Introduction
The central nervous system (CNS) is a complicated network of neurons that integrates a myriad of sensory input from both inside and outside the body and effects motor responses. The CNS consists of neurons, oligodendrites, and support cells such as microglia. Abnormal CNS development can result in devastating injury to young children, making the administration of general anesthesia very challenging. In order to successfully care for children undergoing neurosurgical procedures, it is essential to understand the interaction between the disordered CNS and the anesthetic milieu. In this chapter we review the general principles of anesthetic management of infants and neonates undergoing neurosurgical procedures. The purpose of this chapter is not to give recipes for treating infants and neonates for various neurosurgical procedures. Rather, we hope to give the reader an understanding of the variety of anesthetic considerations when caring for babies that are neurosurgical patients.
Development of the Central Nervous System
Many of the neurosurgical conditions seen in infants occur because of abnormal fetal development. Thus a basic understanding of the embryologic development of the nervous system is helpful to comprehend the variety of neurologic conditions that affect young infants and neonates.
Beginning of the Nervous System
During the third week of fetal development, the ectoderm differentiates into the neuroplate. This neuroplate folds upon itself to form the neural groove. There are two neural folds which form along each side of the neural groove. Neurulation begins at the fourth week of development, when the neural folds fuse dorsally to create the neural tube. The spinal ganglia, cranial nerves, and components of the peripheral and autonomic nervous system are derived from neural crest cells which are located adjacent to the neural folds. The neural tube fuses in a central to caudal and central to cranial direction, creating the neural canal. The small holes remaining at each end of the neural tube fuse at days 25 and 27 days concomitantly with the development of the vascular supply to the neural tube. The ventricular system and central canal of the CNS develops from the neural canal, with the walls of the neural tube forming the brain and spinal cord.
Development of the Spinal Cord
The spinal cord develops from the caudal end of the neural tube, with the neural canal becoming the central canal at about ten weeks’ gestation. The cells of the neural tube differentiate into two different layers; the ependymal layer which becomes neurons, astrocytes, and oligodendrocytes, and the marginal layer which becomes the white matter as axons grow into this area from spinal cord neurons. The sulcus limitans is a longitudinal groove which forms on each side of the fetal spinal cord. This groove forms a separation between the dorsal or afferent portion of the spinal cord from the ventral or efferent portion of the spinal cord. The mesenchymal cells surrounding the protospinal cord differentiate into the dura mater, pia mater, and arachnoid mater. These meninges become fluid filled as cerebrospinal fluid (CSF) is produced during the fifth week of development, which forms the subarachnoid space.
As the spinal cord is developing, the cranial portion of the neural tube undergoes rapid growth and turning, with the neural canal forming the cranial ventricular canal. It is believed by many authors that normal development of the ventricular canal system is dependent of the caudal closure of the neural tube, which causes an increase in intraluminal pressure which stimulates brain growth and ventricular enlargement. This is one possible explanation for the finding that infants with open neural tube defects of the spinal cord, such as meningomyeloceles, very often have associated abnormalities of their brain and ventricular system.
Physiology
Intracranial Contents
After infancy the cranial vault should be considered a closed space that is normally occupied by three components: the brain and interstitial fluid comprising 80 percent of the volume; the CSF comprising 10 percent of the volume; and the blood comprising 10 percent of the total volume. The Monro-Kellie hypothesis, which states that the sum of all intracranial components are constant, necessitates that an increase in the volume of one component demands a decrease in volume of one or two of the remaining components in children in whom the sutures and fontanelles are closed. Infants with their unfused sutures and open fontanelles can tolerate slow increases in intracranial volumes [1]. Thus a child with an increase in head circumference should be evaluated for a space-occupying brain lesion or hydrocephalus even if there are no signs of increased intracranial pressure. Rapid increases in the volume of CSF or growth of a space-occupying lesion such as a brain tumor even in a young infant will overwhelm the infant’s ability to increase the size of his cranial volume, leading to brain herniation as a result of acute elevations of intracranial pressure (ICP).
