Emergence of CA-MRSA with cases of rapidly progressive necrotizing pneumonia, often in previously healthy adults and children, is a new challenge for proper empiric antibiotic choices (24). The further introduction of highly resistant gram-negative pathogens, such as the Enterobacteriaciae with extended-spectrum β-lactamases (ESBL) and the emergence of Klebsiella pneumoniae with carbapenmase resistance may impact the empiric antimicrobial choices for pneumonia in other clinical settings—ventilator-dependent patients, nosocomially acquired infections—but generally do not impact the etiology of true CAP.
P. aeruginosa are naturally resistant to numerous antibiotics and can elevate to a high-level resistance under treatment. Risk of P. aeruginosa is increased in patients presenting with a previous chronic pulmonary disease such as chronic obstructive pulmonary disorder (COPD) or cystic fibrosis, recent antibiotic therapy, or a stay in the hospital, especially the ICU (11,25).
Specific Etiologies in Immunosuppressed Patients
Immunosuppressed patients have an increased risk of severe CAP; these patients have more frequent bacterial pneumonias, with the typical pathogen dependent upon the underlying immune deficiency.
Human immunodeficiency virus (HIV)-infected patients used to have a 25-fold higher risk of developing bacterial pneumonia as compared to the general population (26). This has largely abated due to the use of more active antiretroviral drugs and, in some studies, shows little difference from the general population (27). P. jirovecii pneumonia (PJP) remains a frequent acquired immunodeficiency syndrome (AIDS)-defining diagnosis, while less frequent causes include cytomegalovirus (CMV) and mycobacteria.
Patients with chemotherapy-induced neutropenia, particularly when severe (<500 neutrophils/μL) and prolonged (>10 days), have an increased risk of invasive pulmonary aspergillosis as well as severe bacterial pneumonia (28,29). In the proper clinical setting, with cavitary or mass-like lesions, this population may require empiric treatment for Aspergillus, usually with a triazole antifungal, such as voriconazole. This risk also exists with targeted monoclonal antibody therapies, which increase the risk of CMV and PJP (30). Patients with solid organ transplant and those receiving antitumor necrosis factor (TNF) monoclonal antibodies both have an increased risk of severe CAP caused by the usual bacterial pathogens, as well as by opportunistic infections such as P. jirovecii and Aspergillus (31,32).
Treatment
Antibiotic Therapy
Antimicrobial Spectrum. The ideal antibiotic should have a bactericidal activity against the major pathogens responsible for severe CAP. Considerations are given to the severity of clinical presentation, with non-ICU patients being distinguished from ICU patients in terms of empiric therapy. Additionally, special epidemiologic considerations may factor into this decision along with comorbid host conditions that may favor more resistant or unusual pathogens.
Timing of Initial Therapy. The most recent IDSA guideline recommends initial antibiotic administration within 4 hours of admission (25). A reduced mortality (adjusted odds ratio [AOR], 0.85, 95% confidence interval [CI] 0.76 to 0.95) was observed in patients with early therapy in a retrospective study of 18,209 Medicare patients (33). However, other studies contradicted this finding, and suggested that the time to first antibiotic dose was a marker of disease severity rather than an indicator of prognosis (34,35).
Antimicrobial Choices. Drug choice depends on numerous factors, including causative pathogen, pharmacodynamics/pharmacokinetics of the antimicrobial agent, spectrum of activity, adverse events, cost, host factors and, possibly, availability.
The antimicrobial agent must have sufficient diffusion in pulmonary tissues. β-Lactam antibiotics have a good extracellular diffusion, but are ineffective on intracellular organisms; their concentration in the alveolar lining fluid (ALF) reached 10% to 20% of the serum concentration after a single dose. Macrolides, on the other hand, have a variable intracellular distribution—low for erythromycin and elevated for clarithromycin and azithromycin. Fluoroquinolones have an excellent intracellular and extracellular diffusions with levofloxacin having increased activity against Legionella species as well as enhanced activity against S. pneumoniae (36).
