase in regional pulmonary vascular resistance triggered by alveolar hypoxia, thus relatively diverting flow away from the nonventilated areas [8]. In comparison, systemic arterioles respond to hypoxia with dilatation; this is a major distinction between the two parallel circulations. Although its precise mechanism remains unclear, hypoxia triggers via several pathways a small rise in intracellular ionized calcium in pulmonary arterial smooth muscle cells. When persistent, animal models suggest that altered gene expression leads to myocyte hypersensitivity to endothelial thromboxane, which may be relevant in persistent pulmonary hypertension of the newborn [9]. This mechanism is believed to be inhibited by volatile agents, nitrates, and calcium channel blockers, although some studies have shown no effect of clinically relevant concentrations of isoflurane.
Thoracotomy
The first recorded chest operation dates to the Ebers papyrus, roughly 3600 years ago, while the modern era of thoracic surgery can be dated to 1909, when Lillienthal performed the first thoracotomy under positive pressure ventilation with a tracheal tube [10]. The epidemic of post-pneumonic empyema accompanying the 1917 influenza pandemic led to the first wave of rapid development in thoracic surgery by Graham and others [11]. Entry into the chest can be through an intercostal space, or through the bed of a subperiostially resected rib, and is typically described anatomically as anterior, lateral, axillary, or posterolateral [12]. The latter affords the widest exposure and operative flexibility, not surprisingly at the cost of greatest morbidity as it sections both the serratus anterior and the latissimus dorsi. Particularly in infants and children, this can lead to long-term problems with scoliosis and other deformities; muscle-sparing incisions are an effort to limit these [13]. Postoperative pain control can be challenging, even with effective regional or neuraxial techniques, and can lead to secondary complications from poor coughing and atelectasis. The incidence of chronic pain after thoracotomy approaches 60 percent at one year [14].
Thoracoscopy/VATS (Video-Assisted Thoracic Surgery)
Thoracoscopy was first performed in 1910 by Jacobeus in Sweden (using a cystoscope), and proved useful in the diagnosis and treatment of pleural disease, which at the time usually meant tuberculous effusion [15]. It took the technological explosion of instrumentation and imaging systems that was spurred by laparoscopic cholecystectomy to bring this into the mainstream, and allow its extension to parenchymal disease. As more complicated procedures were undertaken, including anatomic resections, the term VATS (for video-assisted thoracic surgery) was introduced, to replace the implication that “thoracoscopy” was a noninvasive medical procedure. As with laparoscopic surgery, the promise of smaller incisions, less postoperative pain, and faster recovery seemed self-evident, and led to widespread enthusiasm and adoption, more often than not without randomized controlled trials demonstrating superiority. In fact, a recent pilot randomized controlled trial from Great Ormond Street showed that compared to open surgery, VATS repair of congenital diaphragmatic hernia (CDH) and tracheoesophageal fistula (TEF) were associated with severe and prolonged hypercapnia and acidosis, raising questions about the safety of the practice [16]. Other reviews caution that the improved cosmetic and musculoskeletal benefits must be balanced against a potentially higher risk of complications, especially in young infants [17,18].
Single Lung Ventilation
From a surgical perspective, all intrapleural procedures would ideally be conducted with the operative lung collapsed and unmoving; that is, under single lung ventilation of the contralateral side. Prior to the mid-1990s almost all chest operations, especially in children, were performed through open thoracotomy. In patients under about ten years of age, this involved conventional tracheal intubation/ventilation with the surgeon retracting the inflated lung as necessary for exposure. As the trend toward minimally invasive surgery has moved forward, an increasing percentage of interventions are performed using VATS techniques. While conventional ventilation/retraction aided by CO2 insufflation is sometimes unavoidable, the technical performance of any procedure is vastly simplified by single lung ventilation. Similarly, the risk of lung injury (tear, intraparenchymal hemorrhage, or postoperative air leak) and uncontrolled bleeding is lessened. But single lung ventilation is an iatrogenic pneumothorax, hence by definition a pathologic state. The ability of an individual to tolerate this depends on the health of the nonoperative (dependent) lung, and their capacity to mitigate the V/Q mismatch triggered by the sudden increase in shunt.