Intracranial Pressure
The normal ICP in children is <15 mmHg and < 6mmHg in infants. The normal ICP for premature infants is not known. In infants with open fontanelles, the ICP may remain normal even in the setting of significant intracranial pathology. Careful physical examination should always include an examination of the fontanelles. Full or bulging fontanelles can be a sign of intracranial disease. Often the first sign of intracranial disease in an infant is a normal steady state ICP with occasional ICP pressure waves. These pressure waves should never be considered normal.
Other infants will manifest an elevation of ICP despite open fontanelles. These elevations of ICP can cause either cerebral ischemia and/or brain herniation leading to secondary brain injury. Elevations of the ICP without concomitant elevations of mean arterial pressure (MAP) will result in a decrease in cerebral perfusion pressure (CPP), which can result in cerebral ischemia.
With a decrease in CPP, cerebral blood flow (CBF) decreases. This results in decreased delivery of metabolic substrates and oxygen. Cell damage then occurs, which leads to cell death and an increase in inflammatory mediators, a cascade causing further increases in extracellular water and exacerbating the elevation in ICP. As CPP falls, neuronal dysfunction and ultimately cell death occur [1].
In addition to decreasing cerebral perfusion pressure, elevations of ICP can lead to brain herniation. This occurs when intracranial contents herniate from one compartment into another, leading to further brain ischemia and injury. Transtentorial herniation occurs when the temporal lobe displaces into the infratentorial space. Infants will show signs of hemiparesis, pupillary dilation, and eventual loss of consciousness. This is a medical emergency that will lead to death if the condition is not expeditiously relieved. The clinical signs of elevated ICP are less reliable in infants and children. Infants may exhibit signs of papilledema, hypertension, and bradycardia, but have normal ICP. Or infants with elevated ICP may show no clinical signs at all [2,3]. Classically, young children with chronically elevated ICP will have headaches, irritability, decreased oral intake, and morning emesis without papilledema [4]. Late signs include alterations in the level of consciousness, with children exhibiting abnormal responses to painful stimuli [2]. Depending on the level of obtundation, general anesthesia or sedation may be needed to evaluate these patients. Imaging studies are most commonly done, such as cranial X-rays, computed tomography (CT), and magnetic resonance imaging (MRI). These studies often reveal a decrease or obliteration of the lateral ventricles, hydrocephalus, midline shift, and/or obliteration of the third and fourth ventricles.
Cerebrospinal Fluid
Cerebrospinal fluid is produced by the choroid plexus at the rate of 0.35 ml per minute in both infants and adults [5,6], resulting in approximately 500 ml produced per day in adults [5]. However, the total volume of CSF in the subarachnoid space in adults is only 100–150 ml, meaning that the CSF is replaced several times during the day. The overall volume of CSF in infants and children is much less than in adults because the subarachnoid space is much smaller. Cerebrospinal fluid production is only marginally affected by elevated ICP.
Arachnoid villi within the subarachnoid space absorb the CSF, which enters the venous system via one-way valves between the subarachnoid space and the sagittal sinus. There is also a limited amount of reabsorption that occurs through the ependymal lining of the ventricles. The rate of reabsorption increases with elevation of ICP. Obstruction of the arachnoid villi or obstruction within the ventricular system can lead to decreased absorption. Pathologic processes such as inflammation, infection, intracranial hemorrhage, congenital malformations, and CSF absorption and ICP elevations [2].
