Introduction
The differential diagnosis of hypotonia in newborns and infants is significantly different from that of older pediatric patients. Core myopathies (central core disease and multiminicore disease), which are considered highly susceptible to malignant hyperthermia (MH), for example, are the most common causes of congenital myopathy, but not of myopathy in older children [1]. Congenital hypotonia may be caused by primary muscle disorders as well as motor neuron disorders, myasthenia gravis, and mitochondrial defects. Pediatric anesthesiologists will require a working understanding of the causes of hypotonia in newborns and infants in order to avoid potentially disastrous consequences and provide the safest possible care [2].
Primary muscle disorders in neonates consist of a heterogeneous group of diseases that can be broadly divided into congenital muscular dystrophies and congenital myopathies [3]. Research in the last two decades has led to identification of hundreds of genetic loci responsible for the molecular basis of neuromuscular disorders [4–6]. While the identification of specific defective proteins has added to our understanding of the pathophysiology of muscle development and the neuromuscular junction, the landscape of muscle disorders in neonates is confusing since there are numerous naming conventions in widespread use. The aim of this chapter is to provide an overview of primary muscle disorders in newborns and infants, as well as the other major causes of hypotonia in this age group. The final part of the chapter summarizes the approach to the anesthetic management of the hypotonic patient.
Primary Muscle Disorders
Primary muscle disorders presenting in the neonatal period are broadly divided into congenital muscular dystrophies (CMDs) and congenital myopathies (CMs) [3]. In general, the CMDs are characterized by severe disruption of muscle architecture on tissue biopsy, whereas CMs show a relative preservation of muscle cell structure and fiber type proportion.
Congenital Muscular Dystrophies
Congenital muscular dystrophies are a heterogeneous group of conditions that result from disruption of the structural connection between the contractile elements of the muscle cell (the actin–myosin filaments) and extracellular matrix. These connections are crucial for transducing the contractile force of actin–myosin filaments to the surrounding connective tissue. Loss of this transduction results in muscle weakness, disruption of related elements of the muscle cell, and progressive deterioration of the muscle fiber. CMDs should be considered multi-organ system diseases and often present with significant central nervous system (CNS) involvement. While Duchenne and Becker muscular dystrophies are well-known to most anesthesiologists, the CMDs may seem unfamiliar by comparison.
CMDs are classified by their genetic mutations and resultant biochemical defects. The most well-known are the dystroglycanopathies caused by defects in connective tissue glycoproteins which form the contractile element–extracellular matrix connection described above. Of these, the most familiar is caused by defects in the LAMA2 extracellular matrix protein (primary merosin deficiency) often with profound loss of motor function and some CNS white matter deterioration. Patients with primary merosin deficiency are usually symptomatic at birth with generalized hypotonia, weak cry, respiratory insufficiency, seizures, and sometimes multiple congenital joint contractures (arthrogryposis) [7,8].
Mutations in the POMT1, POMT2, and FCMD glycoproteins are also common (Walker–Warburg syndrome [WWS], Muscle–Eye–Brain [MEB] disease, and Fukuyama CMD, respectively). Walker–Warburg Syndrome and the less severe MEB disease typically present at birth with hypotonia, lissencephaly, hydrocephalus, cerebellar and retinal dysplasia, arthrogryposis, respiratory insufficiency, and sometimes cleft lip and palate [9]. Patients with WWS rarely live beyond one year. Fukuyama CMD presents with hypotonia and respiratory insufficiency in early infancy along with cerebral and cerebellar dysplasia, hydrocephalus, and seizures. Cardiomyopathy occurs in patients that survive into the second decade of life [10]. An increasingly large group of other genetic defects can cause rare forms of CMD, most of which require similar perioperative considerations as those listed above [3].