Single lung ventilation is typically carried out with an FiO2 of 1, some degree of permissive hypercapnia, smaller tidal volumes, and higher respiratory rates. With a double lumen tube, the operative side is opened to atmosphere and/or a suction catheter can be passed to facilitate collapse. In VATS procedures, gentle CO2 insufflation can expedite this. If lung isolation is accomplished using a bronchial blocker as opposed to a double lumen endotracheal tube, effective collapse is more dependent on insufflation and absorption atelectasis.
If unexpected hypoxemia develops during single lung ventilation, bronchoscopy should be performed quickly to determine correct placement of the double lumen endotracheal tube or bronchial blocker. In particular, the complication of herniation of a bronchial cuff into the trachea must be immediately recognized and corrected. Continuous positive airway pressure (CPAP) to the nondependent lung is generally agreed to be the most effective next step, but cannot be accomplished if a bronchial blocker is in use since these typically have no functional internal lumen. The application of positive end-expiratory pressure (PEEP) to the dependent lung can also be effective, and is often added empirically. Communication with the surgical team should be ongoing. Isolation and occlusion of the main pulmonary artery either by digital pressure or temporary clamping can rapidly correct hypoxemia and may avoid the need for reinflation of the operative lung, but this is only easily performed in open procedures.
Techniques for Single Lung Ventilation
Mainstem Intubation
The earliest technique for single lung ventilation, still often used, is to selectively intubate the mainstem bronchus of the nonoperative side. Several techniques have been described. This can usually be accomplished by turning the head toward and elevating the shoulder of the opposite side after passing a conventional tube through the vocal cords. Use of a stylet precurved similarly to a double lumen tube shape, and placed with the same technique (see below), has also been reported. Since the standard endotracheal tube bevel faces left, when placing a conventional oral endotracheal tube into the right mainstem bronchus, ventilation of the right upper lobe bronchus is often not possible. Two proposed solutions are to cut the tip to a right-facing bevel, or leaving the original bevel and cutting a Murphy eye. To place the endotracheal tube into the left mainstem bronchus, some clinicians rotate the tube 180° to gain a favorable bevel orientation to promote left-sided advancement, and then rotate back when at depth to minimize risk of covering the left upper lobe orifice. Perhaps the simplest method is to place the tube in the trachea in the usual fashion, advance a bronchoscope into the mainstem of interest, and then advance the tube over the scope. Whatever method or device is used, position must be confirmed with auscultation and fiberoptic bronchoscopy, both immediately and after turning lateral. Finally, in an attempt to regain some of the distinct advantages of a double lumen tube, passing two smaller endotracheal tubes and directing one into each mainstem bronchus has been described, but has the disadvantage of the extra bulk at the glottis potentially causing pressure damage, and is not recommended. Additionally, the smaller diameter conventional tubes required for bronchial as opposed to tracheal placement in this method are of proportionately shorter length, leaving little tube length available at the mouth to secure.
Double Lumen Tubes
Conventional double lumen endotracheal tubes (e.g., Rusch Robert Shaw, Teleflex, Triangle Park, NC) are available down to size 26. While their use has been described in a six-year-old, it is more commonly avoided in patients less than 8–10 years or about 30 kg. These are almost always left-sided tubes; the very short right mainstem bronchus and correspondingly smaller tolerances make right-sided double lumen tubes difficult to use in pediatrics. Unlike in adults in whom pneumonectomy for malignancy may require stapling across the origin of a mainstem bronchus, this seldom presents a technical challenge to the surgeon, even operating on the left lung. They are placed similarly to adults: the tip of the tube is passed just through the vocal cords, the stylet is removed, the tube rotated 90° and then advanced. Usually this results in correct placement of the bronchial lumen in the left mainstem bronchus. The tracheal cuff is inflated and both lungs ventilated, after which the bronchial cuff can be inflated and each side tested clinically for chest rise and auscultation. Fiberoptic bronchoscopy should then be performed for confirmation. With the scope through the tracheal lumen, the blue bronchial cuff should be seen just at the origin of the left mainstem bronchus. Inspection should then be made through the bronchial lumen to be sure that the left upper lobe bronchus has access to the airway; i.e., that it has not been covered by the length of the tube between the bronchial cuff and the tip of the bronchial lumen. Double lumen tubes must be exchanged at the end of the case for conventional devices when continuation of ventilation postoperatively is planned. Newer designs include silicone DLTs (e.g., Sil-Broncho, Teleflex, Triangle Park, NC), which on insertion may be less traumatic and more resistant to balloon tears, but not yet available in smaller than 33 Fr.