Intracranial Compliance
It is important to estimate the amount of intracranial compliance that an infant or child has before administering a general anesthetic. Intracranial compliance is defined as the ratio of change of ICP over change of intracranial volume. In infants who have greater abilities to compensate for increases in cranial volumes because of their open fontanelles, it is difficult to assess the degree of additional compensation available if needed for general anesthesia. Once the ICP is elevated, it is reasonable to assume that compensatory mechanisms have been overridden. But infants with normal ICP may be at high risk for brain injury with even the small increases in cranial volumes/CBF that may occur with certain anesthetic techniques. Assessment of the fontanelles to determine whether the fontanelle is full in infants suspected of having intracranial pathology is essential before administering a general anesthetic. Although infants have higher compliance than adults because of their sutures and open fontanelles, children have lower compliance than adults. This is thought to be due to a higher ratio of brain water content, less CSF volume, and a higher ratio of brain content to intracranial capacity [1]. Infants with fused cranial sutures are at higher risk than normal infants for developing ICP elevations.
Cerebral Blood Volume and Cerebral Blood Flow
The cranial compartment most easily manipulated by anesthesiologists is the cerebral blood volume (CBV), though this compartment only represents 10 percent of the total volume of the cranial contents. Most of the CBF by volume is contained in the low-pressure, high-capacitance venous system. Initially increases in intracranial volume are met with decreases in CBV. Often, venous blood shifts from intracranial to extracranial, as seen in infants with hydrocephalus who develop distended scalp veins [7].
In a normal adult, the CBF is approximately 55 ml per 100 g of brain tissue per minute, which represents approximately 15 percent of the adult cardiac output [8–10]. The CBF is almost double in children, at approximately 100 ml per 100 g of brain tissue per minute, which represents about 25 percent of cardiac output [11,12]. Infants and young children in general have a larger portion of their cardiac output devoted to cerebral perfusion than do adults. Neonates and premature infants appear to have a lower CBF at about 40 ml per 100 g per minute than any other age group. The reasons for this are unclear. There is also greater variability of CBF in neonates and premature infants depending on the physical state of the infant. Cerebral blood flow is decreased during sleep and feeding states [12,13].
Cerebral blood flow in the nonanesthetized state is usually very closely coupled with cerebral metabolic rate for oxygen (CMRO2), which is known as cerebral autoregulation. In adults, CMRO2 is approximately 3.5–4.5 ml O2 per 100 g per minute; in children it is higher, but in premature infants it is lower [11]. Most general anesthetics will depress the CMRO2 by as much as 50 percent, but in very young premature infants these same general anesthetics can be excitotoxic, leading to an increase in CMRO2 [14]. Inadequate CBF will lead to conditions that result in acidosis, such as hypoxemia, hypercarbia, and ischemia. These conditions increase the hydrogen ion concentration within the cerebral blood vessels, which will cause cerebral dilation and thereby increase CBF and CBV. When there is a loss of coupling between CMRO2 and CBF, cerebral autoregulation is impaired. CBF then becomes pressure passive, meaning it varies with the system MAP.
Cerebrovascular Autoregulation
Effects of Blood Pressure
Cerebrovascular autoregulation refers to the ability of the brain to have a relatively constant CBF within a MAP range. This range is believed to be between 50 and 150 mmHg in older children and adults. In infants the lower limit of autoregulation is unknown, but studies have shown that there is a decrease in CBF when the MAP in infants less than six months of age is less than 40 mmHg. Cerebral autoregulation can be diminished or abolished by a variety of physiologic disturbances, including extreme prematurity, acidosis, sepsis, cerebral edema, tumors, various medications, and vascular anomalies.
Although the absolute lower limits of autoregulation are undefined in premature and term infants, and are likely to vary within that population, most neonatal intensive care units try to maintain the MAPs in mmHg of their patients as equal to or higher than the gestational age in weeks of the infant. It should be assumed that in critically ill infants there is a loss of cerebral autoregulation and systemic blood pressures should be maintained to ensure adequate cerebral perfusion [15].
Neonates are particularly susceptible to hypoxic events when MAP is too low. Further, these patients are particularly sensitive to drugs that depress myocardial function. The end result is that without meticulous management of blood pressure and ventilation to normocapnia, neonates and infants can suffer significant neurologic injury [16]. We have been conditioned to worry about the neurocognitive effects of anesthetic agents by the relatively recent myriad of literature. Anesthesiologists must first ensure that adequate physiologic parameters are maintained in order to achieve the best possible outcomes in neonates and small children.