The final common form of CMD is myotonic dystrophy type 1 (DM1), which results from an unstable trinucleotide expansion in the DMPK gene on chromosome 19. While there is rough correlation between the number of triplets and earlier age of onset when CTG repeats are less than 400, the congenital phenotype is often characterized by more than 1500 CTG repeats. Prenatal findings often include polyhydramnios and decreased fetal movements. Postnatally, hypotonia and immobility are apparent, as are clubfoot deformities and arthrogryposis. Weakness in the head and neck results in a weak cry, impaired swallowing, and a characteristic triangular open mouth. Respiratory muscle weakness is common and mechanical ventilation is often required for the first weeks of life. Cardiovascular problems are not common in the newborn period, but cardiomyopathy and pulmonary hypertension have been reported [11]. Although DM1 is an autosominal dominant trait, the mother is the transmitting parent in 94 percent of cases because the female locus is more unstable than the male locus [12]. In most cases the mother is unaware of being affected, but detailed examination reveals mild facial weakness and grip myotonia.
Muscular dystrophies associated with older pediatric patients have also been reported in patients younger than one year of age. In particular, limb-girdle muscular dystrophy and Emery–Dreifuss muscular dystrophy have been described in the newborn period, presenting with reduced fetal movements and severe hypotonia at birth [13,14].
Congenital Myopathies
Congenital myopathies are primary muscle disorders that are typically nonprogressive and characterized by “nondystrophic” changes on muscle biopsy, i.e., no degeneration of the muscle. Traditionally, CMs are classified by histopathologic staining patterns.
Nemaline Myopathies
Nemaline (from Greek nema, thread) myopathies first took their name from the threadlike structures seen on muscle biopsy specimens. Two forms are seen when the disease presents in the newborn period: classical and severe. In the classical form, infants show generalized weakness involving facial and axial muscles as well as bulbar and feeding difficulties often requiring frequent suctioning and tube feeding. The severe phenotype is characterized by a history of polyhydramnios, severe weakness, arthrogryposis, severe feeding difficulties, and respiratory failure [15]. Serum CPK levels are normal or mildly elevated. No susceptibility to MH is documented in nemaline myopathies. However, as is the case with all myopathies, depolarizing muscle relaxants should be avoided due to the potential for hyperkalemia.
Central Core Disease
Central core disease (CCD) is one of the most common CMs [1]. With histochemical staining, distinct cores of absent oxidative activity are seen running the length of Type 1 muscle fibers. There is a wide variation in the clinical presentation of CCD, and while weakness may become clinically apparent for most affected individuals during infancy and early childhood, contractures are frequently present at birth (hip dislocation, equinovarus foot deformities). For the majority of patients, there is no bulbar or diaphragmatic weakness. Severely affected patients, however, may present in the neonatal period with scoliosis, arthrogryposis, and facial, bulbar, and respiratory insufficiency [16]. Serum CK levels are normal or mildly elevated [17]. Central core disease is usually inherited in an autosomal dominant fashion and is associated with at least 22 different mutations in the skeletal muscle ryanodine receptor (RyR1). Sporadic mutations and even recessive pedigrees have also been reported [18]. The RyR1 mutations seen in CCD are associated with susceptibility to MH in older patients; however, MH-like responses have not been reported in neonates.
Multiminicore Disease
Multiminicore Disease (MmD) is a rare autosomal recessively inherited CM. In contrast to CCD, the cores are multiple and indistinct and do not extend the length of the muscle fiber. Four clinical phenotypes have been described: a classical form with predominantly axial muscle weakness progressing to scoliosis; a moderate form with generalized muscle weakness affecting the pelvic girdle and hand involvement; a classical form with external ophthalmoplegia; and an antenatal onset form with arthrogryposis. Both the moderate and external ophthalmoplegia forms are associated with an RyR1 mutation, whereas other forms are associated with mutations in SEPN1 and ACTA1. Malignant hyperthermia has been reported only in MmD with RyR1 mutations, unlike with the other forms of MmD; nonetheless, some authors have advised caution in using volatile anesthetics in all patients with the disease [17,19].