Empiric choices must cover the most common pathogens in severe CAP. While less severe CAP will include S. pneumoniae, other atypicals, such as Mycoplasma, Chlamydia, and respiratory viruses, play a larger role. In severe CAP requiring ICU admission, S. pneumoniae remains common, but S. aureus, Legionella, and gram-negative bacilli rise in significance and must be empirically covered. Additionally, there are epidemiologic situations that may contribute to empiric choices. For example, alcoholism raises the concern for oral anaerobes and Klebsiella, COPD, and underlying chronic lung disease for Pseudomonas and Moraxella. Travel history, animal/bird exposures, and intravenous drug abuse may all suggest more unusual pathogens, including Coccidiodes species, Histoplasma, Hantavirus, or CA-MRSA.
Data suggests that combination regimens which include a macrolide or a quinolone are superior to β-lactam monotherapy for severe CAP (37–39). This is particularly important in cases of bacteremic pneumococcal pneumonia, in which combination therapy was confirmed as superior in an international, multicenter, prospective observational study (38,40,41). Lower mortality was associated with combination therapy for critically ill patients (14-day mortality, 23.4% vs. 55.3%; p = 0.0015), but not for all patients receiving combination versus monotherapy (10.4% vs. 11.5%, p = NS). All combinations using a β-lactam had an enhanced response.
The regularly updated guidelines published by North American and European medical societies recommend the utilization of a β-lactam with a macrolide or a respiratory fluoroquinolone. The 2007 IDSA/ATS guidelines concur, and are presented in Table 88.2.5.
Duration of Therapy. Length of treatment may be modified based on the pathogen, response to treatment, comorbid illness, and complications (42). In general, patients with CAP should be treated a minimum of 5 days, but at least until they are afebrile for 72 hours. Longer durations are needed for necrotizing infections caused by S. aureus—particularly when bacteremic due to endocarditis and/or metastatic infections—P. aeruginosa and Klebsiella, which should probably be treated no less than 2 weeks. Similarly, atypical intracellular pathogens are generally treated for a minimum of 2 weeks.
TABLE 88.2.5 Initial Empiric Antibiotic Therapy in Patients Admitted to the ICU for Severe CAP | |
![]() |
Nonantimicrobial Therapy
Besides antimicrobial therapies, most patients admitted to the ICU for severe CAP need additional treatment. End-organ dysfunction, such as respiratory failure, septic shock, or renal failure, require supportive measures. Similarly, the standard care for acutely ill patients (i.e., nutrition, prevention of ICU-related complications, and treatment of underlying diseases) must be utilized.
Activated Protein C. Severe sepsis is associated with a generalized inflammation and a procoagulant response to infection. Activated protein C is an important endogenous modulator of this response; there are reduced levels of activated protein C in most patients with severe sepsis.
Although drotrecogin-α activated, a recombinant form of human activated protein C (r-aPC), exhibits profibrinolytic, antithrombotic, and anti-inflammatory characteristics, and while large studies suggested that treatment with r-aPC significantly decreased 28-day and hospital discharge mortality rates in patients with severe sepsis, although not in lesser ill patients (43–46), this agent was ultimately found to be inefficacious and has been removed from the market.
Corticosteroids. Confalonieri et al. (47) studied hydrocortisone—200 mg i.v. bolus followed by infusion at a rate of 10 mg/hr for 7 days—randomized against placebo in a cohort of 46 patients. Findings included improvements oxygenation, radiographs, shock and multiorgan dysfunction, length of hospital stay, and mortality. While additional randomized trials are needed to recommend routine use for the treatment of severe CAP, use of hydrocortisone is clearly indicated in patients who do not have normal cortisol responses, and screening for occult adrenal insufficiency in patients who remain hypotensive after fluid resuscitation may be warranted. This is at least controversial (see Chapter 46, Sepsis and Septic Shock).
Expected Clinical Course
Evaluation on Day 3. Clinical response to treatment for severe CAP may not be seen in the first 48 hours. Expectations are that fever and oxygenation will, at least, stabilize even though they may not yet begin to improve. Empiric therapy should be continued during this time, allowing for preliminary culture data to return. Upon reassessment with microbiologic data, de-escalation may be supported or therapy modification may be necessary.
Complications and Failure to Improve. A poor clinical response by day 3 may be a sign of treatment failure. However, in the ICU, other diagnoses such as pulmonary embolism or cardiac failure should be considered in this situation. Treatment failure may be due to a non-infectious cause of the pulmonary issue (concomitant cardiac failure, pulmonary embolism), or to an organism not covered by the first-line antimicrobial therapy, thus necessitating a change or addition to the antimicrobial regimen. Other causes of treatment failure can include infectious complications of the pneumonia despite adequate antimicrobial therapy. These include lung abscess, empyema, endocarditis, or other superinfection.