Bronchial Blockers
In patients too small for double lumen tubes, an appealing alternative is the bronchial blocker. This was first described using a Fogarty embolectomy catheter (Edwards Life Sciences, Irvine, CA) passed through the cords outside of (and usually prior to) the endotracheal tube (ETT), advanced under bronchoscopic guidance into the operative mainstem [19]. Still used by some, they are manufactured with inflated balloon diameters of 4, 5, and 9 mm. Alternatively, in larger patients passing a guidewire through the scope and then exchanging the scope for a catheter, with or without fluoroscopy, has been described; the Fogarty Thru-Lumen (over-the-wire) embolectomy catheter is available with balloon sizes of 5, 9, and 11 mm diameter. While balloon-tipped PA catheters and atrial septostomy balloons have been used, neither has as small a catheter diameter or is as guidable as the Fogarty.
Released in 1999, the Arndt bronchial blocker (Cook Medical, Bloomington, IN) has gained popularity for its improved ease of use [20]. This device is available in 5, 7, and 9 Fr sizes, which can be placed through ETTs of at least 4.5, 6.5, and 7.5 mm respectively (using a 2.2 mm bronchoscope). A multiport adapter has a 15 mm connector on its side for the anesthesia circuit, an in-line diaphragm for a flexible scope, and an angled Tuohy-Borst adapter through which the catheter itself is passed. The tip of the catheter holds a wire guide loop, which can be cinched around the tip of the bronchoscope, either before connecting the adapter (preferred, if scope plus catheter fit together through the tube) or in the airway. This system has the distinct advantage of being able to continuously ventilate during the process, and giving more control over the direction taken by the catheter. After placement, the guide loop assembly is removed, leaving a small central lumen to which suction can be applied to expedite collapse.
The UniBlocker is a 5 Fr and the Fuggiano Blocker a 9 Fr device by LMA North America (San Diego, CA). These are conceptually similar to the Arndt Blocker described above.
Another device recently available is the Rusch EZ Blocker (Teleflex Medical, Research Triangle Park, NC), essentially a single catheter which bifurcates to short bronchial lengths, each with its own, independently inflatable balloon. Placed straddling the carina, the lung is allowed to passively deflate with exhalation, and the balloon is inflated on the surgical side prior to resuming ventilation. Particularly advantageous for bilateral procedures, it is manufactured in a single size, 7 Fr.
With any bronchial blocker, one of the main challenges is dislodgement. Balloon herniation into the distal trachea can acutely impede all ventilation, and can often be precipitated by surgical manipulation. The immediate response is to deflate the balloon and to assess and reposition as necessary under bronchoscopic guidance. Using saline rather than air to fill the blocker balloon makes it somewhat less likely to dislodge.
Univent
A Japanese product, the Univent (LMA North America, Inc., San Diego, CA) consists of a single lumen ETT with an integral balloon-tipped catheter that can be advanced independently into a mainstem bronchus using a malleable shaft for torque control. It is available in sizes 3.5–9 mm. Its main disadvantage is a comparatively large overall external diameter for a given internal tracheal lumen size. The blocking catheter does have a central lumen to allow aspiration of, as well as PEEP application to, the nonoperative lung.
Bronchoscopes
As essential to these procedures as the anesthesia workstation itself is the flexible fiberoptic bronchoscope. First developed in the 1970s, the smallest currently available instruments are 2.2 mm in diameter (e.g., BF-N20, Olympus, Tokyo, Japan). The smallest scopes available with a suction channel (of 1.2 mm) has a 2.8 mm outer diameter (e.g., BF-XP60, Olympus, Tokyo, Japan). While the increased number of optical fibers delivers a marginally better image, the suction channel is not surprisingly overwhelmed by anything beyond the smallest drops of fluid.
One important limitation of these devices is their inherent fragility and the associated expense of maintenance and replacement, especially in a teaching hospital.
Preoperative Assessment
Unlike in the adult or older child, where spirometry, peak flows, and flow-volume loops are routine and provide an objective assessment of many aspects of pulmonary function, no such testing is possible in the neonate and infant. History, physical exam, and selective imaging still provide the compass with which to navigate safely through a thoracic intervention in this population.