Centronuclear Myopathy
Histochemical staining in centronuclear (myotubular) myopathy demonstrates a characteristic pattern of numerous centrally placed nuclei with a surrounding zone of absent oxidative enzyme activity. Like other CMs, there is a high degree of variability in clinical phenotype. The most severe neonatal form follows an X-linked mode of inheritance and presents with marked hypotonia, respiratory failure, dysphagia and undescended testes, whereas the autosomal recessive forms are more variable, ranging from significant weakness and inability to walk to milder phenotype with presentation in later childhood. Centronuclear myopathy is not associated with MH [20].
Motor Neuron Disorders
Spinal Muscular Atrophy
Spinal muscular atrophy (SMA) is a relatively common autosomal recessive progressive degenerative disease affecting motor neurons of the anterior spine and brainstem. SMA is the result of mutations in the protein survival motor neuron 1 (SMN1). The function of SMN1 is unclear, although it is thought to have an anti-apoptotic role specifically in motor neurons. The classification of SMA is based on age of onset and severity of symptoms. Type 1 SMA, also known as Werdnig–Hoffman disease, presents at birth to six months of age with sudden-onset axial and proximal limb weakness without facial weakness. Intercostal muscles are severely affected, but the diaphragm is spared. As a result, the chest assumes a characteristic bell-shaped appearance, but overt respiratory failure usually does not occur in infancy. Bulbar weakness is a classic feature, which results in difficulty swallowing and pooling of secretions in the hypopharynx. Infants with SMA are prone to recurrent respiratory infections and are rarely expected to survive beyond two years of age without tracheostomy placement. Type 2 SMA presents at 6–18 months of age and is less severe than Type 1. Type 3 presents in later childhood and has the slowest progression of disease. Cardiac malformations such as hypoplastic left heart and atrial and ventricular septal defects have been reported in babies with SMA Type 1. Pulmonary complications are the leading cause of anesthetic mortality and morbidity in SMA Types 1 and 2. In addition to the classical SMA Types 1–3, a more profound form of the disease has been more recently described. Type 0 SMA presents prenatally and requires mechanical ventilation immediately at birth [21,22]. The anesthetic approach to these patients must be individualized depending on the severity of the disease, although succinylcholine should be avoided to prevent hyperkalemia [23].
Diaphragmatic Spinal Muscular Atrophy
Diaphragmatic SMA, also known as spinal muscular atrophy with respiratory distress (SMARD), has features in common with SMA but with additional diaphragmatic weakness. SMARD therefore presents in infancy with life-threatening respiratory failure. Unlike with SMA, the distal rather than proximal muscles tend to be affected in SMARD. Mutations in the gene encoding immunoglobulin-binding protein 2 are responsible for the disease.
Myasthenia Gravis
Transient Neonatal Myasthenia
Transient neonatal myasthenia is a phenomenon occurring in 20 percent of infants born to mothers with myasthenia gravis and is the result of placental transfer of acetylcholinesterase receptor antibody. The severity of maternal myasthenia gravis does not correlate to severity of neonatal signs and symptoms, and the disease is sometimes seen in babies born to undiagnosed mothers with subclinical myasthenia. Transient neonatal myasthenia presents at birth or within the first three days of life with generalized hypotonia and weakness including facial involvement. The diagnosis is confirmed by administration of anticholinesterase. Ventilatory and nutritional support may be needed until spontaneous recovery occurs by 2–4 months. Symptomatic infants may be given scheduled pyridostigmine until symptoms resolve [24].
Rarely, other forms of neonatal myasthenia present and are the result of genetic defects in neuromuscular transmission (e.g., decreased or dysfunctional acetylcholine receptors, defective acetylcholine recycling, abnormalities in synapse formation). These forms of disease are difficult to treat and may present with arthrogryposis and profound weakness in all muscle groups.
Glycogen Storage Diseases
Glycogen storage diseases are generally thought to appear later in childhood; however, the severe forms listed below can present during the neonatal period and even prenatally.