Prognosis
Mortality in patients with severe CAP requiring admission to the ICU remains high, ranging in various series from 18% to 46% (8,48–54). A meta-analysis of 788 ICU patients found a mean mortality rate of 36.5% (55); in this analysis, Fine and colleagues (55) identified the following 11 factors independently associated with a higher mortality: male gender (OR = 1.3), pleuritic chest pain (OR = 0.5), hypothermia (OR = 5.0), systolic hypotension (OR = 4.8), tachypnea (OR = 2.9), diabetes mellitus (OR = 1.3), neoplastic disease (OR = 2.8), neurologic disease (OR = 4.6), bacteremia (OR = 2.8), leukopenia (OR = 2.5), and multilobar disease (OR = 3.1).
TABLE 88.2.6 Independent Prognostic Factors Associated with Mortality of Patients with Severe CAP |
![]() |
Numerous studies have focused on the prognosis of patients admitted to the ICU for severe CAP (48–50,52–54,56–58). Though inclusion criteria were variable, most independent prognostic factors were similar and demonstrated that, in general, survival in cases of severe CAP depends on the preadmission health status of the patient, the initial severity of illness, and the evolution during the ICU stay (Table 88.2.6). While these findings suggest that there are important nonmodifiable factors which influence mortality, the following points must be underscored:
- The initial empiric antimicrobials must be instituted as soon as possible (<4 hours after hospital admission).
- The empiric antibiotics must be broad enough to cover the most likely pathogens.
Studies have suggested that empiric initial antimicrobial treatment which utilizes a macrolide or a respiratory fluoroquinolone as a second agent to a β-lactam reduces mortality from CAP (37,59,60). Similar results are seen with bacteremic pneumococcal CAP when combination therapy is prescribed (40,41,61). Of note, however, is the fact that in these retrospective analyses, critically ill patients were often excluded. Rello et al. (38) specifically compared multiple antibiotic combinations and the resultant mortality for patients admitted to the ICU with severe CAP. Their major finding was that the addition of empiric aminoglycosides was suboptimal. Wilson and colleagues (62) compared the two regimens which are concordant with the latest IDSA/ATS guideline (β-lactam + macrolide and β-lactam + respiratory quinolone) and found no significant effect on mortality between these two options, though their findings did suggest an increased length of hospital stay when the quinolone was used instead of the macrolide.
Mortality risk can be assessed by the Pneumonia Severity Index (PSI),which stratifies patients into five classes according to the risk of death within 30 days using a two-step approach (63). First, patients with a low risk (class I) are identified by age younger than 50 years and the absence of comorbidities and vital sign abnormalities. For the remaining patients, a score is determined by adding points assigned to age, comorbid conditions, physical findings, laboratory and radiographic abnormalities (Table 88.2.7). According to the value of this score, patients are classified into class II (≤70 points), III (71 to 90 points), IV (91 to 130 points), or V (>130 points). From class I to class V, mortality rates observed were 0.1%, 0.6%, 2.8%, 8.2%, and 29.2%, respectively. Despite major interest in this finding, the indexing of patients in this manner was to identify patients at low risk for complications, who might be safely treated as outpatients. Consequently, the implications of the PSI for the medical care of patients exhibiting severe CAP, and requiring admission into an ICU, is unclear.
TABLE 88.2.7 Criteria and Point Scoring System Used in the Pneumonia Severity Index (Step 2; Classes II–V) | |||
![]() |
A specific prediction rule for mortality of patients with severe CAP admitted to an ICU was proposed by Leroy et al. (57), emphasizing both initial baseline patient characteristics and the patient’s evolution during the ICU stay. Upon ICU admission, an initial risk score based on the following six independent variables and their respective point value is established: age 40 years or older (+1 point); anticipated death within 5 years (+1 point); nonaspiration pneumonia (+1 point); chest radiograph involvement greater than one lobe (+1 point); acute respiratory failure requiring MV (+1 point); and septic shock (+3 points). Summation of these points places patients into one of three classes: class I (0 to 2 points; mortality risk is less than 5%), class II (3 to 5 points; mortality risk is 25%), and class III (6 to 8 points, mortality risk is >50%). Step two is to score three independent variables during the ICU stay. These three variables and their point scores are hospital-acquired, lower respiratory tract superinfections (+1 point); nonspecific CAP-related complications (+2 points); and sepsis-related complications (+4 points). This modified risk score most significantly altered the prognosis for patients initially categorized in the moderate range (class II) risk. The adjusted risk score determined subgroups of patients within this class who exhibited significantly different mortality rates ranging from 2% to 86%. Therefore, this score may help clinicians to reassess severe CAP patients during the ICU stay.