Gestational age and perinatal history should be considered, with particular regard to intubation, surfactant therapy, length of NICU stay if any, or apnea events. The timing of symptom onset, or prenatal diagnosis, as well as any prior interventions, especially previous thoracotomy, should be considered. In addition to review of chest radiographs, a cardiac echo is often a necessary part of pre-anesthetic assessment in these patients, particularly in the infant with airway anomalies or VACTERL association. In the older infant, the usual concern over possible recent upper respiratory tract infection or exposure to second-hand smoke is always present. While having blood available is advisable for all but the most minor thoracic procedures, sending a type and cross after line placement can avoid a difficult venipuncture in an awake infant. Some centers use type-specific irradiated blood without crossmatch in neonates when necessary. Prior to going to the OR, a thorough discussion must be held with the surgical team regarding the operative plan, extent of pulmonary resection anticipated, likelihood of major blood loss, and the degree to which single lung ventilation is important. The plan for post-op disposition must be understood but flexible.
General Considerations
Following standard inhalational induction of general anesthesia, with parental presence or premedication as indicated in older infants, and taking care to keep the infant warm, IV access adequate for resuscitation is obtained. This usually includes both upper and lower extremity sites. Arterial catheterization is indicated for most thoracotomies, particularly in longer and more complex procedures or in patients in whom postoperative ventilation is anticipated, where its availability for arterial blood gas sampling is invaluable. Central venous access is obtained if peripheral access is inadequate, inotrope use is considered likely, or if any other considerations make determination of mixed venous O2 saturation desirable. For any of these access procedures, ultrasound can be significantly time-saving, especially in the smallest patients, and is becoming the standard of care for placement of CVLs. Bladder and orogastric catheters are placed, and the patient is usually placed on an under-body forced-air warmer. Caution must be exercised and the device typically not operated at its high (43 °C) setting on these smallest patients, particularly if in direct contact with skin. The patient is then moved to the lateral decubitus position with appropriate padding and protection of the axilla, extremities, and ear; new-generation fluidized positioners (e.g., Z-FLOW, Sundance Solutions, White Plains, NY) can be extremely useful in this regard. The position of the airway management device is then rechecked with bronchoscopy.
If epidural placement has been planned, it is now undertaken, and used as an intraoperative adjunct as well as for post-op analgesia. In these vulnerable patients it is imperative that a second provider be present to assist in positioning and attend to the details of the anesthetic during placement of the epidural. In contrast, if a paravertebral catheter has been selected, it is often best placed at the end of the procedure, as it is practically impossible to keep out of the surgical field.
A balanced anesthetic is maintained with oxygen, opioid, and low-dose volatile anesthetics with muscle relaxation. The transition to single lung ventilation requires particular vigilance of both respiratory parameters and attention to the surgical field to assess efficacy. End-tidal CO2 values are known to be sometimes inaccurate in neonates due to circuit volume/Vt ratio and sampling rate factors; transcutaneous CO2 sensors have been developed and are under investigation (TCM CombiM, Radiometer Medical, Copenhagen, Denmark), but measurement of arterial blood gases is the gold standard to assess the adequacy of ventilation. Patients who arrive intubated, have significant comorbidities, or are less than a few months old are rarely extubated in the operating room.
Regional Anesthesia/Analgesia
Surgical breach of the thoracic cavity unavoidably results in tissue injury, which in turn leads to a local inflammatory response as well as activation of a systemic neurohumeral cascade. Nocioceptive afferents to the central nervous system trigger efferent autonomic activation and catecholamine release from the adrenal medulla. Alterations in hemodynamics, metabolism, and immune function follow, which have been associated with less favorable outcomes. Regional anesthesia combined with general anesthesia has been shown to more completely suppress this stress response compared to general anesthesia with opioids [21]. Neuraxial anesthesia has been shown to essentially eliminate the hyperglycemic response and relative insulin resistance following thoracic procedures in children. There are other potential advantages to the addition of a regional technique to the general anesthesia for these patients. Diminished NK cell activity is a marker for downregulated cellular immunity, and is associated with increased risk for metastasis in several human tumors. In animal models this has been directly observed following exposure to both opioids as well as volatile anesthetics. The latter have also been observed to increase hypoxia-induced factor (HIF-1alpha) in tumor cells, stimulating angiogenesis [22]. Effective regional anesthesia/analgesia allows the administration of lower dose opioid and inhaled anesthetic agents, potentially mitigating these untoward effects. In the pediatric population, perhaps most relevant is the growing body of literature concerning apoptosis and neurocognitive development risk. In a recent consensus conference, a panel of experts stressed the importance of developing strategies to limit potential brain injury from general anesthetics [23].