Effects of Oxygen
Oxygen tension also affects CBF at the extremes, with CBF remaining constant over a wide range of oxygen tensions. In adults, when the partial pressure is less than 50 mmHg, CBF begins to increase to the point that at a PaO2 of 15 mmHg the CBF is four times normal in an effort to maintain O2 delivery [17]. This extreme increase in CBF will result in an increase in CBV, which in turn will result in an increase in ICP if the intracranial compliance is low. Hyperoxia conversely will lead to a decrease in CBF. Adults breathing 100 percent O2 will have a 10 percent decrease in CBF while neonates breathing 100 percent O2 will have up to a 33 percent decrease in CBF [9,10,18]. Hyperoxia has many adverse effects on neonates, especially premature neonates, so it is essential to balance adequate oxygen delivery with potential oxygen toxicity.
Effects of Carbon Dioxide
Carbon dioxide tension and CBF are related in a linear fashion. In adults, a 1 mmHg increase in PaCO2 increases CBF by approximately 2 ml per 100 g per minute [19]. Medically induced hyperventilation will lead to a decrease in CBF and CBV and thus ultimately decrease ICP for a short period of time, and is the basis of treating elevated ICP patients with hyperventilation. Elevations in PaCO2 will increase CBF. There is growing evidence that medically induced hyperventilation is on balance detrimental to most patients, including infants with brain injury, and is generally reserved to acutely treat high spikes of ICP. Cerebral ischemia can be exacerbated in brain-injured children who are treated with moderate hyperventilation for elevations of ICP [20].
Perioperative Management
Preoperative Evaluation
It is important to realize that the perioperative period is extremely stressful to both child and family. Parents have concerns about both the immediate risks of general anesthesia as well as the long-term consequences of their infant undergoing a prolonged general anesthetic for a neurosurgical procedure. These issues should be addressed during the preoperative interview in a reassuring manner. A very careful history and review of systems with an emphasis on the neurologic system is essential to obtain from the parents. In addition, it is very important to obtain a history of any coexisting diseases such as pulmonary and cardiac anomalies, prematurity, or evidence of difficult airway issues. Physical examination should focus on the airway, cardiac, pulmonary, and neurologic systems. A thorough history and review of symptoms with particular emphasis on neurological symptoms should be obtained from the parent. A focused physical examination with particular emphasis to the neurological system should be done.
Emergent neurosurgical procedures in infants pose special challenges. In most cases, the infants should be considered as a “full stomach” and airway precautions should be taken to prevent pulmonary aspiration. There may not be resources available to do a complete preoperative evaluation in neonates suspected of having congenital cardiac disease. A transthoracic echocardiogram along with pre- and postductal oxygen saturation on both room air and 100 percent oxygen can discriminate between cardiac and pulmonary disease.
For elective procedures, infants and neonates are fasted before general anesthesia in order to decrease the risk of pulmonary aspiration. It is important to minimize fasting time to decrease the risk of developing dehydration and hypoglycemia. For routine procedures, fasting times for clear liquids should be no more than two hours and for young infants intraoperative glucose monitoring should occur if the infants are not receiving intravenous glucose solutions intraoperatively.
As part of the preoperative evaluation, the anesthesiologist providing care for the infant should evaluate all neuroimaging studies that have been done on the infant in order to assess the type of lesion, location, the presence of intracranial hypertension, the vascular supply, and the risk of perioperative herniation. Most major neurosurgical procedures in infants are associated with intraoperative blood loss. Accordingly, it is necessary to type and crossmatch blood for intraoperative use. It is also important for the anesthesiologist to confer with the surgeon about special neurophysiologic monitoring that may be done intraoperatively in order to plan the optimal general anesthetic.