Prevention
Immunization remains the most significant method of prevention (25). Two vaccines are available for preventing pneumococcal disease. Pneumococcal polysaccharide vaccine (PPSV23) and pneumococcal conjugate vaccine (PCV13). Currently, the Advisory Committee on Immunization Practices (ACIP) recommends that all adults 65 years of age or older receive one dose of PCV13 followed by PPSV23 one year later. Adults 19 years of age or older who have functional or anatomic asplenia, CSF leaks, cochlear implants, and immunocompromising conditions (HIV, congenital immunodeficiencies, hematologic malignancies, solid organ transplant, chronic renal disease) should receive PCV13, again followed by PPSV23 no sooner than 8 weeks later. PCV13 alone is also recommended for children 2 years of age or greater who have immunocompromising conditions such as asplenia, sickle cell disease, HIV, or other immunocompromising conditions (64–67).
The ACIP recommends that all persons >6 months of age receive influenza vaccination annually. Persons who are between 2 and 49 years of age may receive either the live-attenuated intranasal vaccine or the inactivated intramuscular injection; the inactivated influenza vaccination should be used in persons 50 and older (68).
Ventilator-associated Pneumonia
VAP is defined as a pneumonia occurring in patients undergoing MV. Although usual guidelines suggest a delay of 48 to 72 hours between the beginning of MV and the occurrence of pneumonia to qualify for this diagnosis (69), some data suggest that a pneumonia acquired earlier than the 48th hour of MV could also be considered a VAP (2,70). VAP represents 80% of pneumonia acquired during hospitalization, and is the most frequent hospital-acquired infection in ICUs.
TABLE 88.2.8 Incidence of Ventilator-associated Pneumonia in the ICU |
![]() |
Immediate Concerns
The major considerations regarding VAP are:
- Prevention
- Acceptable “gold standard” for diagnosis
- Increasing rates of nosocomial drug-resistant pathogens
Incidence
The exact incidence of VAP is difficult to assess, as study populations vary widely because the criteria used to define VAP has evolved, especially from organizations such as the United States Centers for Disease Control (CDC). This leads to overlap between VAP and other hospital-acquired lower respiratory tract infections, such as nosocomial tracheobronchitis, nosocomial or community-acquired aspiration, and even non-infectious respiratory illnesses. With no gold standard for diagnosis, studies show very different incidence rates ranging from 5.6% to 82.4% (Table 88.2.8) (70–78). Nevertheless, the CDC reports a decline in the incidence of VAP over the past several years in the United States, with rates up to 6% (79).
While risk for VAP increases the longer the patient is intubated, the daily hazard rate may decrease over time. In a cohort of 1,014 patients ventilated for 48 hours or more, the overall incidence of VAP was 14.8 cases per 1,000 ventilator days. The daily hazard rate for developing VAP was estimated to be 3% per day at day 5 but only 1.3% by day 15 (80). Overall, the risk of infection is the highest during the first 8 to 10 days of MV and increases with the duration of MV (80,81).
Pathogenesis
Pneumonia is essentially the introduction of pathogenic bacteria into the normally sterile lower respiratory tract, where colonization followed by invasive infection may occur (69,82). Bacteria may reach the lower respiratory tract by four different pathogenic mechanisms: (1) contiguous spread; (2) hematogenous spread; (3) inhalation; and (4) aspiration. The first two mechanisms of invasion are infrequent (83). Inhalation refers to the direct inoculation of the respiratory tract by way of an aerosol, such as a contaminated ventilator circuit, a nebulized treatment, or even a contaminated bronchoscope; these would all be considered fairly rare events. The major mode of entry for pathogenic bacteria into the lower respiratory tract is by aspiration of oropharyngeal organisms. Colonization of the oropharyngeal airways by pathogenic micro-organisms occurs during the first week of hospitalization and they are more likely to be gram negative and drug-resistant. The endotracheal tube eliminates the protective barriers between the oropharynx and lower respiratory tract, and leakage around the endotracheal tube cuff of secretions, now colonized with more pathogenic bacteria, allows access to the trachea (69,82).