A recent study of 40 patients has shown that infants undergoing thoracic procedures have superior analgesia, earlier return to full feeds, and shorter ICU stays with perioperative regional analgesia [24]. Although complications from epidural placement in small babies are infrequent, the seriousness of the potential neurologic deficit is leading toward increasing use of paravertebral as opposed to epidural catheters in these patients.
Cystic Lesions of the Chest
Congenital Cystic Adenomatoid Malformation
Failure of normal embryologic development of the tracheobronchial budding from the primitive foregut can lead to a constellation of cystic lesions that have overlapping clinical and histologic findings. The term bronchopulmonary-foregut malformation has been proposed to encompass many of these.
The most common of these is the congenital cystic adenomatoid malformation (CCAM), with an incidence of about 1:30 000 births. First described by Ch’in and Tang in 1949, the term “congenital pulmonary airway malformation” has been used more recently [25,26]. Increasingly diagnosed by prenatal ultrasound, these are believed to represent the disorderly proliferation of terminal bronchioles without distal alveoli. Although some lesions are seen to involute on serial ultrasounds, postnatal CT scans will usually demonstrate their persistence, even with a normal chest radiograph. Congenital cystic adenomatoid malformations are more common on the left, are usually confined to a single lobe, and have an 18 percent rate of associated abnormalities such as cardiac anomalies and renal agenesis. Prenatally the differentiation between CCAM and pulmonary sequestration can be difficult, and indeed hybrid lesions have been confirmed at operation, where the typical histology of CCAM is accompanied by a systemic arterial supply. Other prenatal ultrasound findings suggestive of CCAM include polyhydramnios, pleural effusion, or hydrops fetalis. The X-ray or sonographic finding of multiple air/fluid levels in the inferior left chest can generate confusion with congenital diaphragmatic hernia.
Patients with CCAM can have a range of presentations, from being entirely asymptomatic at birth to having severe fetal compromise. Case reports exist of fetal lobectomy using EXIT, as well as thoracoamniotic shunting. Those born with minimal symptomatology will inevitably develop cough, respiratory distress, recurrent infection, or pneumothorax. Tube thoracostomy can be life-saving in extreme cases. Malignant degeneration can occur, with poorly differentiated blastoma in the younger cohort, and bronchoalveolar carcinoma in adolescents and adults. Surgical resection is most commonly performed between 3 and 12 months, with a trend toward earlier definitive treatment as infectious complications become more frequent after about seven months, and earlier removal offers greater potential for compensatory growth of the remaining pulmonary parenchyma. Anatomic lobectomy is the standard of care and provides excellent long-term results; lesser resections have significant rates of recurrence.
Pulmonary Sequestration
Another lesion of disordered pulmonary embryogenesis, the hallmark of pulmonary sequestration is the absence of a clear connection with the airway, and the presence of a systemic arterial supply. Whereas in normal development, more distal generations of bronchioles are closely coupled with development of the pulmonary arterial tree, sequestrations are felt to arise from continued alveolar development caudally, somehow stimulating a systemic arterial inflow [27]. This is consistent with the observation that they occur most commonly in the medial lower lobes, the left involved more than the right. Arterial supply is usually from a branch directly arising from the aorta and can be infradiaphragmatic in up to 20 percent of cases; venous drainage is much more variable and can be systemic, pulmonary, or both.
Sequestrations are described as intralobar (75 percent) or extralobar (25 percent) based on whether the lesion lies within the visceral pleura of the adjacent lung, or are covered by its own visceral pleura, respectively. Intralobar sequestrations usually present with recurrent respiratory infection or failure to thrive. While associated anomalies are uncommon, a small percentage will have a connection to the esophagus. In contrast, about 40 percent of extralobar sequestrations will have other anomalies, including cardiac, chest wall, and vertebral defects. They have even been described entirely below the diaphragm, especially in the left suprarenal area. Extralobar sequestrations have a lower incidence of infection, but can present with feeding difficulties, pain from torsion, or congestive failure.