Infantile Glycogen Storage Disease Type II (GSD II, Pompe Disease)
Pompe disease (also known as infantile acid maltase deficiency) can manifest in the first weeks or months of life with diffuse hypotonia and weakness, giving these infants an extremely hypotonic appearance (floppy baby or rag doll syndrome) [25]. The early onset of the infantile form compared to forms appearing later in childhood is likely due to less residual enzyme activity. Cardiomegaly is often pronounced and there is progression of respiratory insufficiency due to hypotonia. Of importance, there is a neurogenic component to the weakness with motor neuron involvement. Apparent muscle bulk may be increased, however, and macroglossia, along with pharyngeal muscle weakness, predisposes the patient to upper airway obstruction. Fortunately, the prognosis for this disease has greatly improved in the last decade with enzyme replacement therapy.
Glycogen Storage Disease Type IV (GSD IV, Branching Enzyme Deficiency, Andersen Disease)
This defect can also present in infancy and is termed fatal infantile GSD IV (a fetal version also occurs and is a more severe form than the infantile version) [25]. The infantile version is characterized by hypotonia, muscle and neuronal involvement, cardiomyopathy and, often, early death. However, occasional patients survive into childhood and may be seen by the anesthesiologist. At present, there is no effective therapy for this defect.
Mitochondrial Myopathies
Mitochondrial myopathies are (as their name implies) caused by abnormalities in mitochondrial function. Mitochondria are the principal source of energy metabolism within cells and have critical effects on anesthetic management [26]. Within mitochondria reside the enzymes responsible for the Krebs cycle, fatty acid β-oxidation, and, most importantly, oxidative phosphorylation. Since mitochondria are important for supplying ATP in most tissues (most importantly nerve and muscle), the symptoms usually include myopathy and cardiomyopathy, and in the nervous system encephalopathy, seizures, and developmental delay. Mitochondria are also important in triggering cell death, or apoptosis, thus mitochondrial diseases may also lead to other organ dysfunction via this mechanism as well.
Cells are also able to use glucose to generate ATP by use of glycolysis without involvement of the mitochondria. Restriction of energy production to glycolysis is an anaerobic process and postnatally often leads to lactic acidosis. However, in utero, in the presence of low oxygen tension, most ATP is normally generated by glycolysis, which explains why many infants with severe mitochondrial defects do well until birth. The resulting lactate is then removed by the maternal circulation. In fact, fetal demise is not noted as a common component of mitochondrial disease in multiple reviews of neonatal disease. Other than glycolysis, all other energy-producing substrates (further oxidation of the glucose product pyruvate, fatty acids, amino acids) require the mitochondrion for their effective use. Inhibition of any of these metabolic pathways will lead to chemical derangements, often with profound effects. In general, mitochondrial defects can be recognized by increases in lactate or pyruvate, increases in systemic acylcarnitines, or altered amounts of amino acids. Generally, a metabolic abnormality in a patient with a myopathy or encephalopathy should raise the possibility of a mitochondrial defect. Great care must be taken to account for these defects when caring for these patients in the operative or perioperative period since the abnormal metabolite may be partially causative for the disease symptoms (e.g., acidosis).
The more commonly seen neonatal mitochondrial syndromes are Leigh disease, Kearns–Sayre syndrome, and Leber hereditary optic neuropathy. Much has been written about the presentation of mitochondrial disease in children and adults; however, little has been presented about mitochondrial disease prenatally or in the neonate. Prenatal presentations were discussed in 2003 by von Kleist-Retzow et al. [27]. Generally the authors noted intrauterine growth retardation and a variety of developmental abnormalities. A recent review reported on the neonatal presentation of 32 infants with mitochondrial disease, diagnosed as Leigh-like syndrome (encephalopathy with brainstem involvement), mitochondrial cytopathy, or lethal infantile mitochondrial disease [28]. The most common symptoms were poor feeding, recurrent vomiting, and failure to thrive with a lactic acidosis. Common clinical signs including encephalomyopathy, hepatopathy, intestinal dysmotility and cardiomyopathy were accompanied by an extremely high mortality regardless of presentation [28,29]. Twenty-seven of the neonates with documented disease had defects in the electron transport chain, while the others had various other enzymatic deficiencies. The most common of the other deficiencies was a defect in the DNA polymerase, POLG, which was associated with a hepatopathy.