Risk Factors
Patient-related risk factors include male gender, pre-existing pulmonary disease, coma, AIDS, head trauma, age over 60 years, neurosurgical procedures, and multiorgan system failure (84).
The presence of MV alone is associated with a 3- to 21-fold risk of pneumonia (72). The endotracheal tube not only limits the natural elimination of oral secretions contaminated with nosocomial pathogens, but it also impairs ciliary clearance and cough. Furthermore, the MV patient requires other devices, such as nebulizers or humidifiers, which can be an additional, albeit unusual, source of micro-organisms.
Accidental extubation can be distinguished from a more controlled tracheal decannulation as independently elevating the risk for a VAP (85). This may be due to the fact that proper oral care and preparation for the extubation did not occur, and that the patient may be confused or an aspiration risk. GI risk factors include the use of enteral nutrition administered by a nasogastric, rather than a gastrostomy tube, and the use of H2 blockers and proton pump inhibitors which favor gastric colonization of pathogens due to the elevated pH (80,84).
Other factors that facilitate the inhalation of oropharyngeal secretions include: supine position, patient transportation out of the ICU (86), sedation (87), failed subglottic aspiration of secretions (81), and intracuff pressure less than 20 cm H2O.
Etiology
VAP may be caused by a wide spectrum of bacteria, and is often polymicrobial (Table 88.2.9) (88–90). P. aeruginosa, S. aureus, and gram-negative enteric bacilli are the leading etiologies. However, pathogens may differ according to patient groups, unit types, hospitals, and countries (90). Moreover, the antimicrobial resistance patterns may vary widely with geographic location.
Several studies have tried to identify specific risk factors associated with specific pathogens. The presence of an altered level of consciousness, admission into a medical ICU, and a high Simplified Acute Physiologic Score (SAPS) are independently associated with aspiration of anaerobes (91). In trauma patients, tracheostomy and prior antimicrobial use with cefepime are associated with Stenotrophomonas maltophilia (92). Cytotoxic chemotherapy and use of corticosteroids predispose to pneumonia due to L. pneumophila (93). Neurosurgery, acute respiratory distress syndrome (ARDS), head trauma, and large-volume pulmonary aspiration have been associated with Acinetobacter baumannii (94). COPD, prior use of antibiotics, and duration of MV longer than 8 days are independently associated P. aeruginosa (95). Finally, coma is an independent risk factor for VAP caused by S. aureus (96). These risk factors are not sufficient to narrow initial empiric therapy, but are considerations when empiric therapy must be broadened in the critical patient for whom microbiologic studies are pending.
TABLE 88.2.9 Micro-organisms Causing Ventilator-associated Pneumoniaa | |||
![]() |
The day of onset of VAP may influence the etiology, making it potentially useful to distinguish early-onset from late-onset VAP. The definitions of early and late-onset VAP may vary, but the IDSA/ATS guidelines define duration of hospitalization fewer than 5 days as early onset, and greater than 5 days as late onset (69,82,97). In early-onset VAP, the main causative pathogens are still community-based and include S. pneumoniae, methicillin-susceptible S. aureus (MSSA), H. influenzae, and susceptible gram-negative enteric bacilli (97). In late-onset VAP, the organisms reflect nosocomial pathogens and include MRSA, P. aeruginosa, A. baumannii, and S. maltophilia.
Among these potential nosocomial pathogens, the rate of multidrug-resistant (MDR) pathogens has been increasing. Factors associated with the probability of a drug-resistant pathogen are summarized in Table 88.2.10. Duration of hospitalization (and/or MV) and prior exposure to antimicrobial agents are the major risk factors for VAP due to MDR pathogens (88,89,98–101). Finally, VAP due to fungi such as Candida species, Aspergillus species, or to viruses such as influenza, parainfluenza, and respiratory syncytial virus is uncommon in immunocompetent patients (69).