Most authors recommend that all pulmonary sequestrations be surgically treated in the first year of life, once any infections complication has been controlled. Intralobar lesions are resected by wedge resection or lobectomy as appropriate. Extralobar sequestrations are usually resectable with minimal if any loss of normal lung tissue; the challenge is control of the systemic vascular supply. Magnetic resonance has replaced conventional angiography, but imaging is essential to preoperative planning. Gaining proximal arterial control in the abdomen can prevent exsanguination, should one of these typically thin-walled, tortuous, and high-flow vessels be injured. Patients presenting with heart failure are best served by preoperative angiography with embolization.
Bronchogenic and Dermoid Cysts
Conceptually, perhaps the simplest of these lesions is the bronchogenic cyst, which might be thought of as resulting from “exocytosis” from the bronchial tree. More common on the right, and in males, these are lined with respiratory epithelium and often show cartilage, goblet cells, and smooth muscle. In infants they usually present as mass lesions causing airway compromise with stridor, retractions, wheezing, or cyanosis. Older children more often develop infection as their initial symptom. These lesions should be resected when diagnosed because of the risk of infection as well as malignant degeneration. While not communicating with the airways, they are often densely adherent and technically challenging. If complete resection is not feasible, any residual mucosa should be cauterized in situ. Dermoid cysts are clinically indistinguishable, but show squamous rather than ciliated columnar epithelium [28].
For this and all of the congenital lesions, the primary anesthetic consideration is whether positive pressure ventilation is likely to exacerbate air trapping in the anomaly, leading to mediastinal shift, tension physiology, and resultant hemodynamic compromise. In general, CCAM, sequestrations, and duplication cysts do not significantly communicate with the airway and controlled ventilation presents no undue risk. If, however, the precise anatomic relationships of the anomaly are unknown, maintaining spontaneous respiration until the chest is open or single lung ventilation of the normal side is achieved is the most prudent course.
Congenital Lobar Emphysema
Congenital lobar emphysema (CLE) is an uncommon entity, first described by Nelson in 1932; it is characterized by the abnormal hyperinflation of otherwise normal lung parenchyma, classically in a lobar distribution [29]. CT angiography typically shows absence of arterial flow to the involved segments. The left upper lobe is the most common site, followed by the right upper and middle lobes. Involvement of the lower lobes is rare; this can be a useful distinction from CCAM. Its pathophysiology is produced by a functional one-way valve effect, which can be either extrinsic, usually thought to be from an abnormal vascular structure, or intrinsic due to bronchomalacia. Because of this, positive pressure ventilation can precipitate acute decompensation. Maintaining spontaneous breathing until the chest is open is preferred. Despite the “lobar” nomenclature, a recent series demonstrated that among 24 left upper lobe lesions, all but one in fact showed sparing of the two lingular segments; the authors advocated lung-sparing surgery and saw no recurrences in long-term follow-up [30].
Congenital Diaphragmatic Hernia
Congenital diaphragmatic hernia (CDH) is among the more common life-threatening thoracic anomalies, occurring in about 1:2500 live births. Progress in its management has been frustratingly slow, the discussion of which could occupy an entire chapter if not textbook. The first successful repair is credited to Heidenhain in Germany in 1905 [31]. Congenital diaphragmatic hernia is usually diagnosed with prenatal ultrasound in developed countries. Whether or not there is a prenatal diagnosis, the presentation at delivery usually includes respiratory distress, a scaphoid abdomen with absent breath sounds, and often the presence of bowel sounds on the left. The diaphragm, a uniquely mammalian structure, consists of a central tendon and peripheral muscular elements, now believed to be derived solely from the embryologic pleuroperitoneal fold. Normal development requires a complex interplay of differentiation, migration, and integration of neural and vascular elements. A defect in the amuscular mesenchymal component occurring as early as 3–4 weeks appears to inhibit normal migration of muscle cell precursors. Roughly one-third of cases will have other major anomalies, and over 70 genetic syndromes include CDH as part of their clinical criteria. Associated anomalies, especially cardiac, have been clearly associated with poorer outcomes. The vast majority of defects occur at the left-sided posterolateral foramen of Bochdalek, allowing herniation of abdominal contents into the chest after 10–12 weeks and subsequent mechanical interference with lung development. The resulting pulmonary hypoplasia and pulmonary hypertension are the most difficult physiologic insults to manage. While some reports have demonstrated improvement in mortality since the application of newer technologies such as extracorporeal membrane oxygenation (ECMO), high-frequency oscillatory ventilation (HFOV), inhaled nitric oxide (iNO), and PDE5 inhibitors, population-based studies still show roughly 50 percent mortality because of what has been termed the “hidden mortality” of selection bias and prenatal diagnosis leading to termination [32]. While right-sided Bochdalek hernias do occur, they tend to have less severe pulmonary disease as the liver relatively impedes bowel translocation into the thorax [33]. Bilateral hernias occur in <1 percent, are more frequently associated with other anomalies, and have a very high mortality. Defects can also occur in the anterior (retrosternal) foramen of Morgagni or rarely in the central tendon; these tend to present later, even into adult life, and often with bowel obstruction. Work with rodent models has revealed a critical role of the retinoic acid pathway and its genetic control early in gestation interfering with normal development of the pleuroperitoneal fold and its muscular mesenchymal component [34]. Curiously, the timing of teratogen exposure seems to affect laterality of the condition [35].