Complex I is the largest component of the electron transport chain, consisting of at least 45 protein subunits, and represents the most common type of mitochondrial defect in the neonatal period [30]. Distelmaier et al. found the clinical presentation of newborns with complex I disease to include profound hypotonia, respiratory failure, seizures, and neurologic regression (consistent with early-onset Leigh disease) and notable in that there was generally no prenatal disease [31]. Similarly, defects in Coenzyme Q synthesis may cause multisystemic disease in the neonatal period, often accompanied by nephrosis [32,33]. Defects in other complexes can also cause neonatal disease, but the presentations are similar to those for complex I defects.
It is a common mistake to regard all mitochondrial diseases as a singular entity. Even family members carrying the identical mitochondrial gene mutation may present with dramatically different symptomatology. Because of this variability, it is dangerous to imply that, because an anesthetic technique was successful in a few patients with mitochondrial disease, it is safe for all patients with mitochondrial dysfunction. Since motor neurons may be affected, a hyperkalemic response to succinylcholine may be seen. Lastly, although MH is occasionally reported to be associated with some forms of mitochondrial myopathies, at present there is no documentation of a causal relationship [34,35].
Anesthetic Considerations
The anesthetic management of newborns and infants with congenital myopathy begins with the usual considerations for hypotonic patients in this age group. Nondepolarizing muscle relaxants should be omitted or used sparingly, and elective use of succinylcholine should be avoided entirely. Some additional specific points should also be raised. The first is that many of these children will have metabolic abnormalities that result in increased dependence on normal intravascular volume and on normal serum glucose levels. Fluids should therefore be managed closely by minimizing NPO times and providing continuous dextrose-containing IV fluids intraoperatively. Monitoring of serum glucose intra- and postoperatively is encouraged.
For a review of other perioperative considerations for the infant or child with undiagnosed muscle disease, the reader is referred to the practical approach discussed by Brandom and Veyckemans [36]. In particular, these authors (and others) emphasize the importance of evaluating these patients preoperatively to determine if their hypotonia is more consistent with a primary muscle disorder versus a mitochondrial myopathy. Elevated CK values are most consistent with a dystrophic disease and make the use of volatile agents less desirable. If, on the other hand, lactate, pyruvate, or acylcarnitines are elevated, a mitochondrial disease is more likely and a propofol-based anesthetic is less favored. At the present time, there is no evidence that a brief induction with a volatile agent is contraindicated, even in cases more likely to have a dystrophic pathology. Given the uncertainty of choice between non-triggering agents and volatile anesthetics, intraoperative monitoring for acidosis is also recommended.
Compared to myopathies in older children, there is an increased likelihood of MH-associated myopathies in neonates and infants. However, this is coupled with a paucity of reports of MH in infants, particularly under two months of age. Given the lack of case reports in this age group, neonates and infants under two months of age may have an inherently lower susceptibility to MH. As a result, we really do not know at what developmental point a neonate becomes susceptible to MH. If one is relatively certain that a neonate has a core myopathy, then it seems prudent to avoid volatile agents to the extent possible. On the other hand, most physicians who treat patients with mitochondrial disorders tend to avoid propofol, and such an approach would seem wise if that diagnosis is more likely. Narcotics, regional analgesia, dexmedetomidine, and ketamine have all been used successfully in both groups and are excellent primary anesthetics or additions when appropriate [26].