TABLE 88.2.10 Risk Factors for Multidrug-resistant Pathogens as Causative Organisms in Ventilator-associated Pneumonia |
![]() |
Diagnostic Strategies and Testing
Pneumonia is suspected when a patient exhibits signs and symptoms suggesting both pulmonary involvement and infection. There remains, however, significant interobserver variation in the interpretation of radiographs and clinical determination of pneumonia such that a clear epidemiologic definition is difficult. The CDC, accordingly, removed radiography altogether as a component of the VAP definition used in the NHSN database and currently uses the following criteria to define ventilator-associated events (VAEs), infection-related ventilator-associated complications (IVAC), and possible ventilator-associated pneumonia (PVAP) (102). For a VAE, the patient must have the following:
- A baseline period of stability or improvement on the ventilator defined by at least 2 calendar days of stable or decreasing daily minimum FiO2 or PEEP values. The daily minimum FiO2 or PEEP is the LOWEST value during a calendar day that has been maintained for 1 hour.
- After a period of stability, the patient must have at least one of the following indicators of worsening oxygenation:
- Increase in daily minimum FiOs of at least 0.20 (20 points) over the daily minimum FiO2 from the baseline period, and that increase must be sustained for 2 or more calendar days, or
- Increase in daily minimum PEEP values of at least 3 cm H2O over the daily minimum PEEP in the baseline period, sustained for 2 calendar days or longer
- Increase in daily minimum FiOs of at least 0.20 (20 points) over the daily minimum FiO2 from the baseline period, and that increase must be sustained for 2 or more calendar days, or
In addition to the above criteria, for an IVAC the patient must also have:
- On or after calendar day 3 of MV and within 2 calendar days before or after the onset of worsening oxygenation, the patient meets both of the following:
- Temperature over 38°C or under 36°C, or white blood cell count of at least 12,000 cells/mm3 or no more than 4,000 cells/mm3, and
- A new antimicrobial agent is started and is continued for at least 4 calendar days.
- Temperature over 38°C or under 36°C, or white blood cell count of at least 12,000 cells/mm3 or no more than 4,000 cells/mm3, and
In addition to the above criteria, for a PVAP the patient must also have:
- Positive culture of endotracheal aspirate or BAL or lung tissue or protected tip brush which meet the quantitative or semi-quantitative thresholds defined in the protocol, or
- Purulent respiratory secretions (>25 neutrophils with <10 squamous cells per low powered field, PLUS the organism is identified in one of the following:
- Sputum, endotracheal aspirate, BAL, lung tissue, protected tip brush, or
- One of the following tests must be positive as defined in the protocol:
- Pleural culture
- Lung histopathology is consistent with infection
- Diagnostic test for Legionella
- Diagnostic test for viral pathogens
- Pleural culture
The recent ATS/IDSA guidelines propose a “mixed” diagnostic strategy as follows (69):
- The diagnosis of VAP is suspected in the presence of a new or progressive pulmonary infiltrate associated with at least two of the following three infectious signs: fever over 38°C, leukocytosis or leucopenia, and/or purulent secretions. For patients with ARDS, radiographic changes are difficult to analyze, consequently, hemodynamic instability and/or deterioration of blood gases could be considered sufficient to suspect VAP.
- As soon as the diagnosis is suspected, lower respiratory tract samples are obtained for microscopy, and quantitative or semi-quantitative cultures and empiric antimicrobial therapy are started unless there is both a low clinical suspicion for VAP and a negative microscopy of the respiratory sample.
- On days 2 and 3, the results of cultures should be available, and the clinical response is assessed. According to whether the clinical picture is improving or worsening and the results of cultures, antimicrobial therapy will be stopped, de-escalated, or adjusted, and an investigation for other pathogens, other diagnoses, other sites of infection, or complications is performed.
With the ATS/IDSA definition, all acutely ill, MV patients should have a complete daily investigation including physical examination, an anteroposterior portable chest radiograph, measurement of arterial oxygenation saturation, and determination of necessary laboratory values (complete blood count, serum electrolytes, renal function). When VAP is suspected, each patient should have a complete physical examination to search for another source of infection, arterial blood analysis, and blood cultures collected. In cases of large pleural effusions, a diagnostic thoracentesis is indicated unless there is a contraindication. Samples of lower respiratory tract secretions (endotracheal aspirates or bronchoscopic samples) should be quantitatively cultured (69).