Because the defect occurs early in gestation, and overall treatment results are suboptimal, prenatal intervention is intuitively appealing. Progressive models in rats, rabbits, and sheep showed that tracheal occlusion appears to induce lung growth and decrease the resulting degree of hypoplasia, because the lung is a net producer of amniotic fluid. These efforts have evolved to a percutaneous balloon technique called FETO (fetal endoscopic tracheal occlusion) [36]. While several studies have shown no clear improvement, Ruano et al. have published a prospective randomized controlled trial of FETO vs. conventional therapy for fetuses with severe isolated CDH, in which mortality was 4.8 percent in the treatment group vs. 50 percent with standard therapy [37]. While some experts still consider it an option in the most severe cases, its use has declined. It has been increasingly appreciated that the pathophysiology of this condition involves not only the grossly hypoplastic lung, but the contralateral “normal” lung as well. The more global nature of this anomaly is also reinforced by reports indicating that survivors have a higher incidence of recurrent respiratory infections, GI reflux disease, skeletal anomalies, hearing loss, and neurocognitive delay [38].
Although described in postmortem exams by Riverius in 1679, surgical treatment did not become commonplace until 1940, when Ladd and Gross reported 16 operated cases with 9 survivors [39]. Gross went on to report a successful repair on the first day of life in 1946, and the concept that early relief of pressure on the lung and heart by the abdominal viscera would improve outcomes stimulated the trend toward immediate postnatal repair. Only since the late 1980s, with a growing understanding that the pulmonary hypoplasia and hypertension are the critical physiologic derangements, has surgical repair of CDH evolved from an emergency procedure to a delayed, often scheduled one, today. After intubation and nasogastric decompression in the delivery room, invasive lines are placed for monitoring acid–base status and nutritional support. Strategies for lung protective ventilation and permissive hypercapnia are employed in the NICU. Even with lung protective ventilation, the occurrence of a pneumothorax is still a possibility. In addition to the ventilation strategy, PGE-1 is commonly employed to maintain ductal patency and unload the right ventricle. Echocardiography is obtained early to rule out cardiac anomalies and repeated often to assess the right ventricular/systemic pressure relationship. After a period of stabilization sometimes lasting up to weeks (for infants on ECMO), repair is undertaken either transabdominally (more commonly open) or thoracoscopically. The patient is brought to and returned from the operating room intubated. Continuing the use of the NICU-type ventilator for both transport and surgery is considered advantageous over bag-mask ventilating and use of the anesthesia machine, even if the child is not on HFOV. This approach mandates an intravenous anesthetic technique. Primary repair is preferred, while prosthetic patch repair is required for larger defects. Prostanoids and iNO are continued if in use, with inotropic support immediately available if needed. Use of a chest drain is controversial, but often employed.