Vascular Access and Monitoring
Obtaining adequate intravenous access before incision is essential to conducting a safe general neurosurgical anesthetic. Once the procedure has commenced it is very difficult to place intravenous lines. Two relatively large-bore intravenous lines (larger than 22 gauge) are usually adequate for most procedures. Central venous access can be obtained if peripheral access is difficult. In general, femoral venous access is preferable to internal or external jugular venous access because they are technically easier to place and there is no concern about obstructing cerebral venous drainage. A single lumen central line is preferable to a multilumen central line if rapid transfusion of fluid and blood products is anticipated.
Placement of an intra-arterial catheter for blood sampling and monitoring is also very helpful for procedures in which significant blood loss is anticipated. The most commonly accessed vessel is the radial artery, but dorsalis pedis and posterior tibialis can also be easily accessed. It is important to zero the transducer at the level of the infant’s head in order to get an estimate of the cerebral perfusion pressure (CPP).
Infants undergoing craniotomies, especially in the head-up position, are at increased risk of venous air embolism so precordial Doppler is recommended in addition to standard ASA monitors. During some procedures, electroencephalographic (EEG) and neurophysiologic monitoring are used. Consultation with both the surgeon and neurophysiologist is necessary to coordinate the general anesthetic care.
Keeping the infant warm during neurosurgical procedures can be difficult. Because the head of a neonate is large in comparison with the rest of the body and a larger percentage of cardiac output is directed to the head and upper body, the neonate is at particular risk for significant heat loss during surgery. It is important to use warming lights and forced-air warming blankets, and to keep the operating room temperature high to prevent heat loss. Monitoring core temperature either esophageally or rectally is necessary. Intraoperative hyperthermia should also be scrupulously avoided.
Induction of Anesthesia and Airway Management
The most commonly used inhalational agent for children who are neurologically stable and lack intravenous access is sevoflurane. It lends itself to a rapid and smooth induction without significant myocardial depression and cardiovascular instability. Because inhalational inductions can be accompanied by respiratory depression leading to hypercarbia that could worsen elevated ICP, the anesthesiologist should be prepared to assist in ventilation relatively early during induction. Infants that are cardiovascularly unstable or who have already been accessed intravenously should have an intravenous induction with a rapid-acting hypnotic agent such as etomidate for unstable infants and propofol for stable infants. Although ketamine is a useful medication for infants with cardiovascular instability who are not neurologically compromised, it is not ideal for neurosurgical procedures. It will cause an increase in cerebral metabolism, CBF, and ICP, so it should be used cautiously in this patient population.
For emergency procedures in which the infant is felt to be at risk for pulmonary aspiration, a rapid sequence technique using succinylcholine or a large dose of a nondepolarizing medication such as rocuronium can be used. Care must be taken to ensure there are no contraindications to the use of succinylcholine, such as malignant hyperthermia, myopathies, or denervation diseases.
Elevations of ICP can occur with laryngeal intubation, so it is important to make sure that the anesthetic depth is appropriate before airway manipulation. In infants, a nasal intubation is often more easily secured and less likely to be inadvertently displaced during the surgery, especially when the infant is placed in the prone position.