Finally, purulent sputum, even when associated with fever and leukocytosis, may be due only to nosocomial tracheobronchitis (103). An additional, more simplistic, definition would add the presence of a new or progressive radiographic infiltrate associated with at least two of three major clinical findings (104).
In addition to the issues with a consistent clinical definition of VAP, numerous techniques have been proposed to identify causative organism(s) of VAP. Blood cultures are rarely positive and a positive result may reflect an extrapulmonary infection (105). Pleural fluid cultures are helpful if positive, but are rarely needed. Analysis of lower respiratory secretions is the most frequently used technique to identify causative organism(s) of VAP. Numerous sampling methods have been described, with the major ones being endotracheal aspiration and bronchoscopic techniques with protected specimen brush (PSB) and/or BAL. These samples may be examined by various stains as described previously under CAP (Gram stains, Giemsa, acid fast stains) and by qualitative, semi-quantitative, and quantitative cultures. Among all the described microbiologic techniques each have advantages and disadvantages (69,82). Specifically:
- Microscopy and qualitative culture of expectorated sputum or endotracheal aspirates are associated with a high percentage of false-positive results because of colonization of the upper respiratory tract and/or tracheobronchial tree. However, the initial empiric antimicrobial treatment of VAP could be guided by a reliable tracheal aspirate Gram stain.
- Quantitative cultures with a threshold of 106 colony-forming units (CFU)/mL to differentiate colonization from lung infection provide a diagnostic accuracy nearly similar to that of quantitative cultures from samples obtained by bronchoscopic techniques (106,107). Moreover, when the culture of endotracheal secretions is sterile in a patient with no recent (<72 hours) change in antimicrobial therapy, the diagnosis of a lower respiratory tract infection can be ruled out with a high probability (the negative predictive value is greater than 90%) (108).
- A PSB allows the collection of uncontaminated specimens from the potentially infected pulmonary area. A threshold set at 103 CFU/mL is the most adequate for quantitative cultures (109); false-positive results are infrequent (107). False-negative results may be observed when sampling is performed at an early stage of infection, in a technically incorrect (unaffected pulmonary area) manner, in a patient where a new antimicrobial treatment has been initiated, and/or if the specimens are incorrectly processed.
- BAL explores a larger lung area than PSB. Quantitative cultures of BAL fluid, with a threshold set at 104 CFU/mL, provide results similar to those obtained by PSB (109). Microscopic examination of the BAL specimen may add be critical to rule in certain diagnoses (silver staining for Pneumocytis, for example) (110,111).
While none of these techniques are without risk of false positives and negatives, an accurate microbiologic diagnosis may reduce VAP mortality (112) and should be attempted.
Antibiotic Treatment
Principles of Initial Empiric Treatment
Prompt initiation of adequate antimicrobials is the cornerstone of treatment for VAP, as studies have shown that inadequate initial coverage is associated with an increased mortality (98,113–115). The excess mortality due to inadequate antimicrobial coverage is not reduced if antibiotics are corrected once bacteriologic data is available (114,115); coverage must be broad initially then de-escalated subsequently. In addition to the proper coverage, Iregui et al. (116) showed that if the antimicrobials were delayed by ≥24 hours, mortality more than doubled (69.7% vs. 28.4%; p < 0.001).
Adequate antibiotic therapy could, therefore, be defined as the administration, at an appropriate dose, of at least one antibiotic with good pulmonary penetration, and bactericidal activity against the major causative pathogens, in a timely manner. One of the more common reasons for inadequate initial coverage is the growing pattern of antimicrobial resistant pathogen(s). Consequently, the presence or absence of risk factors for MDR pathogens (see Table 88.2.10) and the local microbiologic patterns must be considered when choosing empiric treatment.