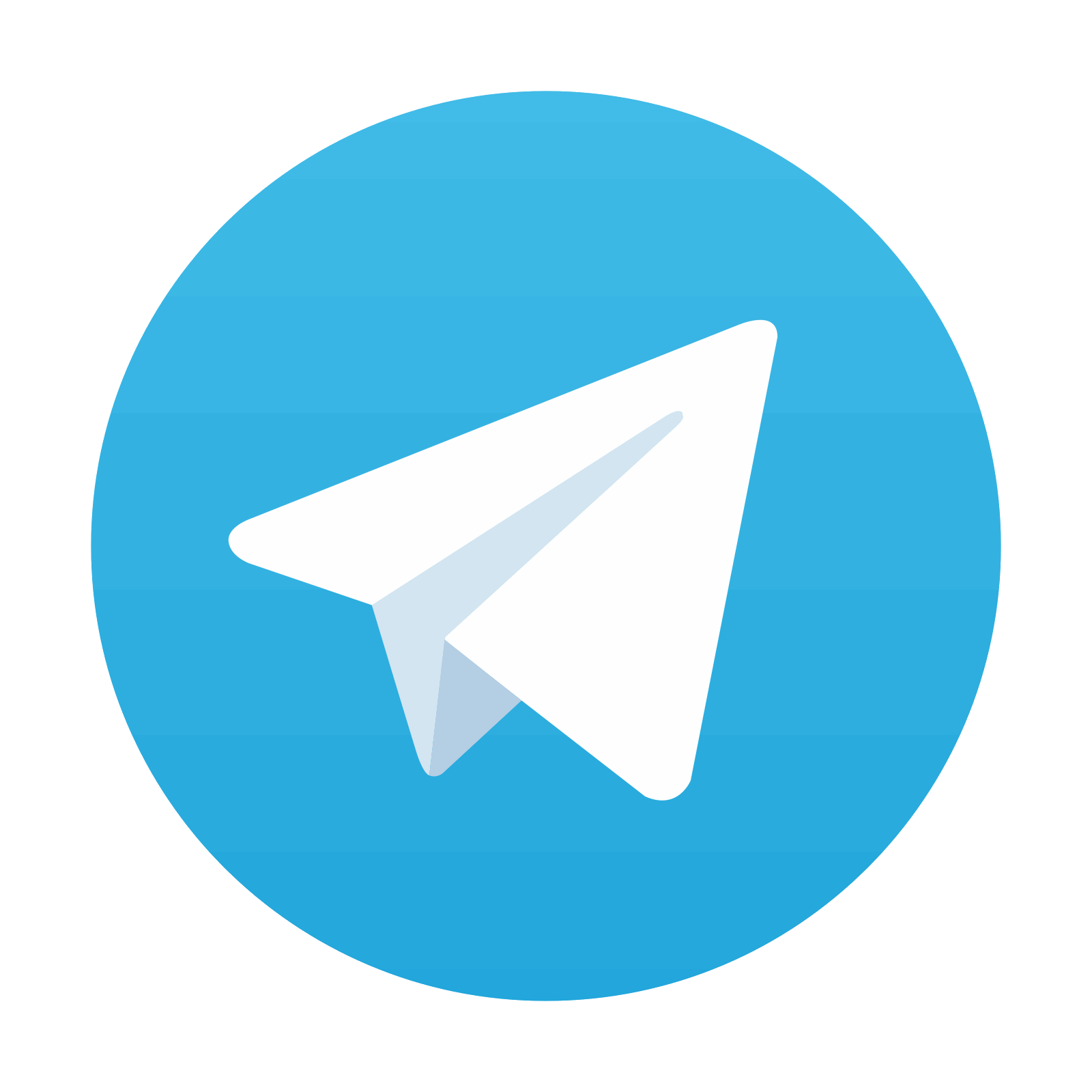
Stay updated, free articles. Join our Telegram channel

Full access? Get Clinical Tree
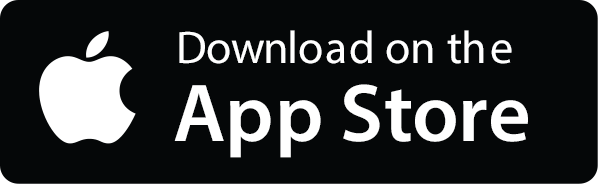
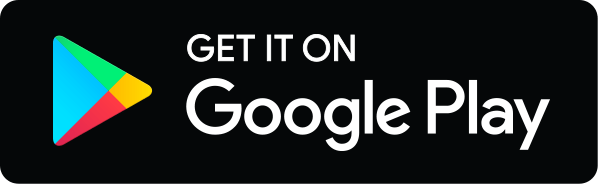