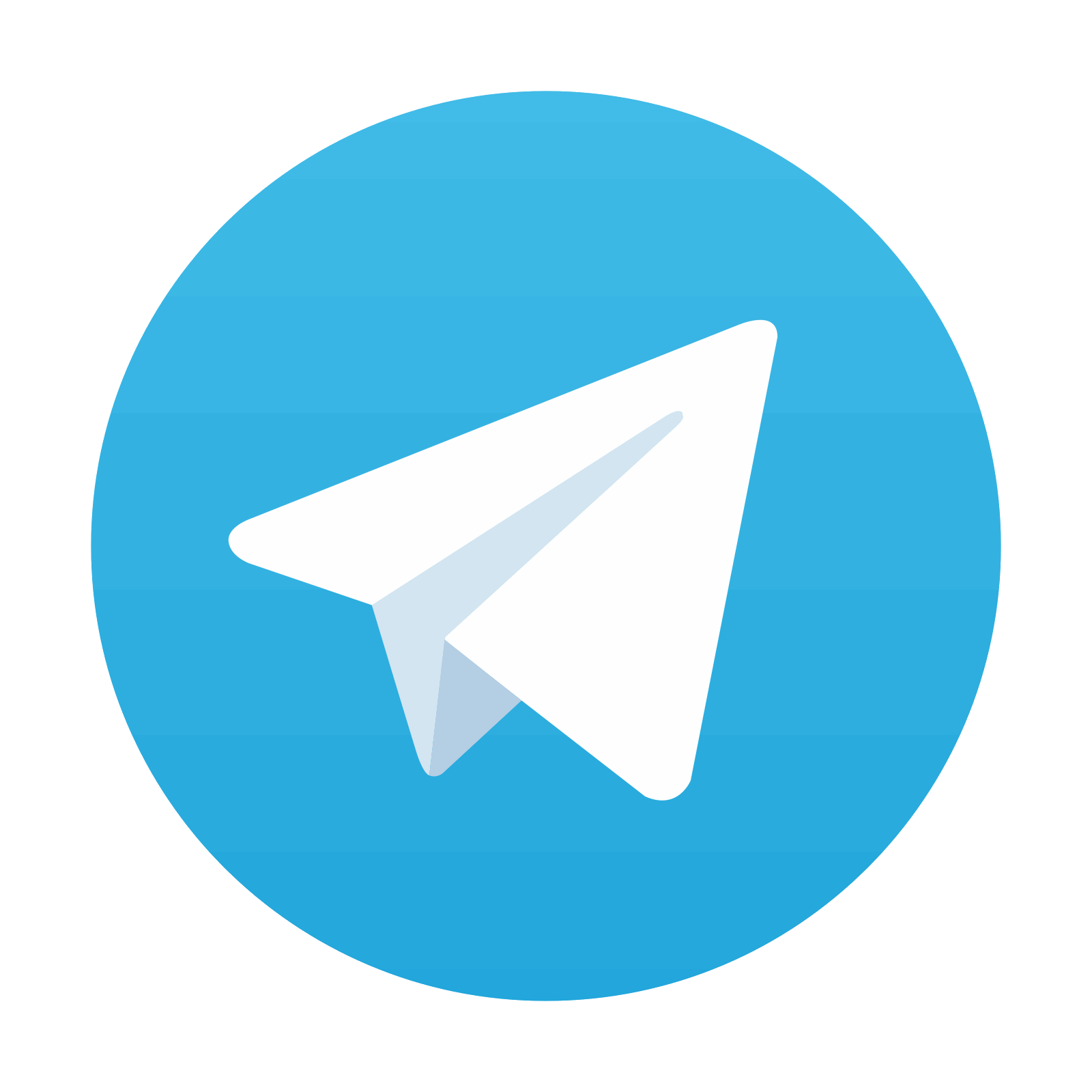
Stay updated, free articles. Join our Telegram channel

Full access? Get Clinical Tree
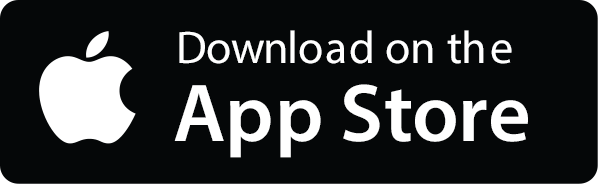
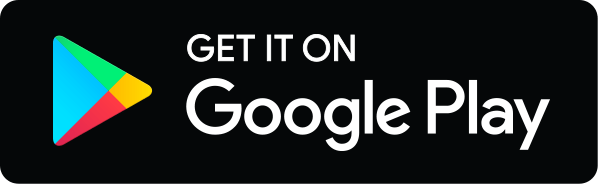