References

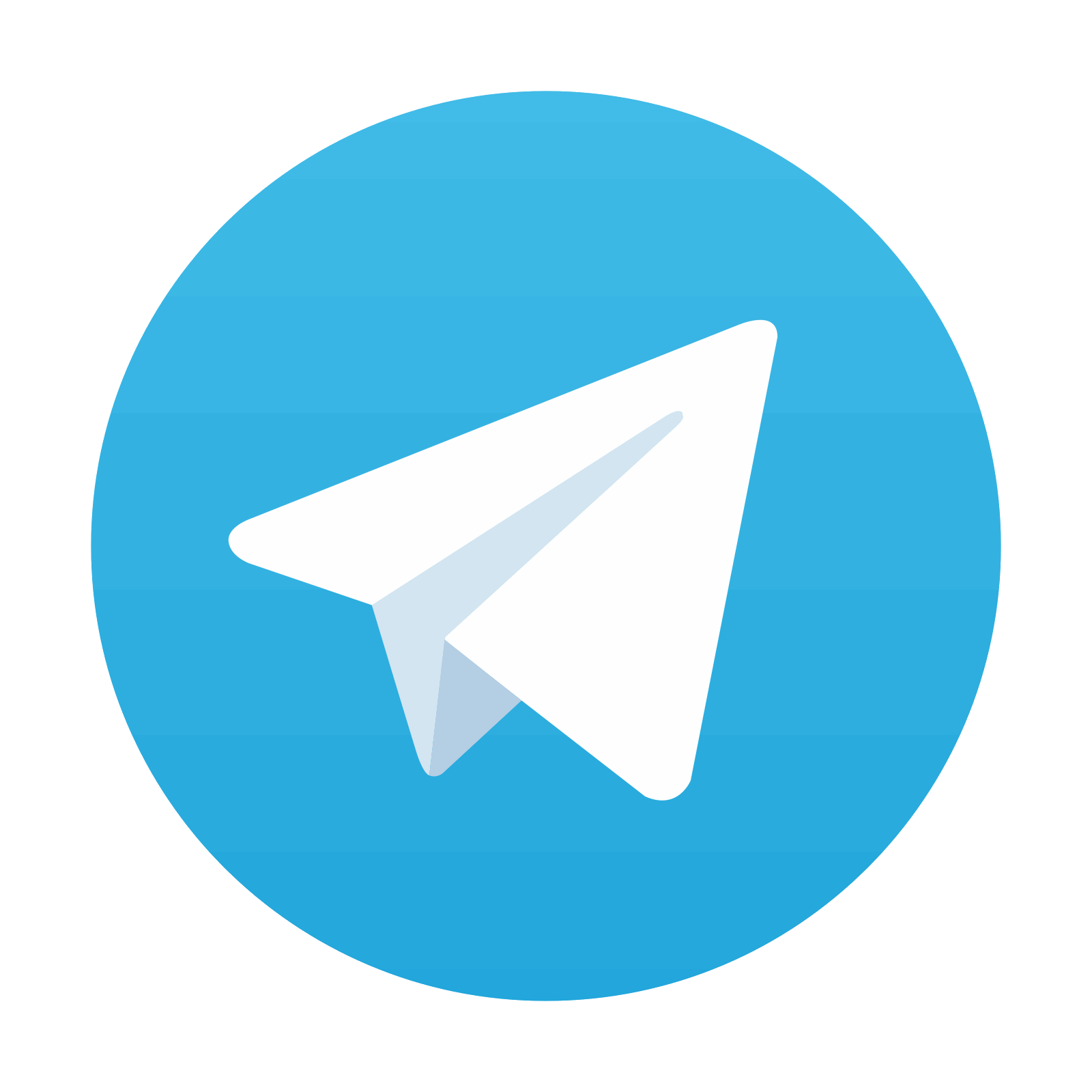
Stay updated, free articles. Join our Telegram channel

Full access? Get Clinical Tree
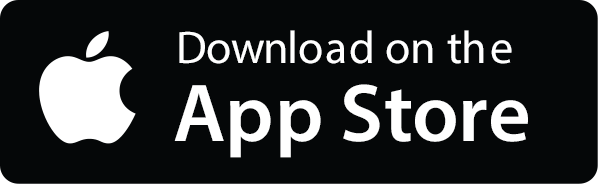
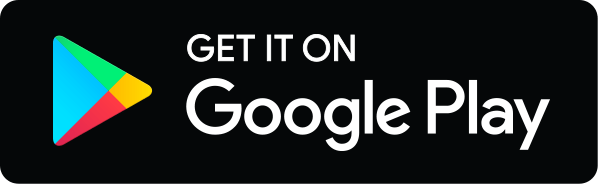