TABLE 88.2.11 Initial Empiric Antibiotic Therapy in Patients with Ventilator-associated Pneumonia | |
![]() |
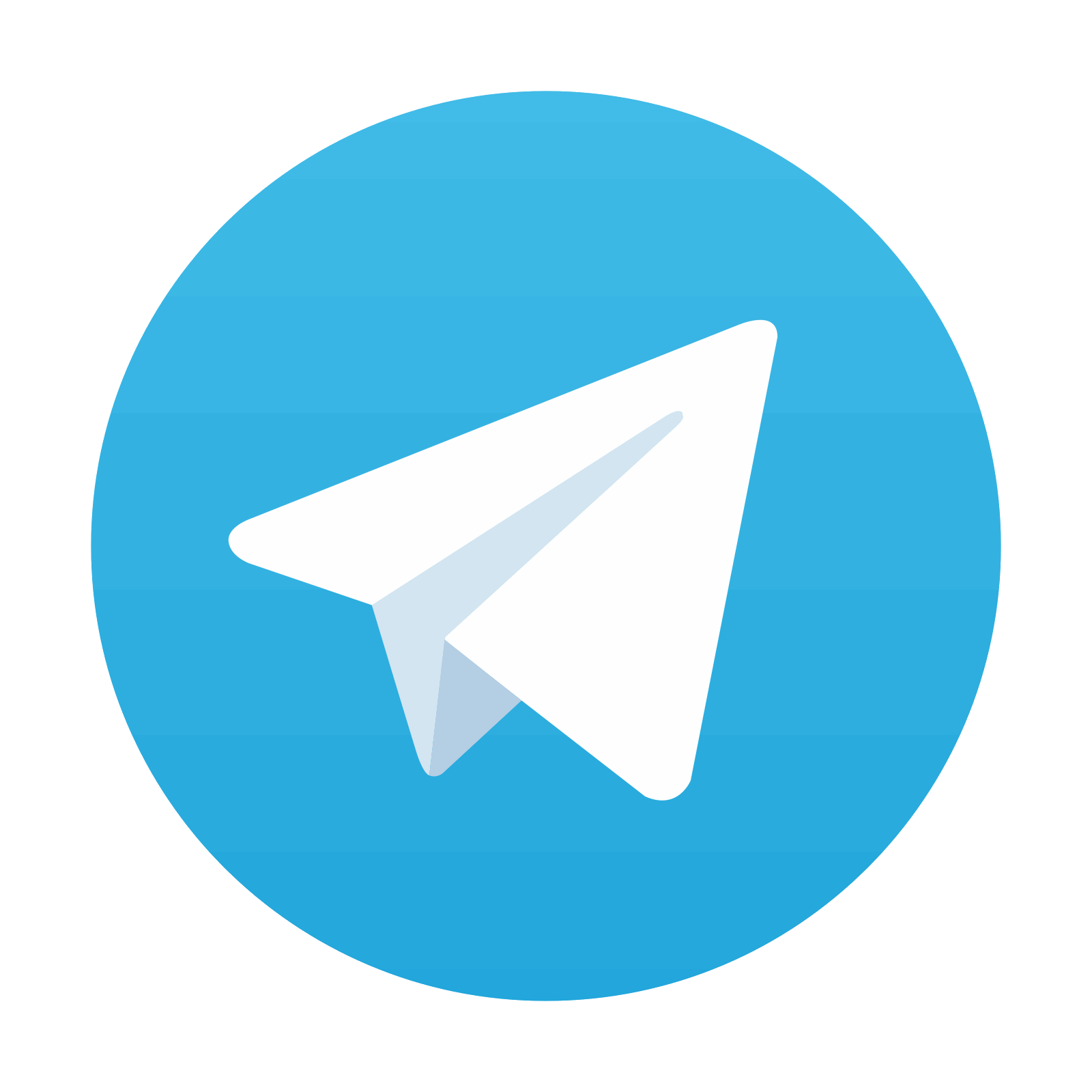
Stay updated, free articles. Join our Telegram channel

Full access? Get Clinical Tree
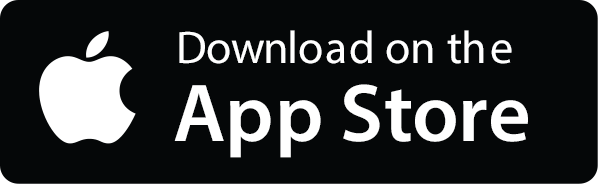
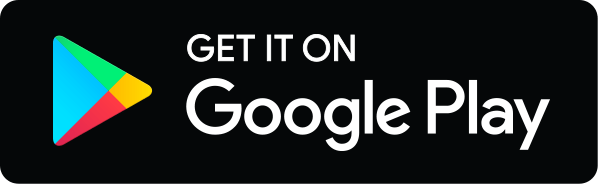